NP name
System
Indication
Status (trial status)
Company or center
References
BIND-014
Polymeric NP loaded with docetaxel targeted to prostate-specific membrane antigen
Solid tumor cancers
Phase I
Bind biosciences
[59]
CALAA-01
Cyclodextrin-containing polymer with siRNA
Solid tumor cancers
Phase I
Calando pharmaceuticals
[60]
CRLX101
Camptothecin (CPT) conjugated cyclodextrin-based polymer
Advanced solid tumor cancers
Phase I
(Phase II for Non-small Cell Lung Cancer)
Cerulean Pharma Inc.
[61]
C32/DT-A
Toxin-based suicide genes
Cationic polymer NP -poly(b-amino ester) polymer
Benign prostatic hyperplasia (BPH) and localized PCa
Preclinical
None
[62]
Doxil and Taxotere
Doxil –
PEGylated liposome encapsulating doxorubicin
Advanced Androgen-Independent PCa
Doxil – Phase II
Taxotere – Phase I
James Graham Brown Cancer Center
[63]
MFL AS
Magnetic NPs in combination with LDR brachytherapy
Localized PCa
Phase II
MagForce Nanotechnologies
[64]
Paclitaxel and Lapatinib
Paclitaxel albumin-stabilized NP formulation
Advanced solid tumor cancers
Phase I
National Cancer Institute, University of California
[65]
Perhaps one of the most attractive advantages of nanomedicines is the fact that they can be developed and manufactured in a versatile manner, allowing for their physical properties to be easily altered and tuned to a specific application. NP properties such as composition, size, surface charge, load, specificity, toxicity, and degree of selectivity can be modified according to the nature of the organ, tissue, or cell population identified for therapy. Therefore, NPs can be envisaged as a tool-kit technology with tuneable properties toward various applications involving therapeutic drug delivery and/or diagnostic imaging. Nanomedicines can be utilized in both a targeted and a non-targeted manner, and more recently interest in the development of targeted NPs has increased and efforts have been made to enhance NP retention at sites of disease through the conjugation of targeting ligands to their surfaces. This has become an effective strategy for increasing the concentration of therapeutic NPs at tumor sites, owing to the high surface-area-to-volume ratios of NP surfaces which accommodate high ligand densities, in addition to research into the identification and isolation of targeting ligands with high affinities to extracellular domains [7].
In summary, specific to drug delivery applications, NPs provide the following advantages: (1) the encapsulation and delivery of poorly soluble drugs, (2) the reduction of systemic toxicity due to free drug, (3) spatial and temporal controlled release of drugs, (4) highly localized release of drug due to the incorporation of targeting elements onto the NP, (5) the co-delivery of two or more types of drugs to sites of action for combination therapy, (6) the visualization and quantitation of drug delivery and/or therapeutic response, (7) the delivery of plasma-sensitive nucleic acids such as siRNA or miRNA in an intracellular manner, and (8) the extension of drug life-cycles post-patent expiration [2, 6].
Polymeric Nanomedicines
Polymeric NPs represent a highly effective nanoplatform for drug delivery [8]. An attractive feature of polymer-based drug delivery platforms is their controlled-release properties which is attainable via the use of biodegradable monomers [9]. Polymer NPs are capable of drug encapsulation and release in a temporally controlled manner, a property which can be achieved through surface or bulk erosion of the polymeric NPs, diffusion of drugs out of the polymer mesh network, or swelling of the polymer matrix and subsequent diffusion [10]. Drug release in polymeric systems may also be achieved in a triggered or smart manner in addition to temporal release, which can be manifested by response of the polymers to various triggers in their environment such as pH, heat, enzymes, or exposure to other appropriate sources of energy or chemicals that can break bonds or displace the drug molecules [10]. Their property of longitudinal controlled-drug release renders polymeric nanomedicines highly appealing as drug delivery vehicles, since drug release can be optimally achieved at the site of action for over long periods, without the patient requiring repeat dosing. Examples of drug delivery and imaging applications of polymeric NPs will be discussed in the subsequent sections of this chapter.
