The soft tissue contrast properties of magnetic resonance (MR) allow excellent discrimination of most intrathoracic structures other than the lungs, and allow good insight into normal anatomy. Using MR imaging, the normal cardiorespiratory system, including portions of the lungs and pleural spaces, as well as the mediastinal, chest wall, and cardiac structures can be well depicted. In addition, using newer MR pulse sequences, dynamic ECG-gated imaging can also be achieved, which allows a window into the normal functional processes of these organs.
Functionally, the human chest is composed of the cardiorespiratory system, the main purpose of which is to facilitate gas exchange and deliver oxygenated blood to the remainder of the body.
Anatomically, the chest contains the lungs, which comprise airspace, interstitial spaces and airways; the mediastinum, including the aorta and great vessels as well as the trachea, esophagus, fat and lymph nodes; and the heart and pericardium as well as the pulmonary vasculature. The pleura cover the lungs, the chest wall provides the basic skeleton for the thoracic cage, and the diaphragm provides the muscular support for passage of air through the lungs.
Lung
The normal lung arises embryologically from the primitive foregut. The right lung has 3 lobes, divided by horizontal and oblique fissures, whereas the left lung has 2 lobes, divided by a single oblique fissure. Although the lung fissures are important landmarks on chest radiography and on computed tomography (CT) scans, the paramagnetic properties of air do not allow for good delineation of these anatomic landmarks on magnetic resonance (MR) imaging. Furthermore, for diagnostic purposes, the secondary pulmonary lobule, the basic unit of lung anatomy and basis for high-resolution chest CT, cannot be delineated on MR imaging. These factors make anatomic delineation of disease process of the lungs with MR imaging suboptimal. However, there does remain an important role for disorders that require tissue characterization, such as cystic lesions.
Pleura
The lungs are invested in a 2-layered pleural covering. The visceral pleura envelopes the lung, whereas the parietal pleura forms an exterior membrane. The space between these layers is known as the pleural space. The visceral pleural layer invaginates to form the fissures. The pleural space becomes distended with pleural fluid in several disease processes and can then be seen; however, the pleura are not routinely visualized on MR scanning of the thorax. The role of MR scanning for pleural diseases is important, but largely confined to disorders in which it is important to achieve tissue characterization instead of anatomic delineation.
Pleura
The lungs are invested in a 2-layered pleural covering. The visceral pleura envelopes the lung, whereas the parietal pleura forms an exterior membrane. The space between these layers is known as the pleural space. The visceral pleural layer invaginates to form the fissures. The pleural space becomes distended with pleural fluid in several disease processes and can then be seen; however, the pleura are not routinely visualized on MR scanning of the thorax. The role of MR scanning for pleural diseases is important, but largely confined to disorders in which it is important to achieve tissue characterization instead of anatomic delineation.
Diaphragm
The diaphragms are dome-shaped muscles that descend on normal inspiration, and ascend on expiration. The normal position of the diaphragms is at the fifth to sixth rib anteriorly and the 10th rib posteriorly. The right diaphragm is usually higher than the left. The diaphragmatic position can be depicted using several modalities including plain radiography, CT, and MR imaging. MR imaging has the added benefit of being able to show diaphragmatic motion using cine sequences.
Chest wall
The chest wall forms the structure for the thoracic cage. It comprises bones including the ribs and thoracic vertebral bodies, as well as the intercostal muscles ( Fig. 1 ). The intercostal nerves, arteries, and veins course just inferior to the ribs but cannot be readily resolved on routine imaging sequences, except in pathologic processes. The thoracic musculature can also be depicted on MR imaging including the muscles of the anterior chest (such as the pectoralis muscles) and of the back (such as the latissimus dorsi, and trapezius). The reader is referred to any standard anatomy textbook for further elucidation on musculoskeletal anatomy. The superior contrast resolution of MR imaging makes it well suited for depicting these normal structures. The distinction between fat and muscle planes is well seen on MR imaging ( Fig. 2 ). Knowledge of the MR anatomy of the chest wall is important for determination of disorders, particularly related to the musculoskeletal system.
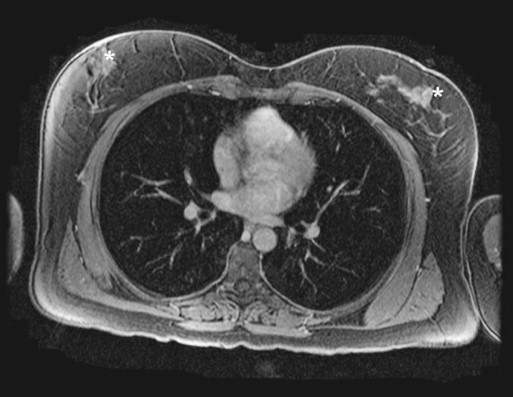
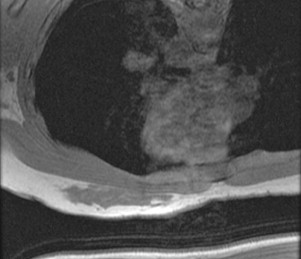
Nonvascular mediastinum
MR imaging can identify several normal nonvascular structures in the mediastinum including the thymus, the trachea, the esophagus, and occasional lymph nodes.
