Continuous improvements in magnetic resonance scanner, coil, and pulse sequence technology have resulted in the ability to perform routine, high-quality imaging of the brachial plexus. With knowledge of the anatomy of the plexus, and a familiarity with common pathologic conditions affecting this area, radiologists can provide valuable imaging evaluation of patients with brachial plexus pathologies.
Key points
- •
Continuous improvements in magnetic resonance (MR) scanner, coil, and pulse sequence technology have resulted in the ability to perform routine, high-quality imaging of the brachial plexus.
- •
Reliable fat suppression on T2-weighted images is an absolute essential for successful brachial plexus MR imaging.
- •
MRI of the brachial plexus is a valuable diagnostic tool for detection and preoperative staging of mass lesions involving the brachial plexus, in evaluating inflammatory and traumatic brachial plexus changes.
Introduction
Direct multiplanar imaging capabilities and superior soft tissue contrast have turned magnetic resonance (MR) imaging into the primary imaging tool for evaluation of peripheral nerves, including the brachial plexus. There is increasing recognition that dedicated nerve imaging, often called MR neurography (MRN), can add valuable adjunct information to the clinical examination findings of patients with suspected nerve lesions, including brachial plexopathies. Although the term MRN often refers to images such as maximum intensity projections created from source 3-dimensional (3D) T2 images, we refer to dedicated high-resolution MR imaging of the plexus as MRN for the purposes of this article. Indications for brachial plexus MRN include intrinsic and extrinsic tumors, trauma, and inflammatory diseases. Brachial plexus MRN can initially appear somewhat daunting, considering the complexity of the neural structure. However, the nerves follow a consistent distribution after exiting the intervertebral foramina, such that anyone having an understanding the topographic anatomy and performing careful imaging can do high-quality interpretation of the plexus. In this overview, we present techniques for imaging the brachial plexus, discuss the anatomy, and demonstrate relevant examples of normal and abnormal findings.
Introduction
Direct multiplanar imaging capabilities and superior soft tissue contrast have turned magnetic resonance (MR) imaging into the primary imaging tool for evaluation of peripheral nerves, including the brachial plexus. There is increasing recognition that dedicated nerve imaging, often called MR neurography (MRN), can add valuable adjunct information to the clinical examination findings of patients with suspected nerve lesions, including brachial plexopathies. Although the term MRN often refers to images such as maximum intensity projections created from source 3-dimensional (3D) T2 images, we refer to dedicated high-resolution MR imaging of the plexus as MRN for the purposes of this article. Indications for brachial plexus MRN include intrinsic and extrinsic tumors, trauma, and inflammatory diseases. Brachial plexus MRN can initially appear somewhat daunting, considering the complexity of the neural structure. However, the nerves follow a consistent distribution after exiting the intervertebral foramina, such that anyone having an understanding the topographic anatomy and performing careful imaging can do high-quality interpretation of the plexus. In this overview, we present techniques for imaging the brachial plexus, discuss the anatomy, and demonstrate relevant examples of normal and abnormal findings.
Anatomy of the brachial plexus
The brachial plexus arises from the ventral rami of nerves C5 to C8 and T1 ( Fig. 1 ). The brachial plexus structures travel through the supraclavicular fossa, closely paralleling the course of the subclavian artery (which is the most helpful anatomic landmark on sagittal images), generally forming 3 trunks, each dividing into anterior and posterior divisions forming a total of 6 divisions, which then merge to form 3 cords. Individual ventral rami or roots are often best seen on axial images. Roots are also well seen on sagittal images. Because sagittal images are perpendicular to the plane of the plexus, in our practice, this is the favored plane for following the elements from the spine to the axilla. The coronal plane also depicts the plexus elements to good advantage, as the plexus courses within this plane paralleling the subclavian/axillary arteries.
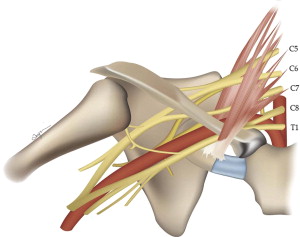
With some experience, and optimized imaging technique, evaluation of the plexus can be relatively straightforward. Although quite detailed evaluation of each level and nerve of the plexus is conceivable, most clinical applications rely on determination of the presence or absence of plexus involvement by tumor or neuritis, with a general anatomic localization. For this latter purpose, we have found that the concept of 5 key sagittal locations correlating with the 5 levels of organization is helpful: roots, trunks, divisions, cords, and branches.
