The use of PET imaging agents in oncology, cardiovascular disease, and neurodegenerative disease shows the power of this technique in evaluating the molecular and biological characteristics of numerous diseases. These agents provide crucial information for designing therapeutic strategies for individual patients. Novel PET tracers are in continual development and many have potential use in clinical and research settings. This article discusses the potential applications of tracers in diagnostics, the biological characteristics of diseases, the ability to provide prognostic indicators, and using this information to guide treatment strategies including monitoring treatment efficacy in real time to improve outcomes and survival.
Key points
- •
New radiopharmaceuticals can enable imaging strategies for the better understanding of disease states in oncology, neurology, and cardiovascular disease.
- •
Oncologic PET imaging agents are intended to probe the biological characteristics of cancers to improve diagnostics and the efficacy of therapeutic strategies.
- •
Nononcology neurologic PET imaging focuses on a range of neurologic disorders with an emphasis placed on neurodegenerative diseases such as Parkinson disease and Alzheimer disease.
- •
Most cardiac PET imaging has focused on blood flow and metabolism and is complemented by additional compounds for imaging of atherosclerosis.
Prominent PET tracers in oncology
This article first discusses many of the most prominent PET imaging agents in late preclinical and early clinical development in oncology. These agents are intended to take advantage of the unique biological and physiologic characteristics of tumors to delineate malignant from normal tissues as a means of improving current methods of diagnosis. In addition, numerous PET imaging agents focus on probing the biological characteristics of cancers and other cell populations of interest in tumors to monitor and predict the efficacy of various therapeutic strategies.
Fibroblast Activation Protein Inhibitors
Cancer-associated fibroblasts and extracellular fibroblasts are among the most abundant cell types in solid cancers. These cells, which dominate the tumor stoma, have been found to play a significant role in regulating the antitumor immune response and thus have been a noteworthy target of interest for both diagnostic and therapeutic applications. , Cancer-associated fibroblasts overexpress the fibroblast activation protein (FAP), which has led to the development of fibroblast-activating protein inhibitors (FAPIs), as a means of selectively targeting these cells to improve therapeutic outcomes. Although therapeutic radionuclides attach via chelators to FAPI and have been evaluated in numerous types of cancer, the focus here is on PET imaging applications. An extensive amount of research has focused on the preclinical and clinical evaluation of variations of the [ 68 Ga]Ga-FAPI PET tracer, including [ 68 Ga]Ga-FAPI-2, [ 68 Ga]Ga-FAPI-4, [ 68 Ga]Ga-FAPI-46, and [ 68 Ga]Ga-FAPI-74 in various types of cancers.
One such prominent study extensively assessed the use of [ 68 Ga]Ga-FAPI-04 as an imaging agent in 28 different types of cancers. Images generated from this study are shown in Fig. 1 . The standard uptake value (SUV) varied depending on the type of cancer, but the investigators reported tumor to background ratios greater than 3 for the moderate uptake groups and more than 6 for the high-intensity uptake groups. In addition, studies have been performed directly comparing the imaging capabilities of [ 68 Ga]FAPI and [ 18 F]-fluorodeoxyglucose (FDG), , , and some physicians argue that this tracer could replace [ 18 F]FDG, especially in cancers where surrounding tissues show high rates of metabolism resulting in high background signal. More recent work has explored the capabilities of an 18 F-labeled FAPI imaging agent as a means of extending the radioactive half-life of the tracer to improve its availability to more remote locations. The results of these studies have shown the potential of these tracers in numerous types of cancer for both diagnostic and staging applications. There are currently 20 active clinical trials exploring the use of FAPI PET imaging agents in various cancers and diseases. There is significant optimism that these tracers could play a prominent role in addressing pitfalls in current standard of care techniques and improve patient outcomes.
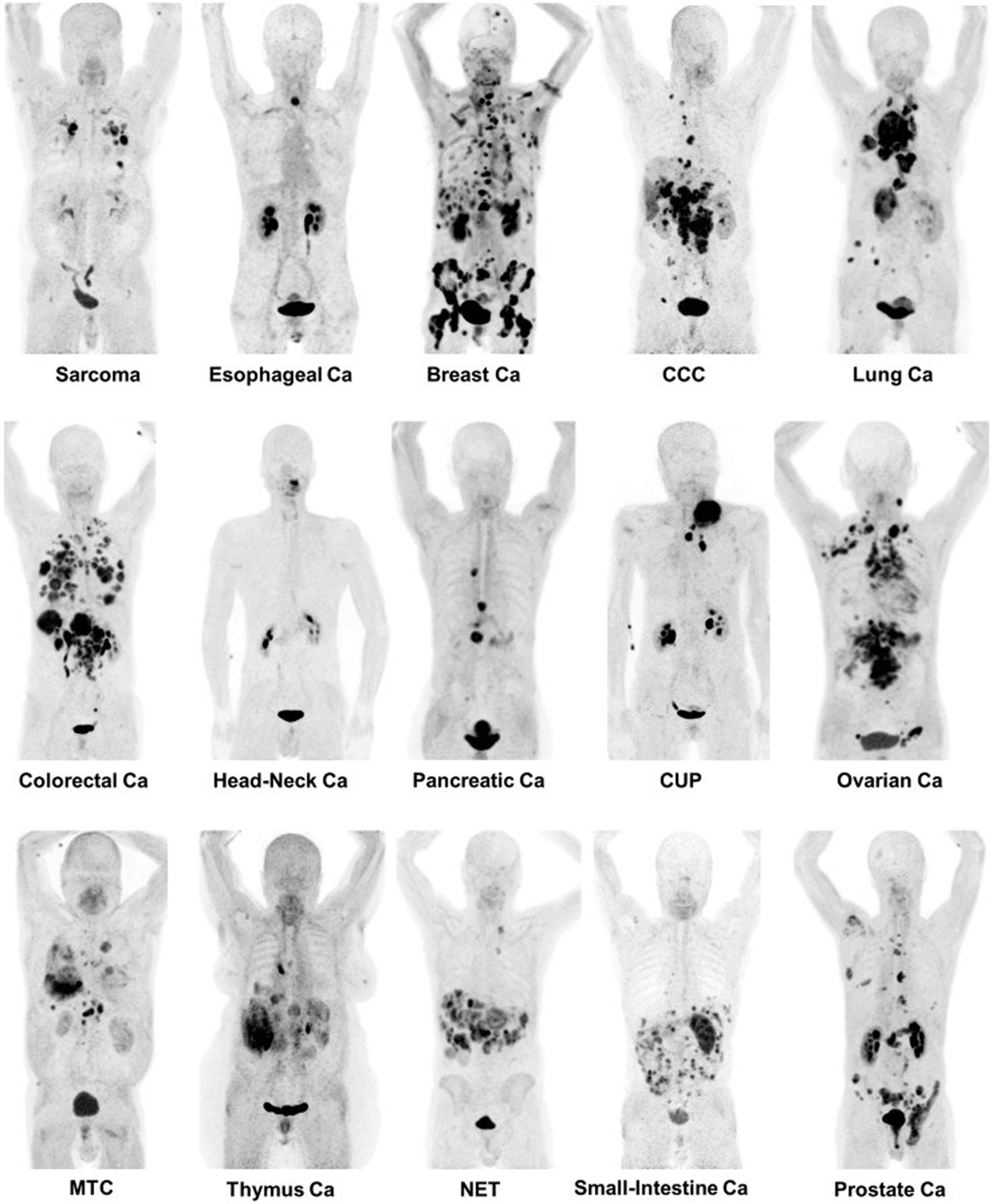
Imaging Increased Rates of Cellular Proliferation
The 18 F-labeled nucleoside analogue 3′-fluoro-3′deoxythymidine (FLT) is a PET imaging agent that has seen significant interest for its ability to quantify cellular proliferation. The capabilities of this tracer were initially reported in 1998, and have since sparked a large number of preclinical and clinical evaluations for its potential in overcoming the challenges associated with [ 18 F]FDG. [ 18 F]FLT has the potential to better delineate malignant tissues in areas with high metabolic rates, such as muscle, lymphocytes, brain tissue, as well as in head and neck cancers. In a clinical evaluation performed by Buck and colleagues, the uptake of [ 18 F]FLT better correlated with staging than [ 18 F]FDG in soft tissue tumors, with mean SUVs of 0.7, 1.3, 4.1, and 6.1 in benign lesions, low-grade sarcoma, grade 2 tumors, and grade 3 tumors respectively.