Nontargeted Nanomedicines for PCa Therapy
Oncology is one of the areas where nanomedicine has had the most impact to date, in particular with many NPs developed for the treatment and imaging of PCa [11, 12]. Both actively and passively targeted nanomedicines have been extensively utilized for oncology applications, in particular for drug delivery to solid tumors. If drug delivery NPs are to successfully reach the tumor and effectively deliver their drug load to cancer cells, they must be capable of effectively maneuvering the complex in vivo environment, and in particular avoiding clearance by the host phagocytic cells post-systemic administration. Indeed the dose of NPs that reach the tumor is dependent on a number of biophysicochemical properties of the NPs. These include the chemical signatures of the constituent material, be it lipids, polymers, or other inorganic components; the nature of the encapsulated drugs; the size and shape of the NP; and surface charge and hydrophilicity. In addition to these NP parameters, the tumor microenvironment, such as the degree of vascularization, necrosis, and size can also be factors influencing the navigation of NPs within tumors and hence their therapeutic efficacy. In the case of nontargeted NP-mediated drug delivery, the non-heterogeneous nature of the tumor microenvironment and the presence of abnormal leaky vasculature, termed the enhanced permeability and retention (EPR) effect, are beneficial as NPs can effectively extravasate through these distorted blood vessels and accumulate in tumor tissues at high concentrations. The degree of extravasation is inversely proportional to NP size, and smaller particles with sizes < 150 nm are deemed to be most effective at trans-endothelial passage into tumor tissue [13].
Considering that docetaxel (Dtxl) is the common first-line chemotherapeutic treatment in castration-resistant PCa and given the adverse effects of Taxotere, which is the clinically approved formulation of Dtxl, Cervin et al. formulated liquid crystal nanoparticles (LCNPs) and showed these NPs to be effective at inhibiting PC3-induced tumors in SCID mice [14]. These 80–90 nm NPs were formulated with the lipids phosphatidyl choline, glycerol dioleate, and polysorbate 80, and their efficacy on tumor growth post-systemic administration was compared to that of Taxotere and empty LCNPs. In one study, the results from these nontargeted and passively accumulating NPs showed that the LCNP/Dtxl formulation led to the highest level of tumor regression of volumes decreasing to 10% or less in comparison to 18% and 70% for Taxotere, 10 days post-administration (total Dtxl dose of 1.62 mg used for entire course of study) [14].
The EPR effect can be successfully utilized for drug delivery to solid tumors, and other disease indications that lead to impaired lymphatic drainage and aberrant vasculature. However, active targeting using affinity ligands may lead to more efficient retention of nanomedicines within tumor sites [15].
Targeted Nanomedicines for PCa Diagnosis and Therapy
Targeted NPs have the advantage of faster retention within tumors due to selective binding to overexpressed receptors on the surface of cancer cells. It is envisaged that the development of targeted NPs which represent the next generation of nanomedicines entering the clinic will lead to more effective therapeutic and imaging agents for PCa [16–18]. These vehicles can be engineered to recognize biophysical characteristics that are unique to the cancer cells. Most commonly, this recognition process involves the binding of vehicles to antigens that are expressed on the plasma membrane of the targeted cells. Small molecule ligands, antibodies, antibody fragments, and peptides have been used as targeting moieties in targeted nanoparticle development. A particular class of targeting ligands with high affinity to PCa cells is aptamers (Apts). Aptamers are single-stranded DNA or RNA oligonucleotides that fold into well-defined 3D structures that are capable of high affinity toward proteins, phospholipids, sugars, and nucleic acids [19]. Aptamers are ideal targeting ligands as they are non-immunogenic and exhibit remarkable stability in a wide range of pH (4–9), temperature, and organic solvents, with minimal loss of activity [20]. Furthermore, Apts can be chemically synthesized without the need for biological production, leading to reduction in batch-to-batch variability, which is one major advantage of Apts in comparison to larger and bulkier antibodies that can elicit toxic immune responses and are not easily produced on large scales [13].