The 5 key sagittal locations are as follows:
- 1.
Intervertebral foramina: Roots (C5–T1). Plexus is formed by ventral rami only ( Fig. 2 A ).
Fig. 2
Normal anatomy of the brachial plexus on sagittal images. Images of sagittal STIR (left, TR, 6000 ms; TE, 45 ms; TI, 170 ms; slice thickness and spacing, 4 and 1 mm, respectively) and T1-weighted FSE (right, TR, 800 ms; TE, 10 ms; slice thickness and spacing, 4 and 1 mm, respectively) demonstrate ( A ) the roots ( white arrows ) of the brachial plexus formed by the ventral rami of C5–T1 just exiting the intervertebral foramina. ( B ) The interscalene region, where the brachial plexus ( white arrows ) travels between the anterior ( white arrowheads ) and middle scalene muscles. This is the level just before the roots merge into the short trunks. Brachiocephalic vein (V) and subclavian artery ( asterisk ). ( C ) The supraclavicular triangle above the middle third of the clavicle where the trunks ( white arrows ) divide into anterior and posterior divisions just before the brachial plexus passes posterior to the clavicle and go from the lateral aspect of the anterior scalene muscle in front of the middle scalene muscle ( white arrowhead ) to the lateral border of the first rib. Subclavian vein (V) and subclavian artery ( asterisk ). ( D ) The divisions merging into cords (lateral, medial, and posterior) at the level where the subclavian artery becomes the axillary artery ( asterisk ) at the lateral border of the first rib. Axillary vein (V). ( E ) The change from divisions ( white arrows ) into terminal branches at the level of the pectoralis minor muscle just medial to the coracoid ( white arrowhead ), axillary vein (V), and axillary artery ( asterisk ).
- 2.
Scalene muscles, anterior and middle: Trunks (upper trunk, C5–C6; middle trunk, C7; lower trunk, C8/T1). The plexus travels between the anterior and middle scalene muscles and the very short trunks form at approximately the lateral border of the scalene triangle (see Fig. 2 B).
- 3.
Supraclavicular triangle: Divisions (anterior and posterior). The supraclavicular triangle is above the middle third of the clavicle. The trunks divide into anterior and posterior divisions just before the brachial plexus passes posterior to the clavicle and go from the lateral aspect of the anterior scalene muscle to the lateral border of the first rib (see Fig. 2 C).
- 4.
Axilla: Cords (lateral, medial, and posterior). The subclavian artery becomes the axillary artery at the lateral border of the first rib. Cords run from the mid-clavicle to the inferomedial coracoid process, and are named according to location relative to the axillary artery (see Fig. 2 D). Typical arrangement anterior to posterior on a lateral view is lateral, posterior, and medial cords.
- 5.
Coracoid/pectoralis minor muscle: Branches. Terminal branches include the radial, axillary, musculocutaneous, median, and ulnar nerves (see Fig. 2 E).
Normal nerve tracts of the plexus are embedded in fat, and are well delineated from neighboring vessels and musculature on T1-weighted sequences (see Fig. 2 ). Individual nerves can be attributed to specific nerve roots with some interindividual variability (eg, the suprascapular and subclavian nerves represent the first branches of the brachial plexus and arise from the upper trunk formed by C5, sometimes also C5 and C6 nerve roots). The suprascapular nerve can often be easily recognized, because it has a relatively large diameter. Of note, the long thoracic nerve arises directly from the nerve roots, usually from C5–C7, less commonly also from C4. The signal intensity of the plexus elements is similar to that of muscle on T1-weighted images, and hyperintense to muscle on fat-suppressed T2-weighted sequences. The axillary and subclavian vessels (especially venous structures) can also show considerable high signal within them on fast spin-echo or inversion-recovery–type pulse sequences, and this signal needs to be distinguished from that of the brachial plexus. Saturation bands may help decrease vascular signal, but is not always effective for veins. Mapping out which structures are vascular or not is effectively accomplished with sagittal gradient-echo sequences, which are a part of our routine MRN protocol.
For clinical correlation, an upper brachial plexus injury/lesion involving spinal nerves C5 and C6 results in paralysis of the shoulder muscles and biceps muscle. If the lesion/injury also includes spinal nerve C7, some of the wrist muscles are also impaired. A lower brachial plexus injury/lesion involves spinal nerves C8 and T1, resulting in paralysis of the forearm flexor and the intrinsic muscles of the hand. Injuries to the stellate ganglion or cervical sympathetic trunk may cause Horner syndrome.
