Neurologic Imaging
Dominique Delbeke
Carlos Cunha
Radiopharmaceuticals
Any radiopharmaceutical injected as a bolus in a dose –20 mCi of technetium-99m (99mTc) for an adult—that provides a high enough count rate to perform dynamic imaging—will allow evaluation of blood flow to the brain. A dynamic flow study is performed by acquiring sequential images rapidly over time, typically every 2 seconds. A flow study can be performed in only one projection—the anterior projection when the brain is evaluated. Technically, the bolus injection can be suboptimal if the patient is hypotensive or bradycardic. If the camera is started too late into the injection, the critical arterial phase of the flow study may be missed.
Two categories of radiopharmaceuticals are used to image the brain: the conventional radiopharmaceuticals, which do not cross the blood–brain barrier and the lipophilic chelate tracers, which do cross it and fix in the brain proportionally to perfusion.
The functional basis of conventional brain imaging is that most intracranial lesions will alter the blood–brain barrier and the radiopharmaceutical will leak from the capillaries into or around the lesion. The lesion appears as a “hot spot” in the normal low background of the brain. A conventional brain study with glucoheptonate includes a dynamic flow followed by immediate static images and, up to 4 hours, delayed images in anterior, posterior, and both lateral projections.
99mTc-hexamethylpropylene amine oxime exametazime (99mTc-HMPAO, Ceretec, GE Healthcare), and 99mTc-ethyl cysteinate dimmer (99mTc-ECD, Neurolite, Merck Dupont Inc.) are lipophilic chelate radiopharmaceuticals of cerebral perfusion that are now available and approved by the Food and Drug Administration (FDA). They have a high extraction fraction and are taken up by the brain proportionally to perfusion. There is a very stable pattern of uptake within a few minutes and slow or no washout from the brain over time. Cerebral uptake of 99mTc-HMPAO correlates well with cerebral perfusion measured with labeled microspheres up to 200 mL/100 g per minute. A limitation of 99mTc-ECD is that the uptake does not increase linearly with perfusion at high flow rates—a limitation for indications such as ictal imaging of epileptic patients. 99mTc-ECD is stable for 6 hours after preparation, an advantage over 99mTc-HMPAO, which must be injected within 30 minutes after preparation. The stability of 99mTc-HMPAO can be increased to 4 hours by the addition of methylene blue, which is required for ictal imaging. The procedure to prepare the 99mTc-HMPAO stabilized with methylene blue is explained in the kit’s package insert (Ceretec, GE Healthcare).
When a brain perfusion study is indicated, 20 mCi of 99mTc-HMPAO or 99mTc-ECD is injected intravenously, and SPECT images are acquired between 10 minutes and 2 hours after injection.
Iodine-123 (123I)-iodo-amphetamine (IMP) and xenon-133 (133Xe) are also tracers of cerebral perfusion. 123I-IMP is not widely available and therefore is rarely used for clinical purposes. 133Xe is a gas and less practical to use than 99mTc-radiopharmaceuticals; in addition 133Xe images are of relatively low resolution with conventional gamma cameras. 133Xe has the advantage of short acquisition time, true quantitation of cerebral perfusion, and rapid washout time—a useful feature in performing challenge tests.
The glucose metabolism of the brain can be evaluated using fluorine-18 (18F)-fluorodeoxyglucose (18F-FDG) as radiopharmaceutical. 18F is a positron emitter that requires coincidence imaging by positron emission tomography (PET) to obtain optimal images. 18F-FDG is transported into the cells by the same mechanism as glucose; it is then phosphorylated by a hexokinase into FDG-6-phosphate. As the brain tissue does not have a glucose-6-dephosphatase, FDG-6-phosphate does not progress into further glycolytic pathways; it accumulates in proportion to the glycolytic rate of the cells. The cortex of the brain normally uses only glucose as its substrate; therefore 18F-FDG accumulation is high. Because PET systems provide for soft tissue attenuation and are calibrated with an external source of known activity of germanium-68 (68Ge), true count rates can be measured over a region of interest. Quantification of the actual metabolic rate using kinetic modeling is possible but requires dynamic scanning after injection of 18F-FDG and dynamic arterial blood sampling to obtain both tissue and plasma tracer concentration.
True quantitative measurements are extremely useful in the investigation of the physiopathologic mechanisms of neuropsychiatric diseases and can be performed with 18F-FDG but also a variety of radiopharmaceuticals labeled with positron emitters. This approach, however, is time-consuming, cumbersome, and more invasive than obtaining a static image after 18F-FDG reaches a plateau, usually 45 minutes following IV injection. Measurement of the absolute metabolic rate is rarely performed clinically. Semiquantitative evaluation using asymmetry indices or ratios to reference structures of the brain are usually satisfactory for diagnostic purposes. When an 18F-FDG study is indicated, 10 mCi of 18F-FDG is injected intravenously and PET images of the brain are acquired after 45 minutes of distribution time. The images are corrected for attenuation with a transmission scan using an external source of 68Ge (measured attenuation) or x-ray source (CT transmission) or with a calculated attenuation using the ellipse model.