We have shown that the generation of NP–Apt bioconjugates with nuclease-stabilized A10 2-fluoropyrimidine RNA Apts that bind to the prostate-specific membrane antigen (PSMA) efficiently targets and enters prostate epithelial cells which express the PSMA protein [21]. PSMA is a well-known characterized transmembrane protein that is overexpressed on PCa epithelial cells, is involved in membrane recycling, and becomes internalized through ligand-induced endocytosis [22]. Using the FDA approved biodegradable drug delivery polymer poly(d,l-lactic-co-glycolic acid) (PLGA) as a controlled release polymer, we were able to develop NPs with surfaces decorated with Apts that were targeted to the PSMA on the surface of PCa cells [20]. The developed Apt-targeted polymer NPs demonstrated a 77-fold increase in binding to prostate LNCaP cells in comparison to nontargeted polymeric NPs. To protect these particles from macrophages and increase their circulation time in vivo, the particles were also functionalized with the widely used poly(ethylene glycol) (PEG) polymer. These proof-of-principle studies using Apts as targeting ligands led us to develop and pioneer Apt-conjugated polymeric NPs to improve the therapeutic index of drugs by targeted delivery and controlled release to PCa cells [21, 23]. To achieve differential cytotoxicity against PCa cells, Apt-conjugated polymeric NPs were loaded with Dtxl, which has been demonstrated to prolong survival of patients with hormone-resistant PCa [20]. Figure 15.1 presents the general procedure used to prepare Dtxl-encapsulated pEGylated PLGA NP–Apt bio-conjugates, which were formulated using a nanoprecipitation technique. The targeted polymeric NPs were formulated by first co-preci-pitating Dtxl with the diblock polymer poly(lactide-co-glycolide)-poly(ethylene glycol) (PLGA-PEG), followed by surface functionalization with the A10 Apt, with affinity to the extracellular domain of PSMA [23]. Using this method, the hydrophilic PEG polymer protrudes outward on the surface of the polymeric NP core, and the terminal carboxy functionalities can then be conjugated with the A10 PSMA Apt targeting ligand. Additionally, the negative charge of the carboxylate groups prevents the nonspecific interaction of the Apt molecules with the surface of the NPs [20].
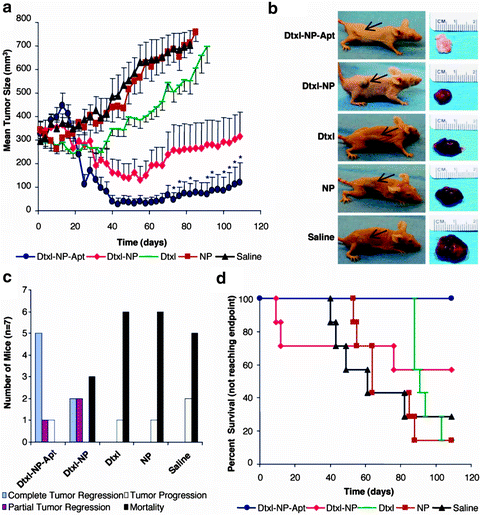
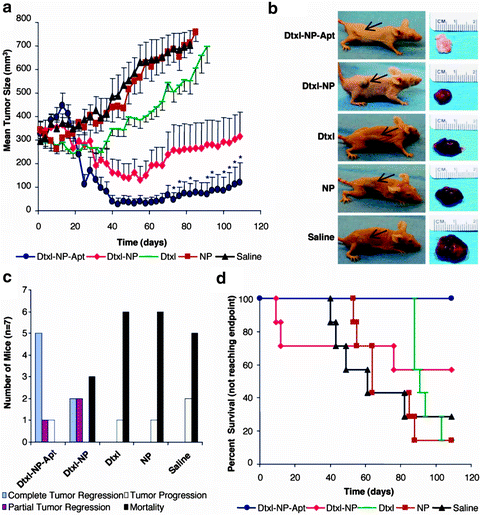
Fig. 15.1
Development of Dtxl-encapsulated PEGylated PLGA NP-Apt bioconjugates. (a) Synthetic scheme and (b) representative SEM image of the targeted NPs. From Farokhzad et al. [20], with permission. Copyright 2006 National Academy of Sciences USA
The efficacy of the Apt NPs was investigated using a mouse xenograft model of PCa and the NPs were injected intratumorally in LNCaP generated solid tumors (Fig. 15.2). PCa was induced in mice by implanting LNCaP prostate epithelial cells s.c. in the flanks of nude mice and allowing the tumors to develop to appropriate sizes (∼300 mm3). The tumor size and weight were monitored for up to 109 days and results revealed that a single intratumoral administration of Dtxl–NP–Apt Bioconjugate NPs was significantly effective at tumor size reduction compared to