Imaging technique
It is slightly controversial whether 1.5-T or 3.0-T field strengths offer the best performance in brachial plexus imaging, but in MRN, there is definitely a trend to use the highest possible field strength for optimal signal-to-noise ratio (SNR) of peripheral nerves at the required fields of view. Some investigators recommend using 3.0-T scanners, although those with 1.5-T scanners can achieve very high-quality imaging with careful technique. The selection of appropriate imaging coils for the brachial plexus is challenging because of the complex anatomy of the supraclavicular region, neck, and shoulders; we are not aware of dedicated coils produced specifically for brachial plexus imaging. There are also many interfaces with soft tissue and air, creating magnetic field inhomogeneities that can interfere with adequate fat suppression. Although the body coil is capable of imaging a large field of view, the signal to noise of this coil is rarely sufficient to achieve good-quality, high-resolution images covering the brachial plexus from the spine to the axilla. Better options include a torso-type phased-array coil, head-neck-spine phased-array coil, or a specialized neurovascular array coil designed for imaging of the aortic arch and neck vessels. The neurovascular array is our coil of choice for brachial plexus imaging in almost all cases. Another reasonable coil selection is a flexi-coil or surface coil; the drawback of these coils is that they have limited anatomic coverage without repositioning the coil.
The classic structural MRN relies predominantly on T2-weighted fat-suppressed (FS) sequences and T1-weighted spin-echo–type sequences. Images are ideally obtained with a high-resolution matrix on a relatively small field of view ( Table 1 ). In addition, selective maximum intensity projections of multiple successive sections may be used to lay out the course of a nerve. In our experience, these “neurogram” images are more useful as a communication tool and adjunct image set; primary diagnosis relies on evaluation of source images on all sequences. Reliable fat suppression on T2-weighted images is an absolute essential for successful brachial plexus MRN. This requisite can actually be very challenging, because conventional spectral (“chemical”) saturation techniques that use the differences in resonant frequencies between water and fat fail owing to the multiple air interfaces inside and outside of the chest in the plexus region. Techniques that use differences in T1 relaxation, such as short tau inversion recovery (STIR) and its faster, multi-echo variants, can offer homogeneous fat suppression, but often suffer from relatively lower SNR, longer imaging times, a higher specific absorption rate, and more pulsation artifacts, depending on the technique used. In the authors’ experience, however, fast STIR sequences have worked very well when measures are taken to maximize image quality, such as increasing signal averaging and decreasing the echo time to intermediate values. Consequently, we use predominantly fast inversion recovery imaging with repetition time (TR) of approximately 6000, echo time (TE) approximately 45, and inversion time (TI) approximately 170 ms. This produces robust fat suppression in virtually all cases. Alternatively, we are also using a 3-point Dixon pulse sequence to obtain images with water only, fat only, and combined signal as detailed later in this article. With adequate local or phased-array coils, the image quality can be routinely excellent.
Sequence | Field of View, cm | TR | TE/TI | Slice Thickness, mm/Spacing | Matrix |
---|---|---|---|---|---|
Cor T1 FSE | 22 | 800 | 14 | 3/0.5 | 512/192 |
Cor STIR | 22 | 6000 | 45/170 a | 3/0.5 | 320/192 |
Sag T1 FSE | 18 | 800 | 10 | 4/1 | 384/192 |
Sag STIR | 18 | 6000 | 45/170 | 4/1 | 320/192 |
Sag GRE | 18 | 25 | 10 b | 4/1 | 384/192 |
Ax T1 FSE | 22 | 800 | 14 | 3/1 | 384/192 |
Ax STIR | 22 | 6000 | 45/170 | 3/1 | 288/192 |
If needed: | |||||
Cor T1 IDEAL post Gd | 22 | 900 | min full | 3/0.5 | 320/192 |
Sag T1 IDEAL post Gd | 18 | 900 | min full | 4/1 | 320/192 |
a Optimal inversion times will vary by scanner field strength and manufacturer. For 1.5 T, we use TI of 140–150 ms. For 3 T, TI is typically set at 170 ms as indicated.