To interpret tomographic images (both SPECT and PET), it is critical to have the software to reorient the images along the anterior commissure–posterior commissure (AC–PC) line and to examine the three projections (axial, coronal, and sagittal). If two scans have to be compared (ictal and interictal, baseline and acetazolamide, pre- and posttherapy or intervention, etc.), similar orientation slice thickness and windowing is critical. Comparison with the current MRI or CT films is also critical if there is a structural abnormality.
Cerebrospinal fluid (CSF) dynamics can be studied by intrathecal injection of radiopharmaceuticals. Indium-111 (111In)-DTPA is used to evaluate hydrocephalus and CSF leaks, both requiring 24 and possibly 48 hours of imaging. To evaluate the CSF dynamics, 0.5 mCi of 111In-DTPA is injected intrathecally and images are obtained immediately over the spinal canal to document the quality of the injection and then at 4, 24, and 48 hours over the head in the anterior, posterior, and both lateral projections until the radiopharmaceutical
reaches the convexity. 99mTc-DTPA can be used to evaluate the patency of shunts because of the shorter imaging time.
reaches the convexity. 99mTc-DTPA can be used to evaluate the patency of shunts because of the shorter imaging time.
Normal Distribution and Variants
Perfusion and glucose metabolic images of the brain have a similar appearance except in pathologic circumstances when there is decoupling of perfusion and metabolism. The adult pattern of uptake is established by the age of 20. The normal perfusion of the gray matter is approximately 70 mL/min per 100 g and that of the white matter 20 mL/min per 100 g. In the newborn, the perfusion and metabolism of basal ganglia, visual cortex, and sensorimotor cortex is close to that of an adult; there is, however, decreased perfusion to the frontal and parietotemporal cortex, giving an immature pattern of perfusion and metabolism (1).
The weight of the brain decreases approximately 10% between the ages of 30 and 75, and cerebral blood flow decreases by 20%, probably because of neuronal loss and replacement by gliosis. On CT and MRI, this is demonstrated by progressive cortical atrophy with age.
In normal individuals, both the blood flow pattern and metabolism are influenced by certain drugs. Methylxanthines, such as caffeine, and sedatives decrease the blood flow and brain metabolism. If a patient needs sedation for the scanning period, sedatives should be administered no earlier than 20 minutes after the administration of the radiopharmaceutical.
The CSF is secreted by the choroid plexus and flows from the lateral ventricles into the third and fourth ventricles and out into the posterior compartment of the spinal subarachnoid spaces through the foramen of Lushka and Magendie. The CSF then flows in the cephalad direction into the anterior compartment of the spinal canal to reach the basal cisternae. When it reaches the convexities, it is reabsorbed by the arachnoid granulations. When a radiopharmaceutical is injected by lumbar puncture, it normally reaches the basal cisternae by 1 hour, the frontal poles and sylvian fissure by 2 to 6 hours, the cerebral convexities by 12 hours, and the arachnoid granulation in the sagittal sinus region by 24 hours. There is normally no reflux into the ventricular system.
Indications
Brain Death (2)
Brain death is a clinical diagnosis taking into account both cerebral and brainstem function. Confirmatory tests such as electroencephalography (EEG) and imaging studies assessing blood flow to the brain give evidence for or against the clinical impression. With the widespread use of brain metabolic suppressive therapy, cerebral blood flow imaging is more accurate than EEG. The guidelines published in JAMA in 1981 recommend a test of cerebral blood flow to confirm brain death. Radionuclide blood flow imaging is noninvasive and can be done portably, giving it two major advantages over angiography (2,3,4,5).
If 99mTc-glucoheptonate is used as a radiopharmaceutical, the interpretation relies entirely on the dynamic flow scan and the quality of the bolus injection. As the flow scan is obtained in the anterior projection, only flow to the anterior and middle cerebral arteries can be assessed. The posterior circulation must be evaluated clinically. In most cases, however, brain death is due to increased intracranial pressure, and the circulation of the entire brain is affected to the same extent.
99mTc-HMPAO and -ECD offer the advantage of accumulating within the brain tissue itself. If the dynamic flow images are of poor quality, delayed images can be obtained in multiple projections; even single photon emission tomography (SPECT) can be performed if necessary, offering the advantage of assessing perfusion to the entire brain, including the posterior fossa (6).