the nontargeted NP controls. The Apt conjugated NPs are believed to bind to PSMA on the surface of the LNCaP cells and become endocytosed, allowing their cytotoxic Dtxl cargo to be delivered post-endosomal release. This study demonstrated how the therapeutic index of Dtxl can be improved with the Dtxl-encapsulated PLGA-PEG-Apt NPs showing almost complete tumor reduction and 100% survival, compared with the survivability of 57% for nontargeted PLGA-PEG NPs and 14% for Dtxl alone (Fig. 15.2) [23]. The materials used in the development of these bioconjugated NPs are FDA approved and the Apts are small in size, relatively stable, nonimmunogenic, and easy to synthesize, which can facilitate the translation of these nanomedicines into clinical practice [23]. However, it is important to note that, akin to other nucleic acid-based ligands, Apts may also suffer from nonspecific interactions after systemic administration and thus it is important to engineer optimal NP surface properties and ligand densities that lead to effective targeting of nanomedicines at sites of disease.
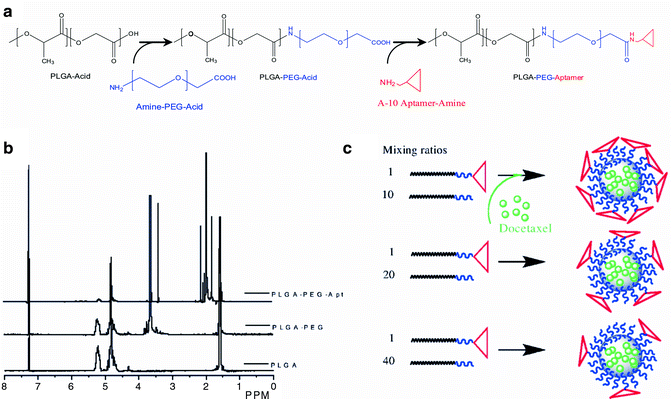
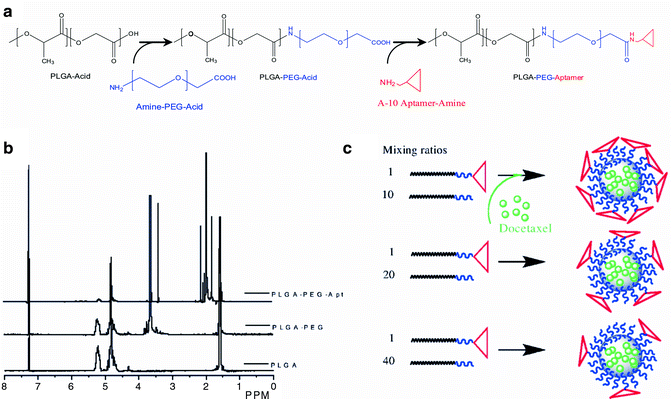
Fig. 15.2
In vivo efficacy study of Apt–NP bioconjugates and controls. (a) Comparative efficacy study of a single intratumoral injection of Apt–NP bioconjugates and controls (40 mg/kg total Dtxl dose). (b) Representative mice at each end point (Left) alongside images of excised tumors (Right). Black arrows; position of the implanted tumor on each mouse. (c) Plot of outcomes for each of the treatment groups, complete tumor regression (blue), incomplete tumor regression (red), tumor growth (yellow), and mortality (black). (d) Kaplan–Meier survival curve (end points defined as tumor load of 800 mm3 or BWL ∼20%). From Farokzad et al. [20], with permission. Copyright 2006 National Academy of Sciences USA
In addition to Dtxl delivery, Apt–NP bioconjugates have also been developed for the delivery of cisplatin to PCa cells [24]. Cisplatin is the most potent member of the Pt anticancer drug family, and its use in PCa therapy is therefore highly attractive. We devised a strategy for cisplatin therapy in PCa that employed Pt chemistry and NP delivery vehicles [24]. In this study, a hydrophobic platinum (IV) prodrug was synthesized for encapsulation into polymeric PLGA-b-PEG NPs using the nanoprecipitation method, which resulted in highly loaded NPs of appropriate size. The NPs were then targeted to PSMA by decorating the surface of the particles with A10 Apts that specifically bound to the extracellular domain of PSMA. The Apt-facilitated cellular uptake of the Pt(IV)-encapsulated NPs by PSMA expressing LNCaP cells via endocytosis was demonstrated using an antibody specific for endosome formation. The Apt-derivatized Pt(IV)-encapsulated NPs were shown to be significantly superior to cisplatin or nontargeted NPs in inhibiting LNCaP cellular growth. Moreover, by encapsulating a cisplatin prodrug, PLGA-PEG-Apt NPs displayed significant dose-sparing, with equivalent antitumor efficacy in LNCaP xenografts achieved at only a third of the conventional administered dose of free cisplatin (0.3 mg/kg vs. 1 mg/kg) [25].
