Diffuse gliomas in adults continue to have a dismal prognosis with the current standard therapeutic methods, including maximal surgical resection, radiation, and chemotherapy. The pathogenesis of adult glioma is complex, involving the loss of function of tumor suppressor genes and activation of oncogenes, which are involved in a network of interconnected signaling pathways. Through activation of these pathways, characteristics of malignant gliomas, including uncontrolled proliferation and growth, invasion, and angiogenesis, are driven. Evolving therapeutic approaches are focused on specifically targeting these genetic lesions. This content gives an overview of the current knowledge about the pathogenesis of adult diffuse gliomas, emphasizing new targeted treatment approaches.
Glioma classification
The current World Health Organization (WHO) classification recognizes astrocytic, oligodendroglial, and mixed oligoastrocytic gliomas, largely based on morphology. Tumor classification and grade remain an important prognostic factor: survival rates for WHO grade II oligodendroglioma are approximately 80% at 5 years and 65% for fibrillary astrocytoma at 5 years. In anaplastic (WHO grade III) tumors, the median survival time is 3.5 years for oligodendroglial and 1.7 years for astrocytic tumors. Glioblastoma (WHO grade IV) is associated with a dismal prognosis, with a median survival of approximately 1 year.
Genetic and molecular characteristics
Diffusely infiltrating gliomas of different lineage and grade not only are morphologically different but also have some distinct genetic characteristics ( Fig. 1 ). With malignant progression, genetic alterations accumulate: for example, TP53 mutations and overexpression of platelet-derived growth factor (PDGF) ligands and receptors (PDGFRs) in low-grade astrocytomas are followed by accumulation of genetic alterations of cell-cycle regulatory pathways, including deletion or mutations of cyclin-dependent kinase (CDK) inhibitor, p16INK4A/CDKN2A, or the retinoblastoma susceptibility locus 1, with the progression to anaplastic astrocytoma followed by amplification or overexpression of CDK4 and loss of heterozygosity (LOH) of chromosome 10q in malignant progression of secondary glioblastomas ( Fig. 2 ).
It has been suggested that secondary glioblastomas in some instances acquire genotypic and phenotypic characteristics of primitive neuroectodermal tumors. In contrast, primary glioblastomas are characterized by frequent amplification of epidermal growth factor receptor (EGFR) and infrequent TP53 mutations.
In oligodendrogliomas, the molecular-genetic hallmark is deletion of the chromosomal arms 1p and 19q, which is present in up to 80% of cases. It is often due to an unbalanced translocation t(1;19)(q10;p10).
The 1p/19q deletion is of prognostic and predictive significance in diffuse glioma, especially high-grade tumors. A landmark study of 35 anaplastic oligodendroglioma tumor samples demonstrated that those patients whose tumors exhibited 1p/19q loss had a therapeutic response and longer survival times than patients whose tumors lacked this change. Since that time, it has been suggested that oligodendrogliomas are inherently more responsive to treatment as compared with other diffuse gliomas. Although combined 1p/19q loss was initially suggested as a predictive marker for response to alkylating chemotherapy, subsequent studies indicate that it is more generally associated with improved response to genotoxic agents in general, including radiotherapy. Nonetheless, the assessment of 1p/19q status in oligodendroglial tumors is currently a widely performed molecular-genetic test in neuro-oncologic practice. Although 1p/19q status seems to influence treatment decisions in some settings, opinions vary on the treatment implications based on the results of 1p/19q status in these tumors. Putative tumor suppressor genes have not been identified on these chromosomal arms; therefore, the link between molecular pathogenesis of oligodendrogliomas and the therapeutic implication is still missing in this case. Other mechanisms of tumor development and progression appear similar to those seen in astrocytic tumors, including deregulation of cell cycle proteins and their inhibitors, loss of phosphatase and tensin homolog deleted on chromosome 10 (PTEN), and up-regulation of angiogenic factors with the progression to anaplastic oligodendroglioma.
The molecular and genetic alterations underlying the tumorigenesis and progression of mixed oligoastrocytomas resemble those of astrocytic or oligodendroglial tumors. Tumors that have 1p/19q deletions do not have TP53 mutations and vice versa, indicating that even if morphologically ambiguous, oligoastrocytomas genetically resemble pure oligodendrogliomas or astrocytoma. Although recognized by the WHO, the use of the mixed oligoastrocytoma diagnosis remains suboptimal due to its ambiguity and difficult with respect to reproducible classification among neuropathologists. A challenge for the future is to identify molecular signatures to more precisely define the clinical behavior of these morphologically ambiguous tumors in individual cases.