In addition, the ability to monitor cell proliferation has made [ 18 F]FLT PET a promising tool to noninvasively monitor therapeutic response and predict outcomes in patients with a variety of cancers. Numerous studies have been performed to evaluate the potential of [ 18 F]FLT to predict patient response to several treatment strategies. A recent study explored the use of [ 18 F]FLT PET imaging to determine response to neoadjuvant chemotherapy targeting the c-met pathway in soft tissue sarcomas. Researchers observed, in a small subset of 15 patients, that 12 had observable changes in [ 18 F]FLT accumulation, 8 patients showed response, and 4 progressed. The results of this pilot study support the potential of this tracer to monitor response, and further evaluation of this tracer is currently being explored. [ 18 F]FLT has been evaluated in numerous types of cancer. In some studies in head and neck cancer and non–small cell lung cancer, [ 18 F]FLT performs worse or about the same as [ 18 F]FDG in monitoring therapeutic response. , In other studies in patients with ovarian cancer and lymphoma, [ 18 F]FLT has better performance in determining therapeutic outcomes. , Although results can vary depending on the type of cancer and treatment strategy, [ 18 F]FLT is a prominent imaging agent that has significant potential in improving detection and monitoring therapeutic outcomes.
Prostate-Specific Membrane Antigen
The development of PET imaging agents targeting the prostate-specific membrane antigen (PSMA) has been an extensive area of research over the past decade, generating close to 1000 research articles in the past 2 years alone. PSMA PET imaging has been implemented in the clinic as an improved method of staging and restaging patients as well as to monitor for recurrence and metastatic dissemination after therapy. More recent work has been designed to evaluate [ 68 Ga]PSMA PET imaging for initial diagnosis that could potentially improve detection of early-stage recurrence in patients with only moderately increased levels of prostate-specific antigen. In addition, there is significant interest in using PSMA PET imaging to guide therapeutic strategies, such as radiotherapy and radioimmunotherapy, as well as predict patient outcomes.
One of the most widely used PSMA targeted tracers is [ 68 Ga]PSMA-11, which is the focus of numerous preclinical and clinical studies and has recently been approved by the US Food and Drug Administration (FDA) in patients with suspected metastatic and recurrent prostate cancer. A more novel tracer, [ 18 F]PSMA-1007, is also being evaluated in the clinic and has the advantage of a longer physical half-life compared with [ 68 Ga]PSMA-11. Another PSMA imaging agent in late phase development is [ 18 F]F-2-(3-{1-carboxy-5-[(6-[18F]fluoro-pyridine-3-carbonyl)-amino]-pentyl}-ureido)-pentanedioic acid (DCFPyL). In a study of 262 patients with biochemically recurrent prostate cancer, 91.4% of lesions with an SUV peak greater than 3.5 and 95.5% of lesions with an SUV peak greater than 4.0 were considered malignant on [ 18 F]F-DCFPyL PET.
Although prostate cancer has been the main area of focus in most of the studies performed to date, more recent work has begun to elucidate the significance of PSMA expression in other types of cancers. PSMA expression in the neovasculature of many different cancers has been shown to be increased, and thus recent research has aimed to take advantage of this increased expression in cancers such as breast cancer, non–small cell lung cancer, colorectal cancer, renal cell carcinoma, pancreatic cancer, and highly vascularized gliomas, such as glioblastoma multiforme. , , One such example includes a recent study evaluating the use of [ 68 Ga]PSMA-11 to detect and stage primary and metastatic breast cancer lesions. The researchers reported promising detection rates in lesions identified by [ 18 F]FDG. These results along with numerous preclinical and clinical evaluations support the prominent role PSMA targeted PET tracers may have on the diagnosis and therapeutic outcomes in patients with prostate cancer and other cancers with PSMA expression in their neovasculature.
Deltalike Protein 3
The deltalike protein 3 (DLL3) is an inhibitory ligand of the Notch pathway and is upregulated in 85% of small cell lung cancers and other neuroendocrine cancers, whereas nonneuroendocrine cancers and normal tissues do not express DLL3. Castration-resistant neuroendocrine prostate cancers also show increased expression of DLL3 and thus this ligand has received attention in recent years for its potential as a target in immunotherapies such as rovalpituzumab teserine (SC16LD6.5), and radioimmunotherapies including 225 Ac and 177 Lu labeled anti-DLL3 monoclonal antibodies. Several multicenter clinical trials have been performed exploring the use of rovalpituzumab teserine as a therapy in small cell lung cancer.
The use of PET imaging to guide therapies is of significant interest in these indications because conventional methods of analyzing DLL3 expression are limited. These limitations include insufficient contemporaneous analysis of DLL3 expression, sampling bias resulting from intratumoral and intertumoral heterogeneity, and the inherently high false-negative rate for histopathologic assessment of DLL3 expression. The implementation of PET imaging can overcome many of these limitations and thus has been of significant interest in this field. Sharma and colleagues reported the preclinical evaluation of an [ 89 Zr]Zr-DFO-SC16 PET imaging agent in small cell lung cancer xenografts as a means of noninvasively quantifying DLL3 expression. In this work, statistically significant differences in tumor accumulation were observed between the high and low DLL3-expressing small cell lung cancer xenograft models. In addition, uptake of the [ 89 Zr]Zr-DFO-SC16 correlated with therapeutic response, highlighting the potential of these tracers to predict patient outcome. With the promising results of this preclinical analysis, a clinical trial ( NCT04199741 ) was initiated to evaluate the use of [ 89 Zr]Zr-DFO-SC16.56 to noninvasively monitor DLL3 expression in patients, and is currently ongoing.
Poly(ADP-ribose) Polymerase 1
Poly(ADP-ribose) polymerase 1 (PARP1) is part of a family of proteins tasked with repairing single-strand DNA breaks as part of the base excision repair pathway. With increased rates of metabolism and proliferation, cancer cells are more prone to developing single-strand breaks and thus show increased expression and activity of PARP1. , The increased reliance on DNA repair pathways is a prominent characteristic of many types of cancer, and thus a significant amount of research has been aimed at targeting these pathways to prevent DNA repair, ultimately leading to cell death. , There are currently 4 FDA-approved inhibitors of PARP1: olaparib, niraparib, rucaparib, and talazoparib.