Nanoparticles for Sensitive Diagnosis of PCa
Novel targeted NPs for the detection of extremely small amounts of PSA were recently developed by Mirkin and coworkers that are capable of detecting PSA at femtogram levels [26, 27]. This system utilizes magnetic microparticles conjugated with antibodies that extract trace levels of PSA from patient’s blood, and then PSA levels are detected using gold NPs that posses PSA-specific antibodies and short DNA sequences that act as “barcodes.” This development can lead to more efficient detection and monitoring of PSA levels posttreatment and therefore PCa outcomes.
Modular Self-assembly of Targeted Nanomedicines for PCa
Conventional methods of synthesizing targeted NPs involve serial chemical processing of particles, whereby drug-encapsulated NPs are first formed, followed by the conjugation of targeting ligands to their surface. This post-conjugation requires the addition of an excess amount of reactants to ensure high coupling efficiencies, after which the ligand-conjugated NPs need to be further purified by removing the excess reactants. This added complexity makes it difficult to adjust the NP surface properties in a reproducible manner and the multistep processing contributes to premature drug release from particles, resulting in batch-to-batch variability of NP surface properties and drug loading and release characteristics. To precisely engineer targeted NPs in a simple and scalable manner, an innovative strategy was developed by first pre-functionalizing polymer components with targeting ligands, and then self-assembling the polymers into NPs [28]. Figure 15.3 presents the development and characterization of PLGA-PEG-Apt triblock polymers, and the self-assembly of targeted NPs simply by nanoprecipitating the mixture of PLGA-PEG, PLGA-PEG-Apt, and drug. In this manner, by using distinct ratios of PLGA-PEG-Apt and PLGA-PEG during NP formulation, the Apt surface density can be precisely tuned. Thus, this approach could eliminate the need for post-particle modification and purification, enabling the formulation of distinct targeted NP libraries.