Most recently, a common genetic mutation for low-grade gliomas of astrocytic and oligodendroglial lineage has been described, which can also be found in secondary glioblastomas arising from a lower-grade glioma: mutations in the isocitrate-dehydrogenase 1 and 2 genes were found in 90% of diffuse astrocytomas and 85% of secondary glioblastomas analyzed. In oligodendrogliomas, isocitrate dehydrogenase (IDH) mutations were found in 84% of grade II and 94% of grade III lesions. In contrast, only 5% of primary glioblastomas carry an IDH mutation according to this study. A summary of novel treatments aimed at the components of the malignant phenotype is given in Fig. 3 .
Genetic and molecular characteristics
Diffusely infiltrating gliomas of different lineage and grade not only are morphologically different but also have some distinct genetic characteristics ( Fig. 1 ). With malignant progression, genetic alterations accumulate: for example, TP53 mutations and overexpression of platelet-derived growth factor (PDGF) ligands and receptors (PDGFRs) in low-grade astrocytomas are followed by accumulation of genetic alterations of cell-cycle regulatory pathways, including deletion or mutations of cyclin-dependent kinase (CDK) inhibitor, p16INK4A/CDKN2A, or the retinoblastoma susceptibility locus 1, with the progression to anaplastic astrocytoma followed by amplification or overexpression of CDK4 and loss of heterozygosity (LOH) of chromosome 10q in malignant progression of secondary glioblastomas ( Fig. 2 ).
It has been suggested that secondary glioblastomas in some instances acquire genotypic and phenotypic characteristics of primitive neuroectodermal tumors. In contrast, primary glioblastomas are characterized by frequent amplification of epidermal growth factor receptor (EGFR) and infrequent TP53 mutations.
In oligodendrogliomas, the molecular-genetic hallmark is deletion of the chromosomal arms 1p and 19q, which is present in up to 80% of cases. It is often due to an unbalanced translocation t(1;19)(q10;p10).
The 1p/19q deletion is of prognostic and predictive significance in diffuse glioma, especially high-grade tumors. A landmark study of 35 anaplastic oligodendroglioma tumor samples demonstrated that those patients whose tumors exhibited 1p/19q loss had a therapeutic response and longer survival times than patients whose tumors lacked this change. Since that time, it has been suggested that oligodendrogliomas are inherently more responsive to treatment as compared with other diffuse gliomas. Although combined 1p/19q loss was initially suggested as a predictive marker for response to alkylating chemotherapy, subsequent studies indicate that it is more generally associated with improved response to genotoxic agents in general, including radiotherapy. Nonetheless, the assessment of 1p/19q status in oligodendroglial tumors is currently a widely performed molecular-genetic test in neuro-oncologic practice. Although 1p/19q status seems to influence treatment decisions in some settings, opinions vary on the treatment implications based on the results of 1p/19q status in these tumors. Putative tumor suppressor genes have not been identified on these chromosomal arms; therefore, the link between molecular pathogenesis of oligodendrogliomas and the therapeutic implication is still missing in this case. Other mechanisms of tumor development and progression appear similar to those seen in astrocytic tumors, including deregulation of cell cycle proteins and their inhibitors, loss of phosphatase and tensin homolog deleted on chromosome 10 (PTEN), and up-regulation of angiogenic factors with the progression to anaplastic oligodendroglioma.
The molecular and genetic alterations underlying the tumorigenesis and progression of mixed oligoastrocytomas resemble those of astrocytic or oligodendroglial tumors. Tumors that have 1p/19q deletions do not have TP53 mutations and vice versa, indicating that even if morphologically ambiguous, oligoastrocytomas genetically resemble pure oligodendrogliomas or astrocytoma. Although recognized by the WHO, the use of the mixed oligoastrocytoma diagnosis remains suboptimal due to its ambiguity and difficult with respect to reproducible classification among neuropathologists. A challenge for the future is to identify molecular signatures to more precisely define the clinical behavior of these morphologically ambiguous tumors in individual cases.
Most recently, a common genetic mutation for low-grade gliomas of astrocytic and oligodendroglial lineage has been described, which can also be found in secondary glioblastomas arising from a lower-grade glioma: mutations in the isocitrate-dehydrogenase 1 and 2 genes were found in 90% of diffuse astrocytomas and 85% of secondary glioblastomas analyzed. In oligodendrogliomas, isocitrate dehydrogenase (IDH) mutations were found in 84% of grade II and 94% of grade III lesions. In contrast, only 5% of primary glioblastomas carry an IDH mutation according to this study. A summary of novel treatments aimed at the components of the malignant phenotype is given in Fig. 3 .