A significant volume of research is focused on the development of imaging agents that are based on these PARP inhibitor (PARPi) scaffolds for their potential applications in cancer diagnosis, patient stratification, and monitoring therapy. As a diagnostic, these imaging agents are particularly focused on improving current standard-of-care modalities such as [ 18 F]FDG, which has limitations in certain areas of the body because of high background signal in normal tissues. Several PARP-selective tracers have been developed and evaluated in preclinical and/or clinical studies, including [ 18 F]FluorThanatrace and [ 18 F]PARPi. The [ 18 F]PARPi tracer was developed based on the FDA-approved olaparib scaffold, and the [ 18 F]PARPi tracer was evaluated extensively in preclinical settings as a diagnostic and a means of monitoring the accumulation and retention of PARPi in the tumor. , This tracer has since been used in clinical trials ( NCT04173104 and NCT03631017 ) for multiple types of cancer and has shown promising results, as shown in Fig. 2 . Continued development of these tracers will likely lead to multiple novel radiotracers that can provide improved contrast in PET images, stratify patients, and identify tumors that are likely to respond to therapies targeting the PARP enzyme.
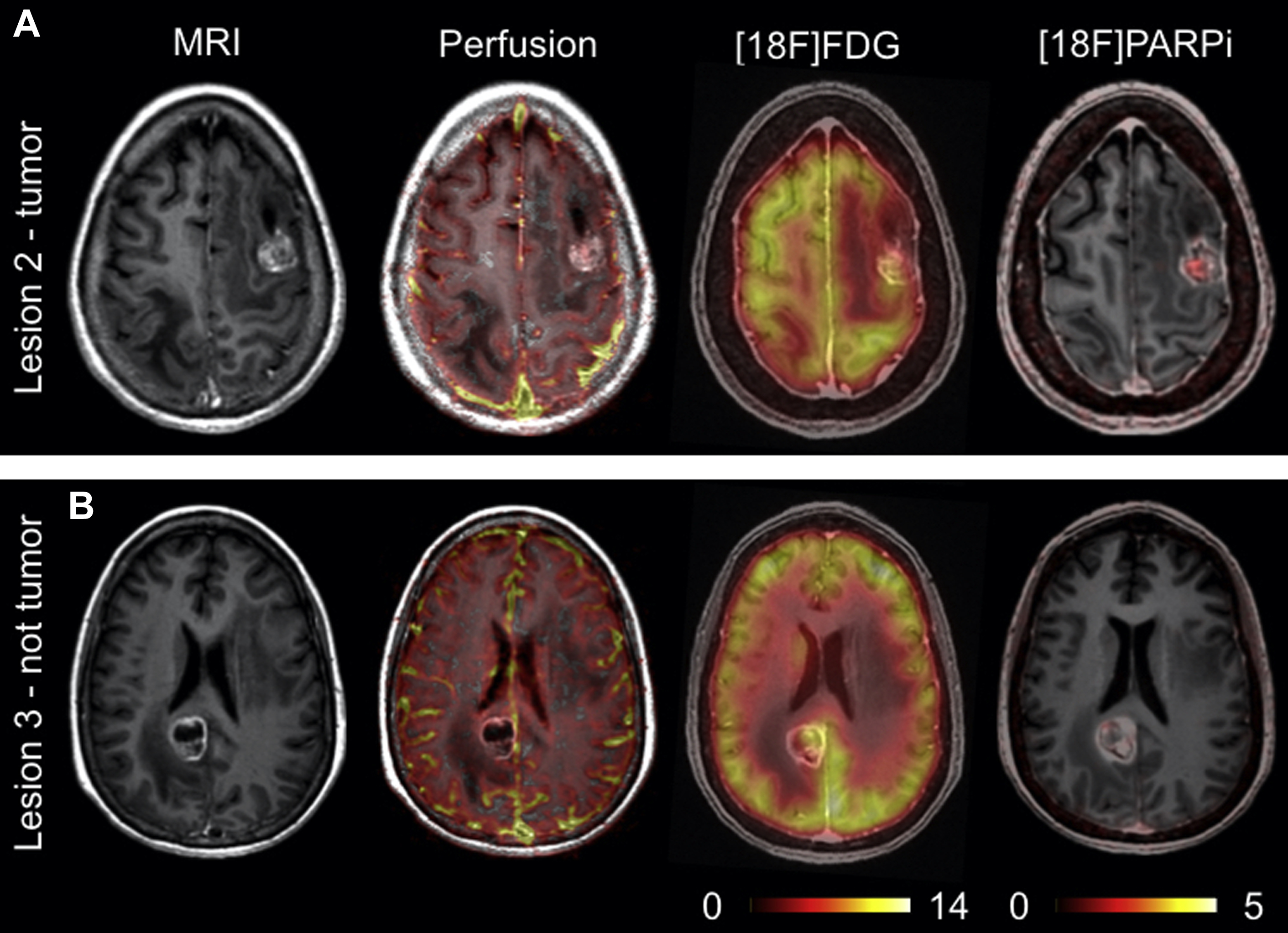
Immune Checkpoint Inhibitors
Immune checkpoint inhibitors such as programmed cell death protein 1 (PD-1)/programmed death-ligand 1 (PD-L1) and cytotoxic T lymphocyte–associated protein 4 (CTLA-4) have received significant attention for their impressive results in some patients. , However, one of the major limitations of these therapies is the lack of a marker that can be used to stratify patients who are likely to respond to immune checkpoint blockade. , There are several possible explanations as to why traditional immunohistochemistry quantification of expression for the ligands that play a role in immune activity does not correlate with patient outcomes. Many in the field believe that heterogeneous expression and the dynamic nature of the ligand expression are the leading factors preventing clinicians from determining whether a patient is likely to respond. , PET imaging has the potential of overcoming these 2 limitations and thus has led to a significant effort to develop an effective PET tracer that can noninvasively monitor expression and correlate well with patient outcomes.
One strategy is to prepare 89 Zr-labeled monoclonal antibodies that selectively bind with the particular immune checkpoint ligand. There are several monoclonal antibodies to date that have been approved by the FDA for use in immune checkpoint therapies. One such monoclonal antibody is pembrolizumab, one of the earliest FDA-approved and widely implemented immune checkpoint inhibitors. There are several researchers who have prepared 89 Zr-labeled versions of the pembrolizumab antibody to evaluate its potential in quantifying PD-1 expression using varying approaches. Several clinical trials ( NCT02760225 , NCT04605614 , and NCT03065764 ) have also been initiated to evaluate PET tracers based on the pembrolizumab antibody in the clinic. Additional clinical trials are also underway for PET tracers based on other FDA-approved antibodies, including ipilimumab, atezolizumab, and avelumab.
Another prominent approach that has seen success in preclinical settings attempts to monitor T-cell activation as a consequence of immune checkpoint therapies using PET/computed tomography (CT) by administering the 18-fluoro-9-(β- d -arabinofuranosyl) guanine ([ 18 F]F-AraG) imaging agent. [ 18 F]F-AraG has been established as selective for activated T lymphocytes, and accumulation of [ 18 F]F-AraG paralleled the course of adaptive immune response in a preclinical colorectal cancer model. Multiple clinical trials are currently investigating the ability of this tracer to monitor T-cell activation in response to immune checkpoint blockade in several types of cancer.