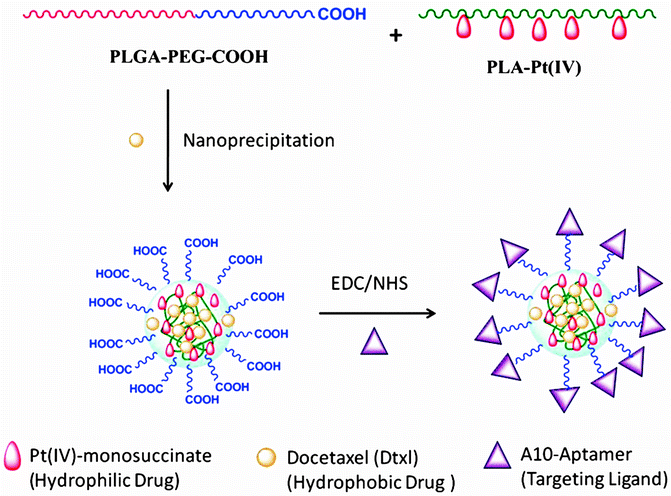
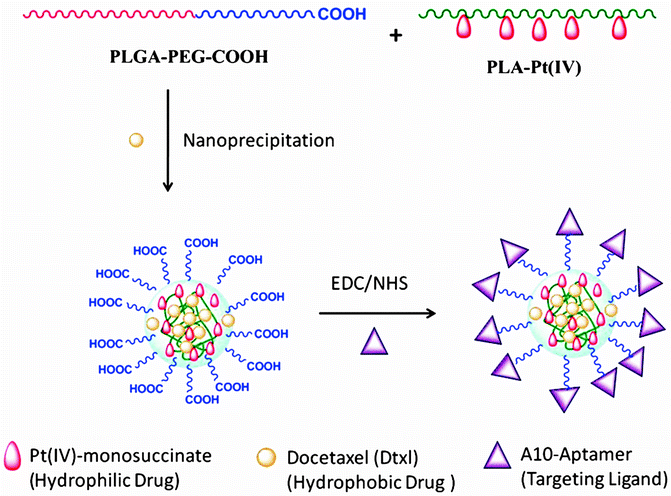
Fig. 15.3
Development of self-assembled targeted NPs. (a, b) Synthesis and characterization of PLGA-PEG-Apt triblock polymer. (c) In situ self-assembly of PLGA-PEG-Apt NPs by the nanoprecipitation method. From Gu et al. [28], with permission. Copyright 2008 National Academy of Sciences USA
Other types of polymer conjugates have also been used effectively for drug delivery to PCa. The delivery of Dtxl using actively targeted N-(2-hydroxypropyl)methacrylamide (HPMA) copolymer conjugates consisting of covalently bound Dtxl and the RGDfk targeting ligand was recently demonstrated by Ray et al. [15]. Their small sized RGDfk bioconjugate polymeric nanoparticles were shown to inhibit the proliferation of human prostate cancer DU145 and PC3 cells in vitro and effectively lead to the regression of DU145 tumor xenografts in nu/nu mice in vivo, following a single dose of either 20 mg/kg or 40 mg/kg of Dtxl [15].
With advances in nanoengineering technologies for the development of targeted NPs and their high-throughput screening, the potential to rapidly develop targeted NPs and accelerate their clinical translation is now therefore feasible.
Novel Nanomedicine Approaches for PCa Treatment and Monitoring
Newly developed nanomedicines have shown promising results in the treatment of PCa, by achieving an improvement in drug biodistribution and therapeutic index. In particular, targeted NP delivery has led to the improvement of site-specific drug delivery. In addition to exploring further specific prostate cell-surface antigens and the delivery of improved PCa drugs, other avenues such as combination therapies, precisely engineered nanomedicines, and the use of smart theranostic NPs also present exciting and promising avenues for PCa treatment and monitoring in the future.
Combination Therapies
Combination therapy by co-delivering multiple drugs via targeted polymeric NPs was proposed to address the challenges of single-agent chemotherapy [29], with several advantages which include the following: (1) co-delivery of precise drug ratios to targets of interest for synergistic therapeutic effects, (2) control over drug resistance, and (3) control of co-drug exposure in a temporal manner. In a proof-of-concept study, cisplatin and Dtxl were effectively co-delivered to PCa cells using a targeted PLGA-PEG NP platform with synergistic cytotoxicity [29]. The hydrophilic Pt(IV) cisplatin prodrug was first conjugated to a polylactide polymer derivative with pendant hydroxyl groups (PLA-OH) to yield a PLA-Pt(IV) copolymer, and subsequently blended with PLGA-PEG and Dtxl by a nanoprecipitation process (Fig. 15.4) [29]. The dual-drug encapsulated NPs were then conjugated with the A10 Apt to develop a targeted co-delivery NP platform [29]. In vitro studies demonstrated that the Apt-targeted, dual-drug encapsulated NPs were ∼ 5.5-10 times more cytotoxic than respective single drug encapsulating NPs (PLA-Pt-NP-Apt and Dtxl-NP-Apt) [29].