Treatment targets
Growth Factor Signaling Pathways—Receptor Tyrosine Kinase Inhibition
Mitogenic growth signals enable tumor cells to move from a quiescent state into a proliferative state. They are transmitted into the cell by transmembrane receptors that bind diffusible growth factors, extracellular matrix (ECM) components, and cell-to-cell adhesion/interaction molecules. Glioma cells (over)express growth factors and corresponding receptors, for example PDGF, PDGFRs, transforming growth factor (TGF)-β, and EGFR, allowing for paracrine and autocrine growth stimulation. In addition, growth-stimulating signaling independentlof ligand binding can occur in tyrosine kinase receptors due to a mutation (for example, EGFR variant type III [EGFRvIII], discussed later).
PDGF/PDGFRs
Coexpression of PDGF and PDGFRs exists in gliomas of all grades and is held to be an early oncogenic event, even though expression directly increases with glioma grade. PDGF promotes not only growth but also angiogenesis through an autocrine loop. Imatinib mesylate (Gleevec, Novartis, East Hanover, NJ, USA), a kinase inhibitor of PDGFRs, c-Kit and bcr-abl, has demonstrated progression-free survival (PFS) at 6 years of 32% in patients who had immunohistochemical expression of PDGFRs. Thus, detection of the target protein may be helpful in predicting response to Gleevec.
EGFR
EGFR is the most frequently amplified gene in glioblastoma. EGFR amplification is associated with EGFR overexpression. EGFRvIII is a truncated variant of EGFR found in 20% to 50% of glioblastomas with EGFR amplification. It results in a deletion in exons 2–7 of the EGFR mRNA, thereby removing the extracellular ligand-binding domain of the receptor. Thus, EGFRvIII is constitutively activated, signaling through the phosphatidylinositol-3 kinase (PI3K), RAS, and mitogen-activated protein (MAP)-kinase (MAPK) pathways, independently of its ligand. The increased activity of EGFR promotes tumor growth through many different mechanisms, promoting survival, invasion, and angiogenesis. In accordance with these effects, EGFRvIII positivity in glioblastoma multiforme (GBM) has been linked to a more aggressive clinical phenotype with shorter overall survival times. Because EGFRvIII is a tumor-specific change on normal cells, it is a promising therapeutic target.
EGFR-targeted therapies include the monoclonal antibodies to EGFR (cetuximab [Erbitux, Bristol-Myers Squibb, New York, NY, USA]) and EGFR kinase inhibitors, gefitinib (Iressa [ZD1839], AstraZenaca, Wilmington, DE, USA) and erlotinib (Tarceva [OSI-774], Genentech, South San Francisco, CA, USA). In glioblastomas, clinical response to treatment with cetuximab has been reported in several cases. Phase I and II clinical trials are ongoing. Gefitinib and erlotinib resulted in radiographic response, but no survival benefit in clinical trials for malignant gliomas. Attempts at correlation of treatment response with molecular characteristics of gliomas produced notable result in retrospective evaluation of tumor tissues from patients enrolled in clinical trials. Coexpression of normal PTEN and mutant EGFRvIII and combined low levels of AKT and overexpression of EGFR have been identified as predictors of radiographic response.
TGF-β
TGF-β is a polypeptide growth factor that binds to its serine-threonine kinase receptor, TGF-βR. TGF-βR phosphorylates the Smad transcription factors, which regulate the transcription of TGF-β responsive genes. In normal cells, TGF-β promotes apoptosis and differentiation and blocks cell proliferation. In neoplastic cells, inactivation of TGF-β through mutation of the receptor or downstream Smad proteins results in uncontrolled proliferation. Gliomas coexpress TGF-β and its receptors in an autocrine loop. The expression levels of TGF-β increase with tumor grade. The TGF-β receptors are, however, not mutated in high-grade gliomas, suggesting a downstream signaling disruption. TGF-β also promotes invasion by inducing the expression of matrix metalloproteinase (MMP)-2. In epithelial tumors, TGF-β is associated with tumor progression and metastatic phenotype by inducing transdifferentiation, termed the epithelial-to-mesenchymal transition. Recent findings indicating an analogous transition in malignant glioma raise the possibility that TGF-β may play a role in this process. Therapeutic exploitation of the TGF-β pathway has been attempted with antisense oligonucleotide, AP12009, which has been tested in clinical trials on malignant gliomas with modest success. One protein of the TGF-β superfamily, MIC-1/GDF15, may be useful as a prognostic biomarker in glioblastoma patients: the level of MIC-1/GDF15 protein in the CSF determined by ELISA revealed a shorter survival in patients with elevated levels.