Imaging Extracellular Acidic and Hypoxic Conditions
There are several distinct characteristics of cancer that allow researchers and clinicians to develop novel therapeutic and diagnostic agents that specifically target tumors. One such characteristic is the reduced pH and hypoxic conditions within the extracellular environment of most tumors as a result of the poorly formed vasculature as well as aberrant and increased rates of metabolism and cell proliferation. , This characteristic has led to a significant research effort to devise unique and clever ways to take advantage of this phenomenon. These efforts have brought to fruition numerous pH-sensitive cleavable linkers that can improve the selectivity and efficacy of drug molecules, peptides that can alter their structure as a result of pH and insert themselves into the membrane of cells, and radiolabeled molecules that are metabolized by cells that have altered metabolic pathways as a result of environmental stimuli.
The evaluation of the family of pH (low) insertion peptides has produced promising results and has shown the capabilities of targeting the acidic microenvironment within tumors. These peptides alter their structures in a low-pH environment, forming a transmembrane alpha helix that inserts itself into the cell membrane. , , These peptides have been labeled with several different isotopes for PET imaging, including 18 F and 64 Cu. , The research performed to date evaluating these peptides has focused on their potential as diagnostic agents as well as discerning which patients or tumors are likely to respond to pH low insertion peptide (pHLIP) variants designed for delivery of therapeutic payloads. At present, a clinical trial has been initiated for an 18 F-labeled version of the pHLIP peptide to evaluate its efficacy for imaging the low-pH environments in patients with breast cancer ( NCT04054986 ).
Another strategy for imaging tumor microenvironment focuses on the hypoxic conditions in the extracellular matrix and the variations in cell metabolism that result. , The tracer [ 18 F]fluoro-misonidazole ([ 18 F]FMISO) is reduced by hypoxic cells and accumulates in regions of the body where these conditions exist. Imaging hypoxia has several clinically relevant applications. These agents can act as a companion diagnostic in regions of the body where imaging metabolism using [ 18 F]FDG is insufficient in diagnosing cancer and/or the presence of hypoxia could alter the therapeutic plan. Imaging hypoxia can act as a prognostic indicator and guide therapeutic strategy, and also has a significant impact on radiotherapy where hypoxic cells are much more radiation resistant than normoxic cells, thus requiring increased radiation dose to obtain sufficient therapeutic efficacy. Although [ 18 F]FMISO seems to be the most prominent tracer currently being explored, many other tracers are also in development, including [ 18 F]fluoroazomycin arabinoside ([ 18 F]FAZA), with numerous ongoing clinical trials such as NCT03418818 , NCT04395469 , and NCT02701699 , and [ 64 Cu]Cu-diacetyl-bis(N4-methylthiosemicarbazone) ([ 64 Cu]Cu-ATSM) also with ongoing clinical trials NCT03951337 .
Radiolabeled Analogues of Bioactive Molecules
As researchers continue to elucidate the complex mechanisms involved in cancer biology and metabolism, many novel radiotracers have been developed based on bioactive molecules such as amino acids, hormones, and antibodies that target or interact with processes more specifically associated with cancer. Many of the tracers mentioned previously would be considered among this class of tracers. Although a complete list of novel and promising PET tracers is beyond the scope of this article, briefly, the following tracers are examples of this diverse and powerful field and will likely make their way into clinical settings in the very near future if they have not already.
The PET tracers 3,4-dihydroxy-6[ 18 F]fluoro- l -phenylalanine ([ 18 F]FDOPA), L-[ 11 C]methionine ([ 11 C]MET), and O-(2-[ 18 F]fluoroethyl)- l -tyrosine ([ 18 F]F-FET) are amino acid analogues of particular interest for their potential in improving diagnosis of primary brain tumors, including glioblastoma. These tracers improve on current standard of care because they are capable of passing through an intact blood-brain barrier and have much improved tumor/normal brain uptake ratio compared with [ 18 F]FDG. Each of these tracers is currently under investigation in ongoing clinical trials. Another example of radiolabeled amino acids is the (4 S )-4-(3[ 18 F]fluoropropyl)- l -glutamic acid ([ 18 F]F-FSPG) PET tracer. This tracer is of particular interest for its ability to determine drug resistance because the tumor uptake can indicate the upregulation of antioxidant pathways. This tracer has been shown to provide an early indicator for tumor response in preclinical studies, preceding other standard methods such as tumor size regression or reduced glucose metabolism. These results have led to several clinical trials that are evaluating the ability of these tracers to act as a diagnostic and monitor therapeutic response in patients.
A tracer that uses a radiolabeled hormone analogue is the 16α-[ 18 F]fluoroestradiol ([ 18 F]F-FES), which is capable of providing a method of noninvasively assessing estrogen expression within a tumor and was approved by the FDA in June of 2020. This tracer has been studied as a diagnostic for recurrent and metastatic breast cancer in patients with a history of estrogen-positive primary cancer. , Ongoing clinical trials, such as NCT02398773 , are evaluating the ability of [ 18 F]FES to improve current diagnostic techniques for assessing recurrent and metastatic breast cancer as well as predict patient response to endocrine therapies. Additional hormone analogues currently under study include 21-[ 18 F]fluoro-furanyl-norprogesterone ([ 18 F]FFNP) as a means of evaluating progesterone expression in breast cancers ( NCT03212170 ), as well as the 16β-[ 18 F]fluoro-5α-dihydrotestosterone ([ 18 F]F-FDHT) to diagnose recurrent and metastatic prostate cancer and evaluate androgen receptor expression to guide therapeutic strategies. These tracers have provided promising results with high selectivity and sensitivity, noninvasive quantitation of receptor expression to inform therapeutic strategies, and the capability of monitoring changes in hormone receptor expression as a result of endocrine therapies.
Antibodies and the various fragments that can be engineered from portions of the antibody is an expansive and invaluable area of research that has generated an extensive and ever-growing library of highly specific therapeutic and diagnostic agents that have seen tremendous success in preclinical and clinical studies. The investigators of the Antibodies to Watch series presented an excellent breakdown of the current field of antibody-based therapeutics for treatment in multiple diseases from academic and industrial laboratories that are at varying stages of clinical development. As antibodies are evaluated and confirmed to be effective therapeutics, novel PET imaging agents can readily be prepared by labeling these antibodies with chelators capable of incorporating radioactive isotopes. Thus, as the number of viable and effective therapeutic antibodies continues to grow, so does the library of imaging agents with the potential of improving diagnostic capabilities, stratifying patients who are more likely to respond to therapy, and more quickly and accurately monitoring patient response. Some examples of approved antibodies that have been adapted as PET imaging agents include [ 89 Zr]Zr-durvalumab for imaging PD-L1 expression in head and neck cancers as well as lymphoma ( NCT03610061 , NCT03829007 ), [ 89 Zr]Zr-ramucirumab for imaging VEGFR-2 expression in prostate cancer, and [ 64 Cu]Cu-Bn-NOTA-hu14.18K322A, a humanized version of the chimeric antibody dinutuximab, used to image disialoganglioside GD2 expression in neuroblastoma and osteosarcoma. In addition, some of the previously discussed imaging agents were developed from approved antibodies such as [ 89 Zr]Zr-atezolizumab ( NCT04564482 , NCT03850028 , NCT04222426 ), [ 89 Zr]Zr-avelumab ( NCT03514719 ), and [ 89 Zr]Zr-ipilimumab ( NCT04029181 , NCT03313323 ).