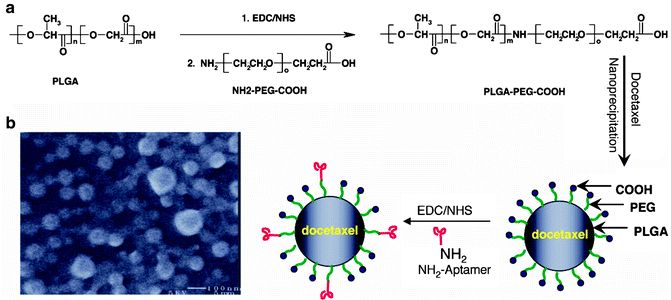
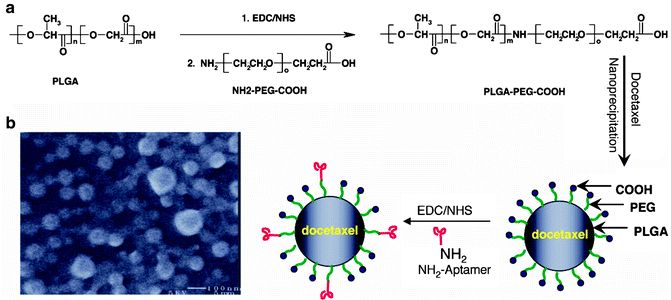
Fig. 15.4
Co-delivery of Dtxl and Pt(IV)-monosuccinate prodrug. From Kolishetti et al. [29], with permission. Copyright 2010 National Academy of Sciences USA
In addition to delivering multiple types of drugs using Apt–NP bioconjugates, NP systems have also been developed that are capable of simultaneous delivery of both hydrophobic and hydrophilic drugs. A novel targeted drug delivery system consisting of NP–Apt bioconjugates that can simultaneously deliver both a hydrophobic taxane and a hydrophilic nucleic acid intercalating drug to cancer cells was recently developed by Zhang et al. [30]. PSMA expressing LNCaP prostate adenocarcinomas were chosen as the target cell line for in vitro testing, and PC3 prostate adenocarcinomas, which do not express the PSMA antigen, were employed as a negative control [30]. To visualize cell uptake of drugs using fluorescence microscopy, a hydrophobic fluorescent probe, NBD cholesterol, was encapsulated within the PLGA-b-PEG NPs since this dye has properties similar to a hydrophobic drug, and Dox was chosen due to its fluorescence emission spectrum which is in the red region and which can therefore be used for visualization of the NPs in the cells [30]. Both NBD and Dox were effectively delivered to LNCaP cells by NP-Apt bioconjugates with minimal signal observed from the control PC3 cells. In vitro cellular cytotoxicity of targeted NP-Apt bioconjugates carrying both Dtxl and Dox [(Dtxl)-Apt(Dox)], Dtxl alone [(Dtxl)–Apt], Dox alone [NP-Apt(Dox)], or no drug [NP-Apt] on LNCaP and PC3 cell lines was then investigated [30]. MTT cell proliferation assay results revealed that for LNCaP cells treated with the same dose of drugs, the [(Dtxl)-Apt(Dox)] NPs were most cytotoxic in comparison to the other controls [30]. These results demonstrated that co-delivery of Dtxl and Dox could potentially be more cytotoxic than the single delivery of either drug alone. Further studies are required to investigate the release kinetics of each individual drug in combination therapies and the results of this action on combinatorially administered drug encapsulating NPs. As such the further optimization of targeted NPs may allow co-delivery of two distinct classes of drugs with varying properties for PCa therapy, and could potentially allow the delivery of different drugs to distinct subcellular compartments.