Herpes Encephalitis
It is important to make the diagnosis early in the disease because there is a treatment available with acyclovir. Nowadays, this may be the only indication remaining for brain imaging with 99mTc-glucoheptonate, because the images are abnormal days before the CT scan. The most specific sign of herpes encephalitis is focal temporal uptake, but uptake in this location is found in only 50% of the patients. The remainder have more diffuse frontal or parietal uptake.
Infarction
The etiologies of a cerebral infarction are multiple: thrombotic, embolic, and hemorrhagic, among others. CT and MRI have replaced radionuclide imaging with the conventional radiopharmaceuticals for several decades. Functional images are usually abnormal before anatomic images, because the physiologic dysfunction of an organ precedes the resulting anatomic changes. Positron emitters include oxygen-15 (15O, half-life = 2 minutes), nitrogen-13 (13N, half-life = 10 minutes), carbon-11 (11C, half-life = 20 minutes), and fluorine-18 (18F, half-life = 2 hours) and require coincidence detection with a PET scanner for imaging. These radioisotopes have the advantage of being naturally part of the molecular structure of endogenous substrates. PET studies using 15O-H2O to evaluate perfusion, 15O-O2 to evaluate oxygen metabolism, and 18F-FDG to evaluate glucose metabolism have contributed to the understanding of the physiopathology of cerebral infarction. Because of the short half-lives of the positron emitters, these PET radiopharmaceuticals are not practical for clinical use except for 18F-FDG. 99mTc-HMPAO and -ECD, however, allow evaluation of cerebral perfusion using the SPECT technique. SPECT images with perfusion radiopharmaceuticals can demonstrate a defect of perfusion as early as 2 hours after the onset of symptoms and before the appearance of positive findings on CT or MRI, which can take a day or more. The CT scan becomes abnormal immediately only in case of hemorrhagic stroke. The size of the defect on early SPECT perfusion images (6 hours after the onset of symptoms) seems to have the best prognostic value for the outcome of these patients.
Within the first hours to days after a stroke, there is decreased relative perfusion compared to the glucose and oxygen metabolism, a phenomenon called “misery perfusion.” Twenty-four hours to a week after infarction, the perfusion usually improves but the symptoms and crossed cerebellar diaschisis persist. There is decoupling of metabolism and perfusion, a phenomenon called “luxury perfusion,” which may last 1 to 10 days and is thought to be due to local accumulation of radicals. The luxury perfusion has usually resolved a month after the onset of infarction. In addition, there is a “penumbral zone” surrounding the infarcted area, which is ischemic and demonstrates decreased perfusion but increased oxygen extraction fraction. If perfusion to the penumbral zone can be restored, irreversible damage will not occur.
There are regions that show decreased perfusion and metabolism that are distant to the region of infarction. For example, cortical infarcts are usually associated with decreased uptake in the contralateral cerebellar hemisphere (crossed cerebellar diaschisis) owing to deafferentation, a phenomenon that occurs when the impulses through the corticopontocerebellar fibers fail to be transmitted and stimulate the contralateral cerebellar hemisphere. Other areas that can demonstrate decreased perfusion and metabolism are the ipsilateral thalamus and caudate nucleus. Infarction of specific nucleus from the thalamus results in cortical hypometabolism, while infarction of other nuclei does not, owing to specific thalamocortical tracts.
Transient Ischemic Attack
Transient ischemic attacks (TIAs) can produce the symptoms of a stroke but resolve within 24 hours. However, 60% of patients with TIAs will develop a stroke later. CT images are usually normal after a TIA, but SPECT perfusion images can be abnormal in as many as 60% of the patients in the first 24 hours and 40% a week later. In patients with TIA and a normal baseline SPECT (99mTc-HMPAO, 99mTc-ECD) perfusion scan, evaluation of the cerebral vascular reserve using the acetazolamide challenge test may show the ischemic area.
Estimation of Cerebrovascular Reserve (8)
Evaluation of cerebral perfusion reserve can be performed using acetazolamide (Diamox), an inhibitor of carbonic anhydrase, to vasodilate the capillaries of the brain. Acetazolamide is administered at a dose of 1 g intravenously 30 minutes before administration of the radiopharmaceutical. CO2 can be used as well but is less practical. In normal individuals, perfusion to the brain increases by 30%. The perfusion increases to a lesser extent in regions of the brain supplied by abnormal vessels that cannot vasodilate. An absolute decrease in perfusion does not occur unless there is a steal phenomenon (e.g., arteriovenous malformation).
Arteriovenous Malformation
Arteriovenous malformations are not seen on SPECT perfusion images but can shunt blood from the normal vasculature and create a steal phenomenon that can induce ischemia. The ischemic zone can be demonstrated on SPECT (99mTc-HMPAO, 99mTc-ECD) perfusion images. After treatment, restoration of normal perfusion pressure in vessels previously submitted to low pressure, because of the steal phenomenon, may lead to their rupture. A challenge test with acetazolamide helps identify territories with enhanced vasoreactivity that are at risk for hyperemic disturbances. SPECT perfusion images can also help monitor the treatment with embolization (8).