Radiopharmaceuticals for PET imaging in neurology
Highlighted here are the radiopharmaceuticals, for nononcology neurologic PET imaging, that are either FDA approved or in late-stage clinical trials. Nononcology neurologic PET imaging focuses on a range of neurologic disorders, , with an emphasis placed on neurodegenerative diseases such as Parkinson disease (PD) and Alzheimer disease (AD). Such neurodegenerative disorders are notable for the accumulation of protein inclusions in the brain and their impact on neurotransmission.
To illustrate this point, patients with PD experience a degeneration of the nigrostriatal pathway that results in the loss of dopaminergic neurons. This neuronal loss has been shown to correlate with the aggregation of protein α-synuclein in the neuronal perikarya, forming the characteristic Lewy body. In addition, the primary pathology features of AD is the aggregate burden of 2 proteins: β-amyloid, the principal component of neurotic plaques, and tau protein, a component of neurofibrillary tangles. Impairment of the cholinergic system is also thought to play a significant role in the cognitive decline experienced by patients with AD.
These unique disease indications, in combination with the chronic inflammation they produce, are used as potential targets in the development and use of radiopharmaceuticals for the PET imaging of neurologic disorders.
Protein-Targeted Imaging
One of the primary pathology features in neurodegenerative diseases such as PD and AD is protein aggregation. Thus, protein-targeted imaging is an especially notable modality in AD, which has a significant aggregate burden of amyloid-beta (Aβ) plaques and tau protein. Note that preclinical targeting of the PD aggregate protein α-synuclein has met with limited success to date, with recent efforts showing promise.
The clinical relevance of imaging agents targeting Aβ plaques and tau protein in AD are discussed next.
β-Amyloid imaging agents
The accumulation of β-amyloid into Aβ plaques is one of the hallmarks of AD. The first widely used PET imaging agent for detecting these plaques, [ 11 C]PiB (2-[4-([ 11 C]methylamino)phenyl]-1,3-benzothiazol-6-ol; Pittsburgh compound B), continues to have widespread use in research ever since its first use in human studies in the early 2000s. Although this tracer is not approved for clinical use by the FDA, it is notable for its high specificity for Aβ plaques and ability to differentiate AD from other types of neurodegeneration that do not involve Aβ deposition, such as frontotemporal lobar degeneration (FTLD). For this reason, [ 11 C]PiB has been used as a comparative standard in the development of new β-amyloid imaging agents. There is also increasing evidence for the presence of comorbidities in neurodegenerative disorders, such as the presence of Aβ plaques in some patients with PD. , [ 11 C]PiB is being used in clinical trials to measure the amyloid burden in PD ( NCT03555292 ), to evaluate the potential presence of comorbid AD. The results of this trial could provide unique clinical insight into the disease burden of Aβ plaques in PD and other types of neurodegeneration.
Beyond [ 11 C]C-PiB, 3 18 F-labeled amyloid imaging agents have been approved for use by the FDA, within the last decade, for the detection of Aβ plaques in patients undergoing evaluation for cognitive impairment with AD as a potential cause. These 3 tracers are [ 18 F]florbetapir (4-[( E )-2-[6-[2-[2-(2-[ 18 F]fluoranylethoxy)ethoxy]ethoxy]pyridin-3-yl]ethenyl]- N -methylaniline; Amyvid), [ 18 F]florbetaben (4-[( E )-2-[4-[2-[2-(2-[ 18 F]fluoranylethoxy)ethoxy]ethoxy]phenyl]ethenyl]- N -methylaniline; NeuraCeq), and [ 18 F]flutemetamol (2-[3-[ 18 F]fluoranyl-4-(methylamino)phenyl]-1,3-benzothiazol-6-ol; Vizamyl), and have proven efficacy in several clinical trials. The tracers can be used to estimate the Aβ plaque density in patients with suspected AD but are not indicated to diagnose AD or other neurodegenerative disorders based on imaging alone. These 3 tracers continue to undergo clinical development and are commonly used as a standard with which to compare novel tracers. For example, florbetaben is being examined, similarly to [ 11 C]C-PiB, as a means to quantify the comorbid Aβ plaque burden experienced by patients with other neurodegenerative diseases, such as PD.
There are 2 next-generation radiopharmaceuticals for imaging β-amyloid that have potential for clinical impact in the coming years: [ 18 F]FIBT (2-( p -methylaminophenyl)-7-(2-[ 18 F]fluoroethoxy)imidazo-[2,1-b]benzothiazole) and [ 18 F]NAV4694 (2-[2-[ 18 F]fluoro-6-(methylamino)-3-pyridinyl]-1-benzofuran-5-ol). In a human study involving 6 patients with AD, [ 18 F]FIBT showed imaging quality comparable with [ 11 C]PiB. [ 18 F]FIBT was also shown to have a higher binding affinity and specificity for Aβ plaques. This study followed the first human study for [ 18 F]FIBT in 2015, which involved 2 patients: 1 patient with AD and 1 control. In this preliminary study, [ 18 F]FIBT was able to successfully differentiate the patient with AD from the control and showed a strong pattern of tracer uptake consistent with AD. Because of the small number of subjects in these studies, larger clinical trials are required to further understand the utility of these agents.
The second radiopharmaceutical, [ 18 F]NAV4694 (also known as [ 18 F]AZD4694), has been shown to have imaging characteristics nearly identical to those of [ 11 C]PiB and has recently undergone phase 2 and phase 3 trials ( NCT01886820 , NCT01680588 ). Despite its demonstrated efficacy, a recently conducted human trial showed low uptake of the tracer in the preclinical phase of AD, suggesting a limit for its clinical use. However, this tracer remains promising for future clinical use because of its favorable imaging characteristics.
Tau imaging agents
Tau protein is most associated with cognitive decline in neurodegenerative diseases such as AD. Note that tau protein accumulation is not a specific biomarker for AD; the protein is also present during acute brain conditions such as stroke. In addition, different forms of tau accumulate in different neurodegenerative diseases, and PET tracers targeting tau typically bind preferentially to certain forms of tau. However, research has shown that measuring the buildup of tau, which can occur before the formation of Aβ plaques, may be important in detecting AD in early stages. Studies such as these suggest the important complementary role nonspecific protein imaging can play in disease-targeted imaging.
There are 3 radiopharmaceuticals that have been widely studied in the targeting of tau protein: [ 18 F]THK5351 ((2 S )-1-[ 18 F]fluoranyl-3-[2-[6-(methylamino)pyridin-3-yl]quinolin-6-yl]oxypropan-2-ol), [ 18 F]AV1451 (7-(6-fluoropyridine-3-yl)-5 H -pyrido[4,3-b]indole), [ 11 C]PBB3 (2-((1 E ,3 E )-4-(6-( 11 C-methylamino)pyridine-3-yl)buta-1,3-dienyl)benzo[d]thiazol-6-ol). In general, these 3 tracers were found to have excellent selectivity for tau protein compared with Aβ plaques with some off-target binding. For example, [ 18 F]THK5351 binds to monoamine oxidase B and [ 11 C]PBB3 has been shown to bind to α-synuclein. A study with [ 18 F]AV1451 involving 8 patients with AD and 8 healthy controls showed some age-related uptake of the compound among the healthy controls, indicating some off-target uptake.