Ras-Raf-MEK-MAPK
The MAPK pathway is activated through integrins or receptor tyrosine kinase (RTK) receptors, which bind growth factors, such as TGF-β. RTK activation results in receptor dimerization and downstream activation of Ras and phosphorylation of MAPK by MEK (mitogen-activated protein kinase kinase), which in turn phosphorylates nuclear transcription factors that induce the expression of genes promoting cell cycle progression, for example, cyclin D1. Although Ras is often activated in gliomas, it does not usually carry any mutations. Rather, Ras activity in gliomas is determined by inappropriate RTK or integrin activation. The rate-limiting step of maturation of Ras is farnesylation. Farnesyltransferase inhibitors, however, including tipifarnib (Zarnestra [R115777], Johnson & Johnson, Brunswick, NJ, USA) and lonafarnib (Sarasar [SCH66336], Schering-Plough, Kenilworth, NJ, USA), have led to mixed results: although there was some efficacy in recurrent GBM, the treatment of residual disease yielded stable disease as the best response in a phase II clinical trial.
PI3K/PTEN/AKT/mTOR
The PI3K pathway is an important mediator of cell growth and proliferation. Its activation is frequent and associated with poor prognosis in glioma patients. In many cases, loss of PTEN, a major tumor suppressor that is inactivated in up to 50% of high-grade gliomas by mutations or epigenetic mechanisms, results in uncontrolled PI3K signaling and AKT activation. Downstream from Akt, the serine/threonine kinase, mammalian target of rapamycin (mTOR), regulates protein translation involving initiation factor 4E-binding protein-1 and the ribosomal S6 kinase. Approaches to blocking this signaling cascade include the mTOR inhibitors, rapamycin (sirolimus [Rapamune], Pfizer, New York, NY, USA) and its synthesized analogs, temsirolimus (Torisel, Wyeth) and everolimus (Certican [RAD001], Novartis), which have been evaluated in clinical trials. In a phase II clinical trial of temsirolimus on 65 GBM patients, a radiographic response to treatment was observed in 36% of patients.
Proliferation: reconstitution of brakes on the tumor cell cycle
Rb Pathway
A central effector of the antiproliferative signaling is the retinoblastoma protein (pRb). It blocks proliferation by sequestering the transcription factor, E2F, which is necessary for the progression from the G1 into S phase. Disruption of the pRb pathway liberates E2Fs and thus allows cell proliferation. The retinoblastoma pathway can be disrupted in different ways. Functional pRb may be lost through mutation of its gene, which occurs in 25% of high-grade gliomas. The pRb can be inactivated by cyclin D1 and CDK4 and CDK6 complexes through hyperphosphorylation. Amplification of the CDK4 and CDK6 genes also result in functional inactivation of RB in high-grade gliomas. In addition, RB activity is lost through the inactivation of a critical negative regulator of CDK4 and CDK6, p16 Ink4a .
Disruptions of the pRb pathway are frequent in high-grade gliomas and tend to involve only one component of the pathway in any given tumor.
Because cyclins and CDK inhibitors are subject to proteosomal degradation, the cell cycle regulation can be modified through proteasome inhibitors, such as bortezomib (Velcade, Millenium Pharmaceuticals, Cambridge, MA, USA). This proteasome inhibitor induces expression of p21 and p27 decreases levels of CDK2, CDK4, and E2F4, and leads to apoptosis in vitro glioma cell lines. A phase I clinical trial of bortezomib in combination with temozolomide and radiotherapy showed safety and tolerability for patients with newly diagnosed and recurrent malignant gliomas.
p53 Pathway
The p53 protein plays a role in cell cycle control, DNA damage response, cell death and differentiation. Its regulatory function in the cell cycle consists of a control function at the G1 to S phase transition point, which is effected through its downstream transcriptional target CDNK1A , which encodes the protein for the CDK2 inhibitor, p21. The proapoptotic effect of p53 is mediated by its up-regulation of proapoptotic proteins, such as BAX.
Molecular therapeutic approaches directed at loss of p53 function in gliomas attempt to restore p53 function, mainly through delivery of the p53 gene. In a phase I clinical trial of adenovirus-mediated gene transfer for recurrent gliomas, treatment was well tolerated by patients, PFS was 13 weeks, and overall survival was 43 weeks. A limitation of this treatment approach, as observed with gene therapy approaches in general, has been poor diffusion of the vector into the affected brain tissue.