Thymus
The thymus is an arrowhead-shaped structure that occupies the anterior mediastinum. It is usually visible in childhood ( Fig. 3 ), after which it gradually involutes, but may be visible as a normal structure up to approximately 40 years of age. After this point, the thymus is typically completely replaced by fat. The thymus has a right and left lobe that are typically not distinguished by imaging. The MR appearance of thymus mimics the fatty replacement that is seen with progressive T1 shortening with age, whereas the T2 properties remain constant regardless of patient age. Notably, the thymic signal often parallels that of lymph nodes, particularly in those less than 40 years of age, and therefore distinguishing these 2 entities can often prove challenging.
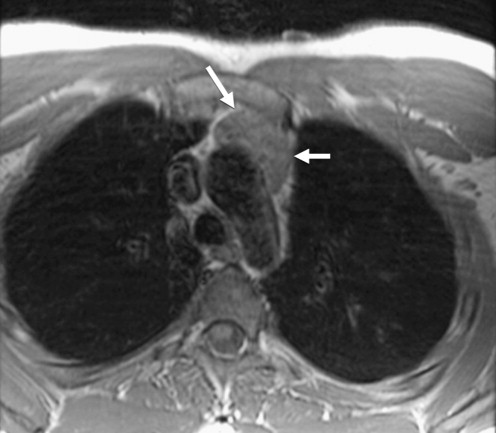
Esophagus
The esophagus is a tubular structure seen in the middle or posterior mediastinum (depending on classification scheme), coursing through the diaphragm and entering the stomach. It is often collapsed in normal thoracic MR imaging and typically has a small amount of air within it. Although MR imaging of the esophagus is not considered a first-line diagnostic modality, there is increasing evidence for use of MR to examine disorders of the gastroesophageal junction such as reflux using cine-MR techniques. The esophagus passes in close proximity to the inferior pulmonary veins, and, because of its close proximity, can be injured during pulmonary vein ablation/isolation procedures, in which case late gadolinium enhancement in that area may be observed.
Trachea and Bronchi
The thoracic portion of the trachea courses from the thoracic inlet and terminates at the carina in the middle mediastinum. The trachea consists of cartilaginous rings anteriorly with a membranous posterior portion. On MR examination, the signal void associated with the tracheal air column can be appreciated, as can the walls of the trachea. The mainstem bronchi and large segmental bronchi can be visualized; however, the smaller subsegmental bronchi, visualized easily on CT, are not readily visualized by MR imaging. The left mainstem bronchus is the hyparterial bronchus, whereas the right mainstem bronchus is the eparterial bronchus, which allows for morphologic delineation of the respective lungs. The tracheal wall can be assessed in pediatric patients to evaluate collapsibility, as well as for stenoses and its relation to vascular structures, using various MR pulse sequences.
Lymph Nodes
MR imaging is not currently the preferred method to assess for lymph node enlargement; however, knowledge of lymph node stations in the thorax is key to avoiding confusion with other processes and other mediastinal structures. A complete review of the most recent American Joint Committee on Cancer (AJCC) lymph node staging system is beyond the scope of this article, and the reader is referred to the official publication for more information. The characterization of lymph nodes on MR is variable on both T1 and T2 imaging. As per CT, size criteria determine the likelihood of malignancy, although work with diffusion-weighted MR imaging may hold promise for increasing specificity for pathologic involvement.
Aorta and great vessels
MR of the aorta ( Fig. 4 ) is a commonly performed examination. MR acquisition can be performed in true planes using double-oblique localizers, and cardiac gating can eliminate motion artifacts ( Fig. 5 ). There are several techniques that are used to image the aorta, including black blood imaging by means of conventional spin-echo and fast spin-echo sequences, bright blood imaging, such as balanced steady-state free precession, time of flight, and phase contrast sequences, as well as gadolinium-enhanced MR angiography sequences ( Fig. 6 ).
The thoracic aorta arises centrally from the left ventricle (LV) and is the main conduit for systemic blood flow, extending to the crus of the diaphragm inferiorly. Initially, the aorta follows a superior course, known as the ascending aorta, followed by a transversely oriented arch, and subsequently a descending portion. From the left ventricular outflow tract (LVOT) and its junction with the aortic annulus, the ascending aorta is divided into the aortic root and the tubular ascending aorta, which is divided by the sinotubular junction ( Fig. 7 ). The proximal 3 cm of the aorta ascends within the pericardium; this portion is most susceptible to pulsatile cardiac motion. The sinuses of Valsalva give rise to the coronary arteries (discussed later). Normal branching occurs at the arch and consists of 3 vessels: a brachiocephalic trunk that subsequently divides into the right common carotid artery and subclavian artery, a left common carotid artery, and a left subclavian artery. Variations in the branching pattern of the aortic vessels are also common. The most common variation is known as a bovine arch, in which case the brachiocephalic trunk and left common carotid share an ostium, and the left subclavian artery has its own branch. The next most common branching pattern involves a left vertebral artery that has a separate origin from the arch, rather than an origin from the left subclavian artery. Bicarotid trunks are a normal variant whereby both carotid arteries originate from a common trunk. An aberrant right subclavian artery is within the spectrum of normal variation, whereby the right subclavian artery is the last branch from the aortic arch, coursing posterior to the esophagus before resuming its normal course. A myriad of other arch variants exist, but a complete review is beyond the scope of this article.