Beyond these 3 compounds, a new generation of tau tracers were designed to reduce off-target binding. Among these new radiopharmaceuticals, [ 18 F]MK-6240 (6-[ 18 F]fluoranyl-3-pyrrolo[2,3-c]pyridin-1-ylisoquinolin-5-amine) and [ 18 F]RO-6958948 (2-(6-[ 18 F]fluoro-pyridin-3-yl)-9H-1,6,9-triaza-fluorene) have shown promising results. The safety and efficacy of [ 18 F]MK-6240 has been shown preclinically and is currently the subject of several active clinical trials ( NCT04104659 , NCT03706261 ). Large clinical trials have shown that [ 18 F]MK-6240 is able to identify individuals with AD with high fidelity and accuracy. The tracer has also been an important tool in developing a better understanding of the role the tau protein plays in AD progression.
A human trial comparing [ 11 C]PiB and [ 18 F]MK-6240 showed patients with increased Aβ burden experience cognitive decline largely accounted for by the level of tau protein. This trial highlights a potentially important role for future tau imaging to monitor disease progression. In addition, the short half-life of [ 11 C]PiB could allow the imaging of Aβ plaques and tau protein in the same patient on the same day ( Fig. 3 ).
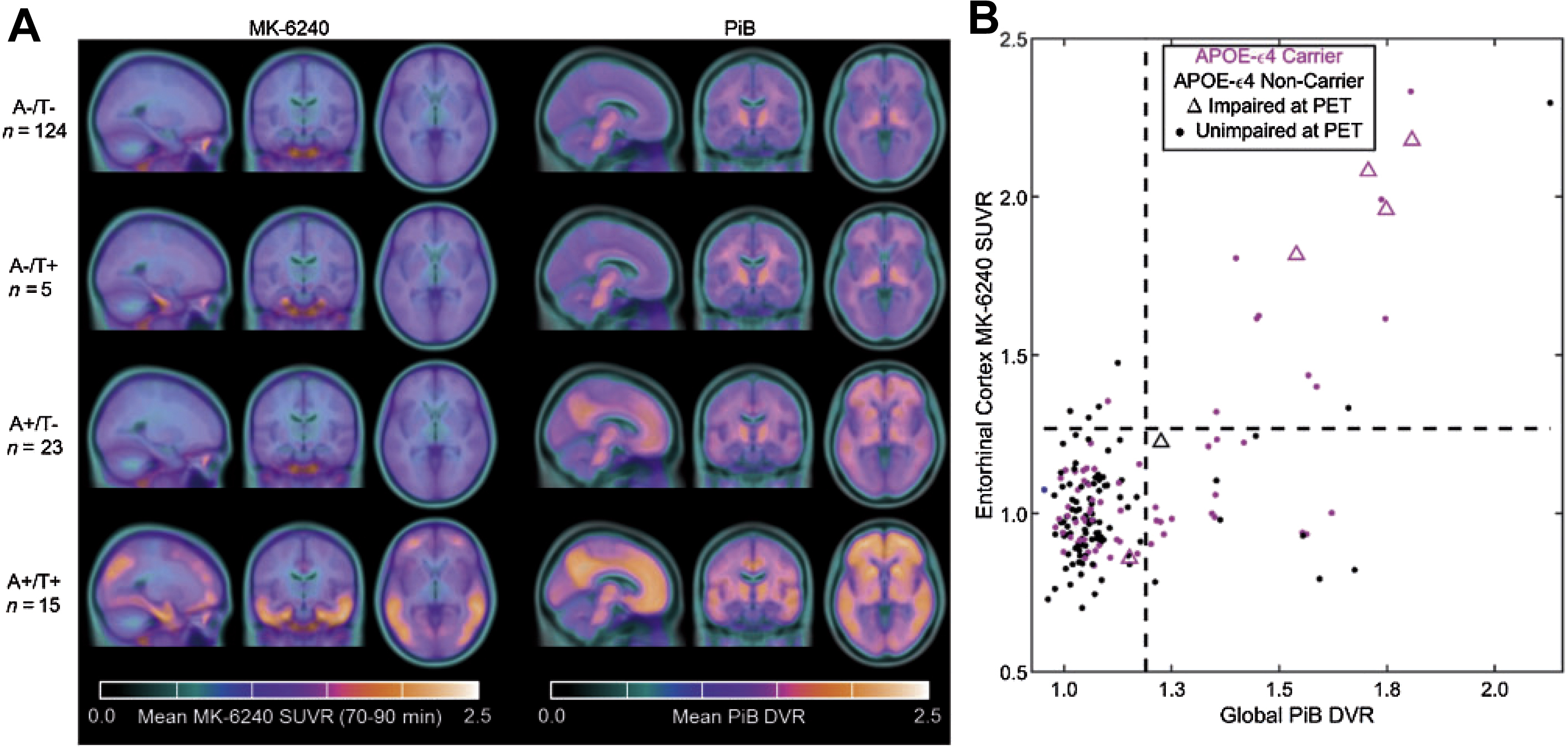
The tracer [ 18 F]RO-6958948 has undergone preclinical testing in mice, which showed its highly specific binding to tau and rapid kinetics. The tracer has also recently completed several phase 1 trials testing for safety and efficiency in humans ( NCT02792179 , NCT02187627 ). Future clinical trials showing its clinical relevance in targeting tau protein are expected in the future.
Neurotransmission-Targeted Imaging
Dopaminergic system imaging
Patients with PD are known to experience a neurodegeneration affecting the nigrostriatal system, one of the 4 dopaminergic pathways in the brain. A well-established tracer for dopamine metabolism is [ 18 F]FDOPA. This tracer is a substrate for aromatic acid decarboxylase (AADC), an enzyme necessary for the conversion of aromatic amino acids into neurotransmitters such as dopamine. This tracer was approved by the FDA in 2019 for the evaluation of adult patients with suspected parkinsonian syndromes, including PD, through the visualization dopaminergic nerve terminals. Human trials have shown that this tracer is both highly sensitive and highly specific.
The design of the next generation of radiopharmaceuticals in this area is primarily focused on targeting 2 additional proteins: dopamine transporter (DAT) and vesicular monoamine transporter 2 (VMAT2). There are 2 novel tracers of interest related to these protein targets: [ 18 F]FP-CIT (methyl(1 R ,2 S ,3 S ,5 S )-8-(3-fluoropropyl)-3-(4-[ 18 F]fluorophenyl)-8-azabicyclo[3.2.1]octane2-carboxylate) and [ 18 F]FE-PE2I (( E )- N -(3-iodoprop-2-enyl)-2β-carbo[ 18 F]fluoroethoxy-3β-(4′-methyl-phenyl)nortropane). In the case of FP-CIT, its 123 I-labeled form, ioflupane, is used clinically for single-photon emission computed tomography (SPECT) imaging in patients with suspected PD. An analysis of 6 PET clinical studies showed that [ 18 F]FP-CIT is a potential biomarker for early PD diagnosis. In addition, a study with 9 patients with early PD was conducted using [ 18 F]FE-PE2I, which was successful in differentiating healthy controls and patients with early PD. This same compound also showed good repeatability and reliability and is a possible marker to monitor the progress of DAT decline. There has also been interest in imaging vesicular acetylcholine transporter, which has been associated with PD, using the PET ligand (2 R ,3 R )-5-[ 18 F]fluoroethoxybenzovesamicol.