Evaluation of Adequacy of Collateral Flow during Balloon Occlusion Test before the Projected Sacrifice of a Large Vessel
Occasionally, it is necessary to sacrifice a large vessel such as a carotid artery. This may occur due to trauma, large tumor encasing the vessel, or treatment of arteriovenous malformation. To assess the adequacy of collateral flow, an intra-arterial balloon catheter is inflated in the vessel at the level of projected ligation. The patient is observed for neurologic deficits with the balloon inflated for 45 minutes, and if signs and symptoms of ischemia develop, the balloon is deflated. Some patients who do not develop symptoms during the balloon occlusion test do develop neurologic deficits after permanent sacrifice of the vessel. Therefore assessment of brain perfusion with 99mTc-HMPAO or 99mTc-ECD during balloon occlusion has been added to the evaluation of these patients (8,10).
Subarachnoid Hemorrhage
Subarachnoid hemorrhage is often due to rupture of an aneurysm. A delayed complication is vasospasm of major vessels, which can lead to ischemia and stroke. SPECT (99mTc-HMPAO, 99mTc-ECD) perfusion images can demonstrate regions of decreased perfusion secondary to vasospasm when the CT images are still normal. SPECT perfusion images can also help to monitor therapy following treatment with balloon angioplasty (9).
Evaluation of Cerebral Distribution of Amobarbital Sodium in the Intracarotid Wada Test
The intracarotid amobarbital, or Wada, test is performed to lateralize both language and memory in patients considered for temporal lobectomy. Amobarbital sodium (Amytal) is injected into a carotid artery to sedate one hemisphere, and neurologic testing is performed. One mCi of 99mTc-HMPAO or 99mTc-ECD can be injected together with the amobarbital sodium to monitor the distribution of amobarbital (11,12).
Head Trauma
Seizures
Seizures may be due to epilepsy or to the presence of an irritating focus such as a neoplasm or infectious process. There are two types of epilepsy: (a) generalized seizures (grand mal and petit mal), thought to be due to abnormal impulses between the neocortex and the thalamic reticular system, and (b) partial seizures, due to sudden depolarization of a focal group of neurons. The partial seizures are called complex if the patient loses awareness or simple if not. Most partial seizures arise from the temporal lobe. More than half of the patients
with partial seizures become refractory to medical therapy. If the seizure focus can be localized and resected surgically, the symptoms improve or are cured. MRI scanning is helpful to localize a structural lesion that may be responsible for the seizure or to demonstrate hippocampal sclerosis. However, often both the MRI and EEG fail to localize the seizure focus. Functional imaging using both SPECT (99mTc-HMPAO, 99mTc-ECD) and PET (18F-FDG) has been demonstrated helpful in predicting the postsurgical outcome of these patients. Both flow and metabolism are increased during the ictal and decreased during the interictal period. 18F-FDG PET imaging appears more sensitive in demonstrating the seizure focus in the interictal state, but 99mTc-HMPAO SPECT imaging is more suitable for ictal administration because it is rapidly taken up by the cells (7,14,15,16,17,18,19,20,21,22,23).
with partial seizures become refractory to medical therapy. If the seizure focus can be localized and resected surgically, the symptoms improve or are cured. MRI scanning is helpful to localize a structural lesion that may be responsible for the seizure or to demonstrate hippocampal sclerosis. However, often both the MRI and EEG fail to localize the seizure focus. Functional imaging using both SPECT (99mTc-HMPAO, 99mTc-ECD) and PET (18F-FDG) has been demonstrated helpful in predicting the postsurgical outcome of these patients. Both flow and metabolism are increased during the ictal and decreased during the interictal period. 18F-FDG PET imaging appears more sensitive in demonstrating the seizure focus in the interictal state, but 99mTc-HMPAO SPECT imaging is more suitable for ictal administration because it is rapidly taken up by the cells (7,14,15,16,17,18,19,20,21,22,23).
Brain Tumors
After being treated for high-grade brain tumors with a combination of surgery, radiation therapy, and chemotherapy, patients often develop neurologic symptoms that may be related to edema and radiation necrosis or recurrent tumor. The changes on CT and MRI are very similar for both etiologies. In the early 1980s, Di Chiro and collaborators were the first investigators to demonstrate the potential for metabolic imaging with 18F-FDG PET in differentiating recurrent high-grade from radiation necrosis (14,24,25). 18F-FDG PET can also differentiate high-grade (uptake well above the level of the white matter) from low-grade glioma (uptake close to that of white matter), and the degree of uptake in high-grade gliomas has a prognostic value (26,27,28,29,30,31,32). In patients with AIDS and brain lesions, 18F-FDG PET can differentiate lymphoma (high 18F-FDG uptake) from toxoplasmosis (low 18F-FDG uptake) (33,34,35).