Newer techniques also offer improved fat suppression compared with conventional fat suppression methods: chemical shift imaging, such as the 3-point Dixon-based iterative decomposition of water and fat with echo asymmetry and least-squares estimation (IDEAL) method that applies decomposition of fat and water proton signals according to their chemical shift to isolate these 2 components into 2 separate images, as well as hybrid techniques, such as spectral presaturation inversion recovery (SPIR) and spectral presaturation attenuated inversion recovery (SPAIR) that combine both, chemical shift techniques and relaxation rate-dependent techniques. The SPAIR technique offers the additional advantages of improved insensitivity to susceptibility limitations or B1 uniformity deficiencies, better SNR than STIR with relative lack of pulsation artifacts, and better SAR attribute.
In traumatic brachial plexus injuries, the application of gadolinium in the acute phase is usually not necessary. The injection of gadolinium contrast agents is usually reserved for neoplastic, inflammatory (infectious, postradiation, autoimmune), and postoperative cases. In chronic plexopathies, gadolinium may demonstrate scar tissue. In plexopathies of unknown origin, it is recommended to check the findings on nonenhanced images to see whether additional contrast-enhanced sequences are required. Although not necessarily adding to the overall diagnostic value for mass detection, selected patients may also undergo 3D contrast-enhanced MR angiography for evaluation of the subclavian, axillary, and brachiocephalic vessels. This may be performed with an ultrafast 3D spoiled gradient echo sequence during dynamic gadolinium injection.
Imaging sequences vary considerably among institutions and for different field strength scanners. The authors’ current protocol includes a sagittal localizing image set through the midline to obtain the orientation of the cervical spine. When tolerated, we ideally place the patient’s head on a small pad to reduce the normal cervical lordosis. With this, slightly oblique coronal T1-weighted and FS T2-weighted images can be obtained in a plane that parallels the cervical exit foramina. For those less familiar with the appearance of the plexus on coronal images, it is reasonable to obtain coronal images with a wider field of view of 32 to 40 cm and to evaluate for symmetry. However, for higher-resolution imaging, we decrease the field of view to approximately 24 cm and either center on the cervical spine, or move the coil off center to focus on the plexus unilaterally. This decision is based on radiologist preference. Following coronal images, sagittal T1-weighted, FS T2-weighted, and gradient echo bright blood vascular images are performed of the symptomatic side. Images begin on the contralateral aspect of the cervical spine and continue to the coracoid process on the affected side. The image field of view for these sequences is 20 to 24 cm. Although coronal and sagittal images are adequate to fully evaluate the plexus, the addition of axial images should be considered for those more comfortable with this scan plane or in any case in which detailed evaluation of spinal extent of a focal lesion is needed. Note that we do not consider a brachial plexus examination to include a comprehensive cervical spine study; rather it screens the cervical spine region and focuses on the plexus itself. The exact parameters of the authors’ current brachial plexus MR protocol are listed in Table 1 .
Newer techniques allow for “microstructural” MRN, such as diffusion-weighted imaging (DWI), which can provide information about the integrity of the white matter. But DWI can provide only limited information about the actual direction of the diffusion of water molecules within the nerve, whereas diffusion tensor imaging (DTI) can provide quantitative information about the degree and direction of water diffusion within the nerves of the brachial plexus and, when used for tractography, it can visualize the white matter tract to characterize its integrity. DTI of the brachial plexus is challenging, with some of the main limitations being small size of the nerve fibers, and the localization and course of the brachial plexus between the neck and the shoulder resulting in geometric distortion and artifacts. Recently, however, tractography has been shown to demonstrate the patterns of nerve fibers passing around schwannoma masses, of nerve fibers passing centrally through neurofibromas, and infiltration/disruption of nerve fibers by metastases in the brachial plexus region, which may further help in lesion characterization. Furthermore, another recent study showed good reproducibility of DTI quantitative analysis with only small variations in calculated fractional anisotropy and apparent diffusion coefficient values in the brachial plexus components of normal volunteers. DTI and tractography are not yet in wide routine clinical use, but offer exciting research opportunities. With ongoing technical improvements, the trend toward ever-higher field strengths, and establishing of valid and reproducible standard normal values, they will likely very soon become important tools in the routine evaluation of brachial plexus pathologies.