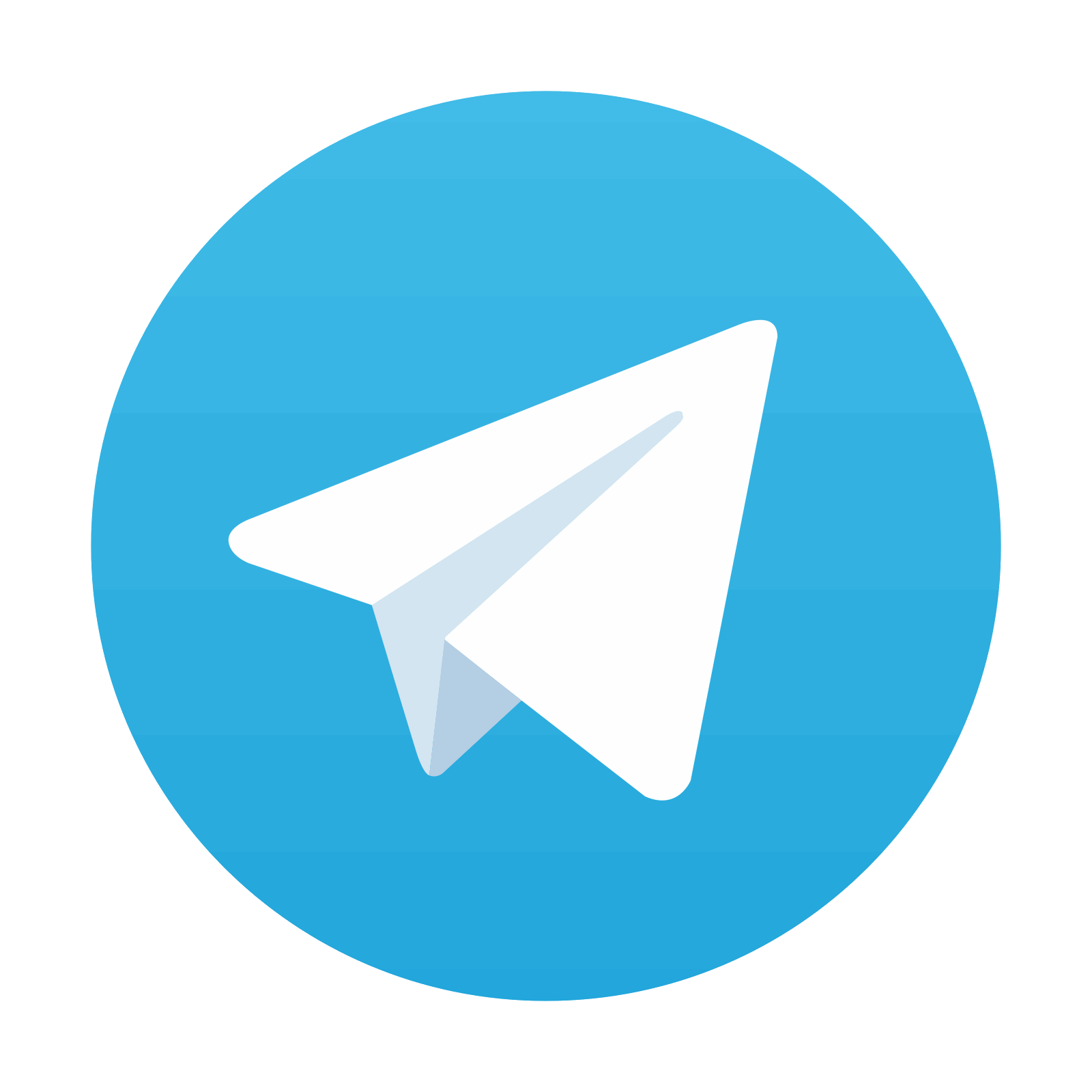
Stay updated, free articles. Join our Telegram channel

Full access? Get Clinical Tree
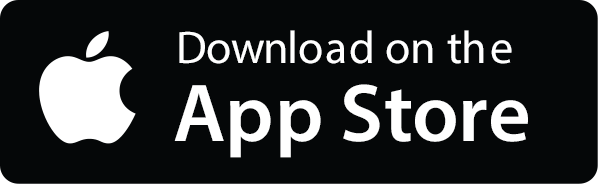
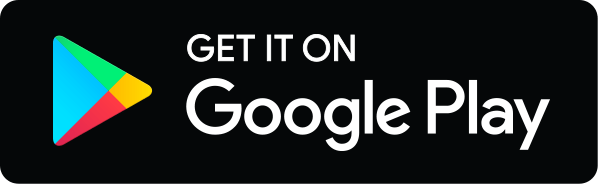