The SPECT radiopharmaceuticals that can be used to differentiate radiation necrosis from recurrent high-grade tumor and high-grade from low-grade gliomas are thallium-201 (201Tl)-chloride and 99mTc-sestamibi.
In degenerative dementia, structural imaging will either be normal or demonstrate nonspecific cortical atrophy. Functional imaging with PET usually demonstrates a pattern of hypoperfusion or hypometabolism that correlates with different syndromes. A first step in evaluating dementing syndromes is to separate patients on the basis of whether they have signs and symptoms of motor dysfunction (cortical dementias) or not (subcortical dementias). In cortical dementia, the cortex is usually affected and the subcortical structures are spared; the patients do not have motor dysfunction but present with apraxia, aphasia, memory loss, abnormal affect (Alzheimer’s or Pick’s disease). In subcortical dementias, the basal ganglia, thalami, and brainstem are affected; the patients do have motor dysfunction (disorder of posture and tone, tremor, gait disturbance) as well as memory loss, slowing of cognition, and depression. This includes extrapyramidal syndromes (e.g., Parkinson’s disease and syndromes, Huntington’s disease, and Wilson’s disease), white matter disease (e.g., multiple sclerosis), HIV encephalopathy, and normal-pressure hydrocephalus. A mixed category includes conditions that involve both cortical and subcortical structures, such as vascular dementias (e.g., multi-infarct dementia and Biswanger’s disease), infectious dementias (e.g., Creutzfeldt–Jakob), and hypoxic encephalopathy.
Alzheimer’s disease (AD) accounts for 75% of the dementias in the elderly. These patients present with short-term memory loss and visuospatial problems. However, clinically the diagnosis is unclear in a large proportion of these patients. In advanced disease, CT and MRI demonstrate more pronounced atrophy in the temporoparietal and anterior frontal cortex, and sometimes the atrophy is severe in the hippocampus. On both 18F-FDG PET and perfusion SPECT images, there is a characteristic pattern of decreased uptake in the posterior parietotemporal cortex, probably related to neuronic depletion in these areas. These functional changes precede the atrophy seen on structural imaging. Thirty percent of the patients present with a unilateral defect first (often the left); some present with a bilateral defect. Later in the disease, there is also decreased uptake in the frontal cortex (39,40). AD can occur in conjunction with Parkinson’s and Lewy body disease. In these cases, the imaging findings are indistinguishable from AD; histopathologically, the findings are those of AD in addition to Parkinson’s or Lewy body disease. The sensitivity and specificity of posterior perfusion defect for AD are in the range of 90% (41). Posterior perfusion defects mimicking AD can be seen in other pathologies such as normal-pressure hydrocephalus, Creutzfeldt–Jakob disease, and AIDS, among others.
Frontal metabolic and perfusion deficits are a landmark for Pick’s disease but can also be found in multiple-system atrophy, progressive supranuclear palsy, nonspecific frontal gliosis, and chronic alcoholism. Pick’s disease presents clinically with personality changes, emotional disturbances, and deterioration in behavior and judgment.
In Huntington’s disease, there is decreased flow and metabolism in the caudate nucleus. These findings are present in some patients with a positive family history; therefore functional imaging may be helpful in identifying the disease before it is expressed clinically.
PET and SPECT images of multi-infarct dementia usually show multiple cortical defects. The large defects follow a vascular distribution. Sometimes vascular dementia and AD can coexist in one patient.
Numerous studies have been published to investigate various neuropsychiatric diseases—such as affective disorders, schizophrenia, obsessive-compulsive disorder, chronic fatigue syndrome, and chronic pain syndrome—mainly with PET, using 18F-FDG and labeled brain receptors. To date, there are few clinical applications, but they may develop in the future.
Normal-Pressure Hydrocephalus
Normal-pressure hydrocephalus (NPH) is associated with the triad of dementia, incontinence, and ataxia. The ventricles are enlarged and the CSF pressure is normal. On radionuclide cysternography, the classic findings are early entry of the radiopharmaceutical in the lateral ventricles, which persists on 24- and 48-hour images, and impairment of flow over the convexities. Because only 40% to 87% of these patients improve
after ventriculoperitoneal shunting and owing to the high complication rate of this therapy (up to 31%), the diagnosis of NPH using radionuclide cysternography has become debatable (42).
after ventriculoperitoneal shunting and owing to the high complication rate of this therapy (up to 31%), the diagnosis of NPH using radionuclide cysternography has become debatable (42).