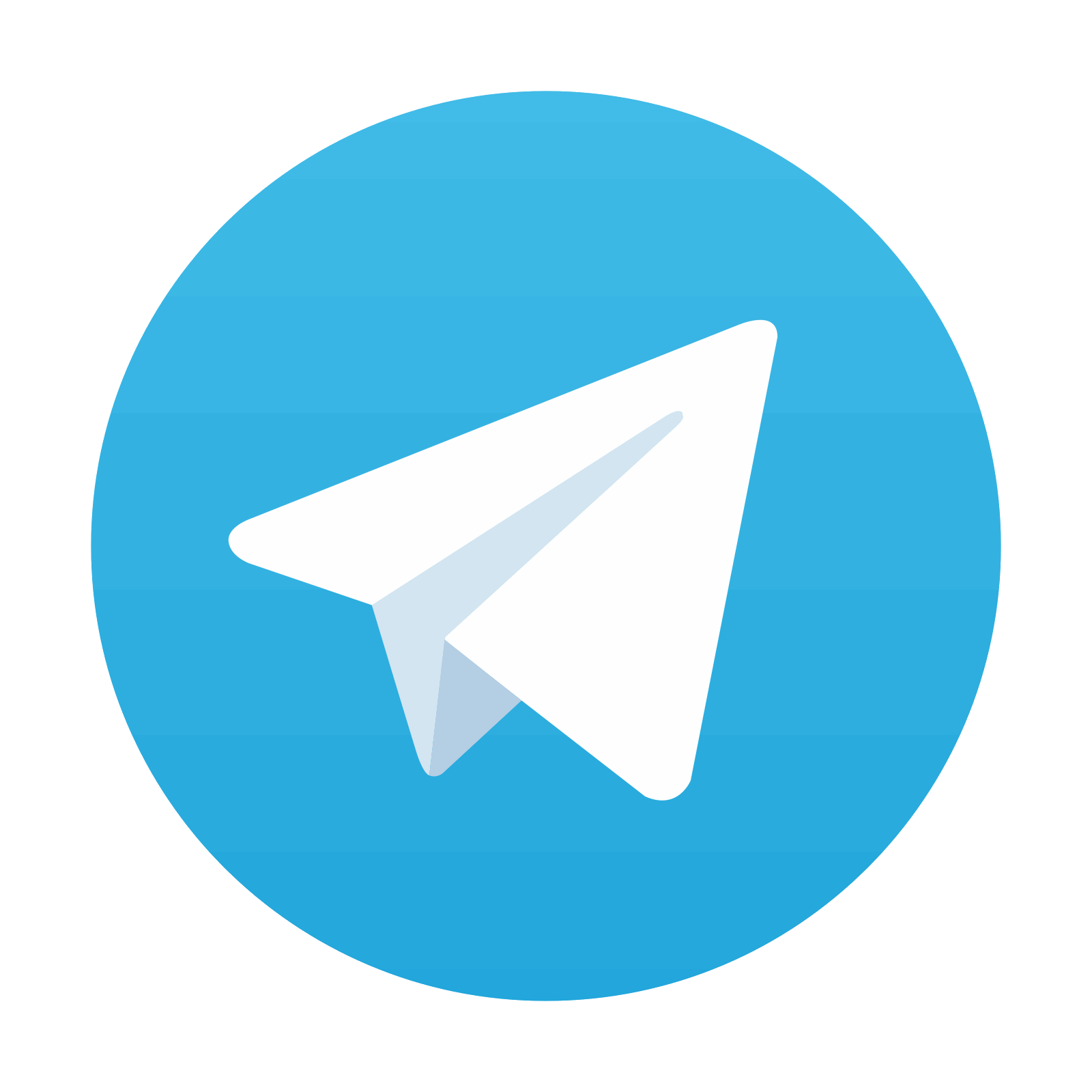
Stay updated, free articles. Join our Telegram channel

Full access? Get Clinical Tree
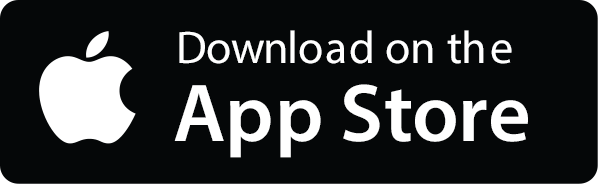
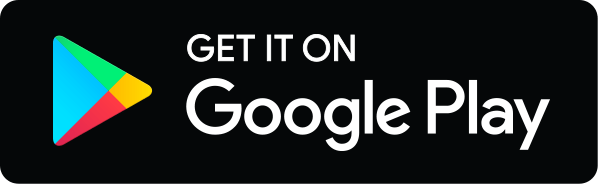