Inflammation-Targeted Imaging and Synaptic Density
There is considerable evidence that both chronic inflammation and reduced synaptic density are associated with neurodegenerative disorders. As a result, there is interest in identifying markers for these two conditions associated with neurodegeneration. Of particular interest for inflammation is the translocator protein (TSPO) that is upregulated with high density in many neurologic disorders. Markers of interest for synaptic density include high-density neural synapse proteins, one of which is synaptic vesicle protein 2A (SV2A). The loss or decrease in density of these synaptic proteins indicates disease progression.
Translocator protein imaging
TSPO is a transmembrane protein upregulated during periods of neuroinflammation, following the activation of microglial cells. One of the first radiopharmaceuticals targeting this protein was [ 11 C]PK-11195 ( N – sec -butyl-1-(2-chlorophenyl)- N -[ 11 C]methyl-3-isoquinolinecarboxamide), which was widely used in research for several decades. However, clinical use of this compound was limited by nonspecific binding, low brain uptake, and the short half-life of 11 C.
A second generation of TSPO-targeting radiopharmaceuticals was developed to address these limitations. Compounds in this second generation include [ 11 C]DAA1106 ( N -(5-fluoro-2-phenoxyphenyl)- N -[(5-methoxy-2-[ 11 C]methoxyphenyl)methyl]acetamide), [ 11 C]PBR28 ( N -[(2-[ 11 C]methoxyphenyl)methyl]- N -(6-phenoxypyridin-3-yl)acetamide), [ 18 F]FEPPA ( N -[[2-(2-[ 18 F]fluoranylethoxy)phenyl]methyl]- N -(4-phenoxypyridin-3-yl)acetamide), [ 18 F]PBR06 ( N -[(2,5-dimethoxyphenyl)methyl]-2-[ 18 F]fluoranyl- N -(2-phenoxyphenyl)acetamide), [ 18 F]PBR11 (2-(6-chloro-2-(4-(3-[ 18 F]fluoropropoxy)phenyl)imidazo[1,2-a]pyridin-3-yl)- N , N -diethylacetamide), and [ 18 F]DPA714 ([ N , N -diethyl-2-(2-(4-(2[ 18 F]fluoroethoxy)phenyl)5,7dimethylpyrazolo[1,5a]pyrimidin-3-yl)acetamide]). Many of these new compounds showed higher brain uptake and greater specificity for the TSPO target compared with [ 11 C]PK-11195. However, additional studies also revealed that genetic variation in the TSPO gene among participants resulted in TSPO expression with varied the binding affinity for the tracer, leading to the classification of so-called high-affinity, low-affinity, and mixed-affinity binding groups. Patients with low-affinity TSPO binding present a significant clinical contraindication for TSPO-targeted imaging and have been excluded from some clinical trials. Despite this limitation, the largest improvement in TSPO-targeted imaging with second-generation radiopharmaceuticals was the development of 18 F-labeled compounds, which benefit from a longer half-life compared with their 11 C-labeled counterparts to allow more widespread use.
TSPO-targeted imaging studies of neurodegenerative disorders with second-generation DPA-714 modeled acute inflammation in rats and showed that [ 18 F]DPA-714 is a selective and reliable biomarker with a more favorable signal/noise ratio than [ 11 C]PK-11195. However, one of the first human trials, involving 10 patients with AD and 6 healthy controls, showed no significant difference in [ 18 F]DPA-714 uptake between the two groups. Note that no information on the binding status of the participants of this trial was available for this study. A subsequent human trial, involving 64 patients with AD and 32 controls, specifically studied high-affinity and mixed-affinity binders. The results of the trial showed greater TSPO uptake among high-affinity and mixed-affinity binders compared with healthy controls and also showed that participants with a greater uptake of TSPO had a slower disease progression over a period of 2 years, indicating a possible protective effect provided by TSPO. The results of these 2 clinical trials introduce new questions regarding the use of [ 18 F]DPA-714 in identifying neurodegeneration and the potential importance of binding status on TSPO-targeted imaging. Further exploration of this compound continues, including current clinical trials to determine whether patients who experience higher neuroinflammation have more symptoms from neuroinflammatory diseases ( NCT03759522 ). Overall, the development of second-generation TSPO imaging agents has shown promise regarding binding specificity and brain uptake, but continued developments may improve image quality and diagnostic utility.
Synaptic vesicle protein 2A
SV2A is a membrane protein localized to the synapse in neural cells. Many neurodegenerative diseases are known to reduce the density of these synapses, thereby reducing the amount of SV2A. As a result, SV2A has been investigated as a clinical marker for neurodegeneration.
Among the first-generation SV2A tracers, 3 have been identified with potential clinical relevance: [ 18 F]UCB-H, [ 11 C]UCB-A, and [ 11 C]UCB-J (4 R )-1-(3-([ 11 C]methylpyridin-4-yl)methyl)-4-(3,4,5-trifluorophenyl)pyrrolidin-2-one. A preclinical analysis of these 3 compounds found [ 11 C]UCB-J to have superior imaging characteristics, including rapid brain uptake, and fast, reversible binding with high specificity to SV2A. Compared with [ 11 C]UCB-J, [ 11 C]UCB-A was found to have a slower kinetics, whereas [ 18 F]UCB-H had less specific binding.
To date, there have been several clinical trials that have shown the efficacy of these 3 compounds as a diagnostic measure in neurodegenerative disorders ( NCT03577262 , NCT04243304 ). The safety and efficacy of [ 18 F]UCB-H as a means of studying synaptic density was first shown in a small human study involving 4 healthy subjects. Similar efficacy was shown in a clinical trial of [ 11 C]UCB-J involving 10 patients with AD and 11 healthy controls, which found a significant reduction of SV2A in areas of the brain associated with the progression of AD disease. A larger clinical trial of [ 18 F]UCB-H involving 24 patients with AD and 19 healthy controls also showed a significantly reduced uptake of the tracer in areas of the brain related to cognitive decline in patients with AD.
Out of the 3 first-generation SV2A tracers, [ 11 C]UCB-J, is considered the best in its class because of its rapid uptake, fast kinetics, and specific binding. However, the short half-life of 11 C presents a limitation for widespread use, similar to the first-generation TSPO-targeting compound [ 11 C]PK-11195. Independent attempts to synthesize a longer-lived 18 F derivative were met with success by 2 research groups, who synthesized a compound that was jointly named [ 18 F]SynVesT-1 (4 R )-1-(3-([ 18 F]methylpyridin-4-yl)methyl)-4-(3,4,5-trifluorophenyl)pyrrolidin-2-one. In a preliminary study comparing the efficacy of [ 18 F]FSDM-8 and [ 11 C]UCB-J, the former was found to have imaging properties comparable with the first-generation compound with the added benefit of a longer half-life. Current clinical trials with this second generation of SV2A tracers are ongoing or recently completed ( NCT03587649 ).