Cerebrospinal Fluid Leak
About 80% of cases involving CSF rhinorrhea are the result of direct head trauma, 16% are iatrogenic due to surgery, and 4% are spontaneous. The major complication of CSF leakage is meningitis, occurring in 25% to 50% of untreated cases. Meningitis can occur immediately or several years after the fistula. Therefore treatment of a CSF leak is indicated as soon as it is diagnosed. Radionuclide cysternography with pledget placement is the most sensitive and specific imaging test to detect CSF leakage, especially in symptomatic patients with small or intermittent leaks (42).
Ventriculoperitoneal Shunt Patency
To evaluate shunt patency, 99mTc-DTPA is a satisfactory radiopharmaceutical because images are usually not obtained later than 1 hour after administration into the reservoir of the shunt. If the shunt is patent, the radiopharmaceutical should be seen free in the peritoneal cavity within 30 minutes (42).
Case 4.1
History: This 12-year-old female was admitted for multiple trauma and a history of smoke inhalation. Brain death was suspected, but the patient was in a barbiturate coma. A nuclear medicine brain flow study was performed using 99mTc-glucoheptonate (99mTc-GH) (Fig. 4.1).
Findings: On the dynamic flow images, both anterior and middle cerebral arteries are visualized. There is high background activity present in the brain itself.
Discussion: The findings are indicative of perfusion to the brain. “Brain death” is the irreversible cessation of brain function, including the brainstem. Over the years, there have been alterations in the guidelines based on the Harvard criteria for the diagnosis of brain death. The clinical diagnosis requires the persistence of the following for 6 hours: (a) unreceptivity and unresponsiveness, (b) no spontaneous movements or breathing, (c) no reflexes (including fixed, dilated pupils as well as absence of brainstem reflexes and deep tendon reflexes), and (d) an isoelectric EEG. Current guidelines require exclusion of hypothermia, drug-induced coma/drug intoxication, or reversible metabolic-induced coma, as these conditions can potentially mimic all signs of brain death clinically and are associated with an isoelectric EEG.
A confirmatory test is necessary if the patient suffers from one of the conditions that can mimic brain death; it is also desirable to abbreviate the observation period required to determine the irreversibility of the patient’s condition, which is highly desirable in potential organ donors. The “gold standard” in evaluating cerebral blood flow is a four-vessel contrast angiogram, but this is an invasive and nonportable technique. Radionuclide angiography is a widely accepted and well-established method of determining the presence or absence of intracerebral blood flow. It has the advantage of being an accurate, noninvasive, and rapid test. The study involves only a peripheral venous injection and lasts less than 10 minutes; moreover, the radiopharmaceutical is easily stored and readily available. A clear advantage is the ability to image at the bedside using a portable gamma camera, because the patient is usually in critical condition, requiring intense nursing care, mechanical ventilation support, and a multitude of catheter lines and monitor wires.
Diagnosis: Brain flow present.
Case 4.2
History: A 13-year-old female presented with refractory complex partial seizures and a normal MRI of the brain. The ictal scalp EEG showed ictal discharges predominantly in the right temporal region. An ictal and interictal 99mTc-HMPAO SPECT study of the brain was performed to localize the seizure focus (Fig. 4.2 A).
Findings: On the ictal SPECT images, a focus of increased uptake is seen in the right temporal lobe anteriorly. The interictal SPECT images were obtained 2 days later and demonstrate symmetrical uptake in the temporal lobes.
Discussion: The combination of interictal symmetrical flow and ictal high flow point to the right temporal lobe as the probable site of the seizure focus, especially with concordant scalp EEG findings.
Epilepsy affects 1% of the population in the United States. The seizures can be partial or generalized. If the patient does not lose awareness, the seizures are called “simple partial”; if awareness is lost, they are “complex partial.” When the seizures become refractory to medical therapy, surgical removal of the seizure focus can be curative. In patients considered for surgery, precise localization of the seizure focus is critical. CT and MRI can identify focal lesions responsible for the seizure in 40% to 60% of these patients, most commonly low-grade astrocytoma, hamartomas, and ganglioglioma/neuroma. In the remaining patients, correlation of functional imaging using radionuclides with the clinical presentation of the seizure and the EEG findings plays a pivotal role in identifying patients who will improve following surgery. The temporal lobe is the most common focus of partial seizures, and mesial temporal sclerosis is the most common pathologic lesion.
Both 18F-FDG PET and 99mTc-HMPAO SPECT imaging are helpful in evaluating patients with refractory complex partial seizures. 18F-FDG evaluates the glucose metabolic rate and 99mTc-HMPAO evaluates the perfusion. During a seizure, both metabolism and perfusion are increased in the seizure focus; between seizures, both are decreased. Functional imaging in combination with other noninvasive techniques has made monitoring with invasive intracranial electrodes unnecessary in 50% or more patients with temporal lobe epilepsy. Identification of a single focus of interictal temporal lobe hypometabolism on 18F-FDG PET images (asymmetry index greater than 15%) is an excellent predictor of seizure control after surgery (Fig. 4.2 B). The sensitivity of interictal 18F-FDG PET in detecting temporal lobe epileptic foci is in the range of 80%, greater than that of interictal 99mTc-HMPAO SPECT, which is in the range of 60%. Therefore interictal 18F-FDG PET is the functional study of choice.