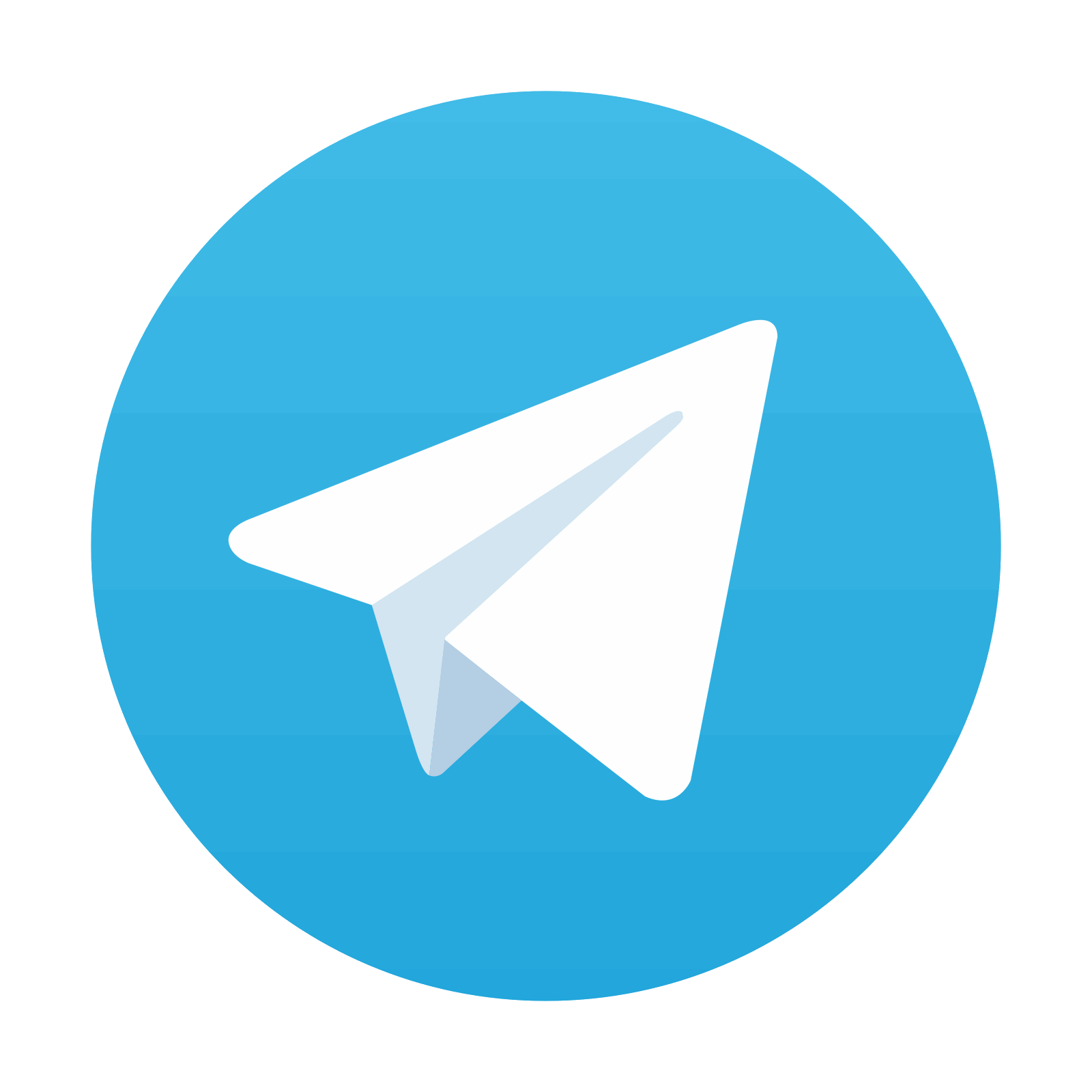
Stay updated, free articles. Join our Telegram channel

Full access? Get Clinical Tree
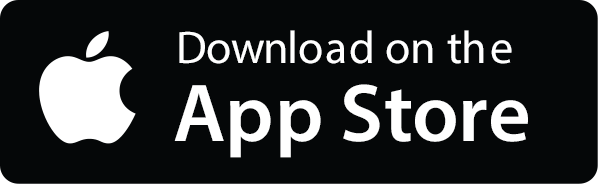
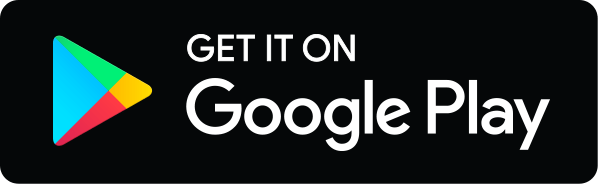