The normal aortic measurements vary with age and body size. There is normal systolic distension of the aorta, which differs significantly from the diastolic. Normative measurement of the aorta is lacking on MR imaging, but has been extensively validated by two-dimensional (2D) echocardiography and, more recently, CT. In general, there is close correlation with 2D-echo results. Consensus data on normal MR measurements of the ascending, arch, and descending thoracic aorta are scarce. At our institution, we use a general rule of 4.0 cm as a top normal size of the aortic sinuses, and 3.8 cm as a top normal for the ascending aorta (specifically at the level of the right pulmonary artery). However, care should be taken when reporting aortic sizes; more accurate correlation with outcomes has been shown when indexing values to body size.
Pulmonary arterial anatomy
The pulmonary arteries function to deliver deoxygenated blood from the right ventricle (RV) to the lungs. The pulmonary arteries arise from the muscular infundibulum of the RV at the pulmonic valve. The main pulmonary artery is an intrapericardial structure that courses superiorly from the right ventricular outflow tract (RVOT). The main pulmonary artery subsequently bifurcates into a longer right pulmonary artery that crosses the midline to supply the right lung, and a shorter left pulmonary artery.
The right pulmonary artery courses posterior to the superior vena cava (SVC) and anterior to the right mainstem bronchus. It then divides into a truncus anterior, supplying the right upper lobe, and an interlobar artery, which supplies the right middle lobe and lower lobe. The pulmonary arteries course with their respective bronchi.
The left pulmonary artery passes anterior and lateral to the left mainstem bronchus and is located higher than the right main pulmonary artery in normal individuals. The left main pulmonary artery divides into a left upper lobe artery and an interlobar artery that supplies the lingula and lower lobe. The blood supply generally follows the adjacent bronchi.
Normative measurements have not been widely reported by MR imaging. One publication measured pulmonary artery diameters in true double-oblique, short-axis measurements. This study by Lyn and colleagues, reported normal main pulmonary arteries between 1.89 and 3.03 cm (fifth to 95th percentiles). This result varied significantly with height and body weight. The pulmonary artery/aorta ratio varied between 0.66 and 1.13.
Pulmonary venous anatomy
Dedicated MR of the pulmonary veins is a commonly performed procedure, owing to pulmonary vein ablation/isolation procedures. Techniques used to image the pulmonary veins include dark blood spin-echo sequences, bright blood sequences, as well as gadolinium-enhanced MR angiography techniques.
The pulmonary veins deliver oxygen-rich blood from the pulmonary capillaries to the left atrium ( Fig. 8 ). Variation in pulmonary anatomy is the rule rather than the exception. Clinically, delineation of the pulmonary vein ostia with the left atrium is most important ( Fig. 9 ). The branching of the pulmonary veins more distally is variable. The most common pulmonary venous anatomy is 2 right-sided pulmonary veins, the superior supplying the right upper lobe, and the inferior supplying the lower and middle lobes, and 2 left-sided pulmonary veins, the superior supplying the left upper lobe ( Fig. 10 ) and the inferior supplying the left lower lobe. The superior pulmonary veins course anterosuperiorly, whereas the inferior pulmonary veins course posteroinferiorly. Normal variants are plentiful in the pulmonary venous system; the most common is a third right-sided pulmonary vein ( Fig. 11 ) that courses anteriorly to supply the right middle lobe, or, less frequently, courses posteriorly to supply the superior segment of the right lower lobe. On the left side, the most common variant is a common trunk arising from the left atrium that subsequently branches to drain the entire left lung. Only rarely is a fifth pulmonary vein seen arising from the left side. Rarer still is the presence of a right top pulmonary vein that arises from the roof of the left atrium and drains an apical portion of the right upper lobe.
Normal pulmonary vein ostia are similar in both right-sided and left-sided pulmonary veins, and similar in both the right and left inferior pulmonary veins. The superior pulmonary veins are typically larger than the inferior pulmonary veins. The proximal pulmonary vein trunks can be either straight or funnel shaped. The branching pattern is also variable, with early branching occurring frequently and of particular importance in planning electrophysiologic interventions.
Heart and pericardium
Imaging Technique
The heart can be imaged using both spin-echo and gradient-echo techniques. Spin-echo acquisitions are black blood sequences ( Fig. 12 ) and can provide important morphologic information. Rapid repetition sequences are used to generate bright blood images and are now predominantly used to generate functional information. Perfusion and delayed enhancement ( Fig. 13 ) images can be generated using separate imaging sequences that are tailored for speed in the former, and more for detail in the latter.