When interictal 18F-FDG PET imaging is nondiagnostic, an ictal study is indicated. 99mTc-HMPAO is better suited for ictal imaging because the uptake of 99mTc-HMPAO reaches a maximum within a minute after injection. Thereafter, up to 15% is eliminated by 2 minutes postinjection, after which little activity is lost except by physical decay. On the other hand, the uptake of 18F-FDG in the brain occurs slowly, reaching a maximum between 30 and 50 minutes after intravenous injection. To perform ictal imaging, 99mTc-HMPAO must be injected intravenously as a bolus; uptake into the brain occurs within seconds of the start of ictal electrical activity on EEG. Of course the patient must be monitored by EEG, and the syringe with the radiopharmaceutical must be hooked up to the intravenous line and shielded to limit radiation exposure to both the patient and medical staff. Ictal SPECT study will detect an additional 20% to 30% of temporal seizure foci compared with interictal SPECT. The combination of increased flow during an ictal injection and decreased flow during an interictal injection is highly predictive of a seizure focus, with a sensitivity in the range of 90% (18).
Other important technical considerations are the half-life of the radioisotope and the stability of the radiopharmaceutical because seizures cannot be scheduled. The stability of 99mTc-HMPAO is 30 minutes after reconstitution, which is obviously not practical. This stability, however, can be extended to 4 hours with methylene blue. The physical 6-hour half-life of 99mTc requires calibration of the dose in the high range (20 + mCi) so as to ensure enough radioactivity when the patient has a seizure.
The temporal lobe is the most common location for seizure foci, and the frontal lobes are the next most common. Frontal seizure foci are often located in the medial or inferior aspect of the frontal lobes; therefore scalp EEG recordings are often nonlocalizing. Interictal PET was found to be a useful modality to localize frontal lobe foci but appears less sensitive than for the detection of interictal temporal lobe foci. The combination of increased uptake on ictal images and decreased uptake on interictal images is particularly helpful in detecting extratemporal seizure foci. Occasionally functional images demonstrate multiple seizure foci, which is a contraindication to surgery.
Several pediatric syndromes are associated with intractable seizure of extratemporal neocortical origin, often refractory to medical therapy. In infants and children, the progressive nature of epileptic syndrome can lead to cortical maldevelopment and focal sclerosis. The psychological effects of intractable epilepsy are disastrous as well. The recent development of surgical techniques with smaller standard resection (amygdalohippocampectomy, modified anterior temporal lobectomy sparing the lateral cortex, computerized lesionectomy, subpial cortical transection, and corpus callosum partial or total transection) has increased the number of patients contemplated for surgery early in the course of their disease. These syndromes include but are not limited to infantile spasms, Lenox-Gastaut syndrome, and Sturge-Weber syndrome. Infantile spasms can be due to an identifiable cause or cryptogenic. Lenox-Gastaut syndrome is defined by the triad of a 1- to 2.5-Hz spike-wave pattern on the EEG, intellectual impairment, and a multiple seizure pattern. Sturge-Weber syndrome is characterized by facial capillary nevus and ipsilateral leptomeningeal angiomatosis. In all these syndromes, PET, in combination with CT and MRI, has proven useful in localizing the seizure foci and guiding placement of semi-invasive (foramen ovale and epidural) and invasive (subdural grids) electrodes as well as in ultimately deciding the best type of surgery for these patients.
Diagnosis: Seizure focus in the right temporal lobe.
Case 4.3
History: A 60-year-old male with worsening dementia was referred for 18F-FDG PET imaging (Fig. 4.3). The MRI showed mild cortical atrophy (not shown).
Findings: Decreased uptake is seen in the posterior parietotemporal cortex bilaterally. The uptake is preserved in the sensorimotor cortex, subcortical gray matter (basal ganglia and thalami), and cerebellum.
Discussion: Decreased uptake in the posterior parietotemporal cortex is consistent with a degenerative disorder, most likely Alzheimer’s disease (AD). Ten percent of the population over 65 years of age is demented and 50% of those are evaluated in hospitals. The cost of long-term care is tremendous. Over half of these demented patients have AD. These patients present with impairment of memory as well as visual and spatial skills. From the physiopathologic point of view, there is accelerated neuronal death, affecting mainly the hippocampus and the posterior parietal and temporal cortex. It has been demonstrated that variants of the apolipoprotein E allele appear to account for most cases of late-onset AD.
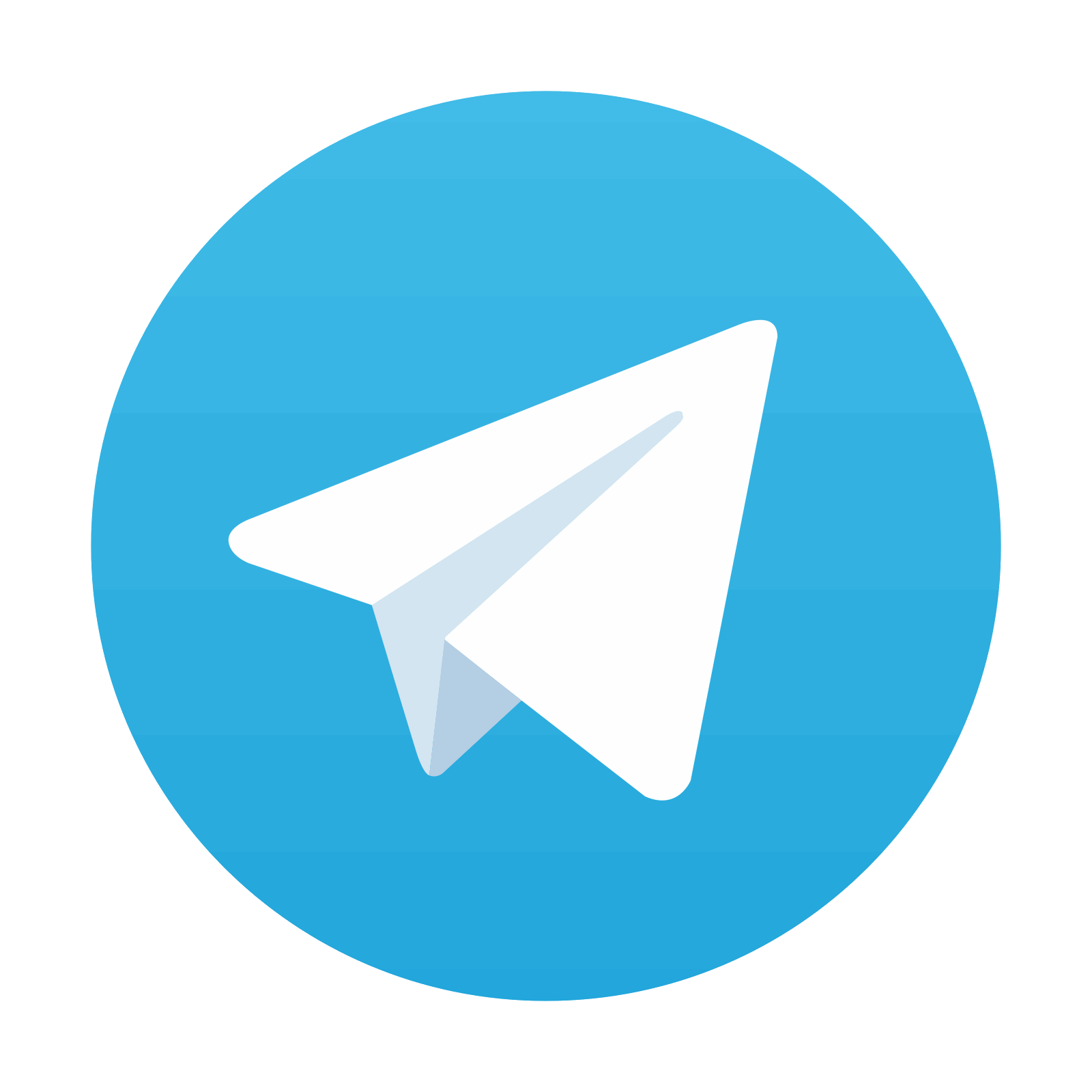
Stay updated, free articles. Join our Telegram channel

Full access? Get Clinical Tree
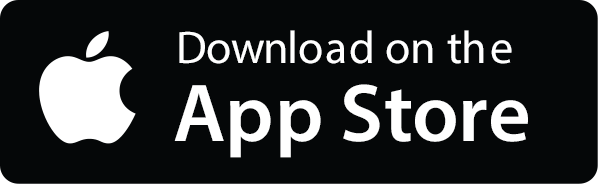
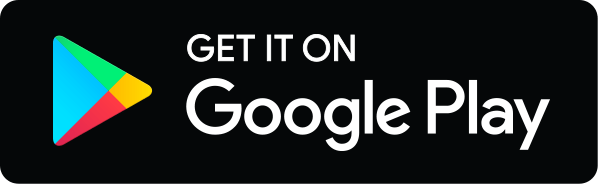