Radiopharmaceuticals for cardiovascular PET imaging
Most cardiac PET imaging has focused on blood flow ( 82 Rb and [ 13 N]NH 3 ) and metabolism ([ 18 F]FDG). Research into newer agents for both of these areas continues and is complemented by additional compounds in imaging of atherosclerosis and angiogenesis.
PET Agents for Myocardial Perfusion Imaging
Although the approval of 82 Rb and [ 13 N]NH 3 has enabled widespread cardiac PET imaging, the very short half-lives of 82 Rb and 13 N make these studies accessible only at sites with access to an 82 Sr/ 82 Rb generator or an on-site cyclotron. Thus, there has been significant research into longer-lived imaging agents for this purpose.
[ 18 F]Flurpiridaz (2- tert -butyl-4-chloro-5-[4-(2-[ 18 F]fluoroethoxymethyl)-benzyloxy]-2H-pyridazin-3-1) is under investigation for myocardial perfusion imaging in late-stage clinical trials. A phase III clinical trial recently reported that, although the imaging study was not as specific as other available agents, the sensitivity was significantly higher than SPECT agents, as shown in Fig. 4 . Studies comparing imaging characteristics of [ 18 F]flurpiridaz with those of [ 13 N]NH 3 showed no significant differences between parameters derived from images with either agent. Other agents labeled with 18 F and 68 Ga are also under investigation in preclinical studies. ,
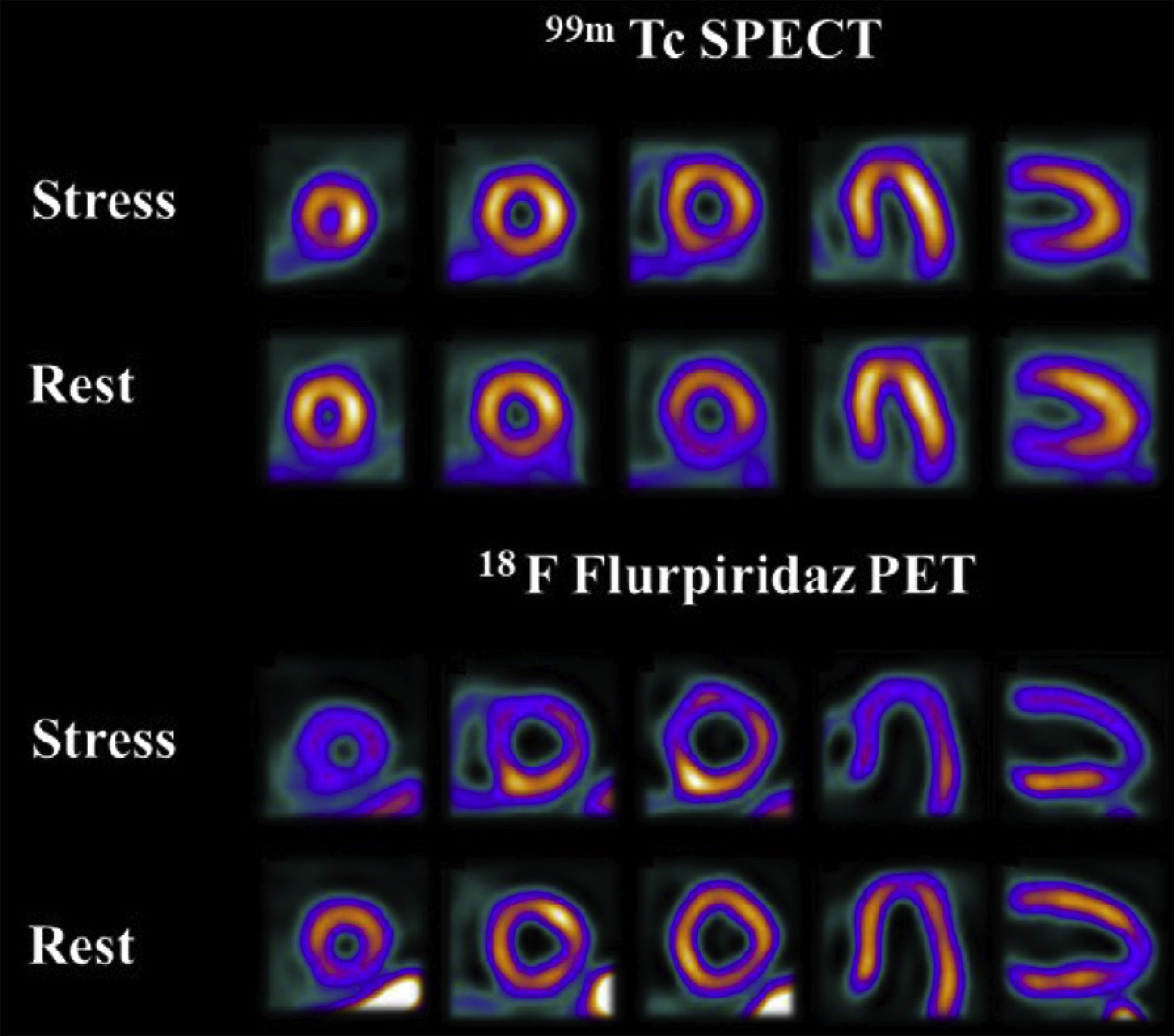
PET Agents for Imaging of Cardiac Metabolism
[ 18 F]FDG is widely available and has been used for cardiac metabolism and sarcoidosis. However, complementary agents have provided additional insight into cardiac function.
[ 11 C]Acetate has long been used for imaging of metabolism in a variety of disease states. In the research setting, this imaging strategy has been used to study cardiac metabolism in several investigations. For example, in a study of asymptomatic men with chromic alcohol consumption, investigators showed that [ 11 C]acetate PET/CT could be used to detect metabolic changes in the myocardium. Newer imaging analysis techniques combined with dynamic imaging have also shown the feasibility of oxygen consumption, myocardial external efficiency, and blood flow in a single scan. ,
Imaging of fatty acid metabolism has also been an ongoing area of study in cardiac research. In particular, [ 11 C]palmitate has been used for many years in basic metabolism studies. In a multiple tracer study involving [ 15 O]water and [1- 11 C]glucose, palmitate, and leucine, investigators were able to image myocardial metabolic changes associated with Barth syndrome. Newer fatty acid agents such as [ 18 F]fluoro-4-thiapalmitate (FTP) are also being studied in ongoing clinical trials in cardiac metabolism. In a paired study with lean controls and diabetic glycemically controlled volunteers, investigators found fatty acid oxidation was higher in the diabetic group and could be altered in both groups by the administration of insulin.
PET Agents for Imaging of Cardiovascular Disease
As mentioned earlier, there are several agents that have shown utility in assessment of amyloid burden in patients with cognitive impairment. Atherosclerosis has also been shown to contain amyloid proteins. Thus, imaging using previously developed agents for β-amyloid has been investigated in atherosclerosis and cardiac amyloidosis. Other biomarkers, including CCR2, are also currently being studied.
Amyloid imaging
Preliminary human imaging studies using [ 18 F]flutemetamol showed visible uptake in carotid arteries, with male gender associated with enhanced uptake. In a recent study in patients with suspected cardiac amyloidosis, imaging with [ 18 F]florbetaben at delayed timepoints allowed the differentiation of immunoglobulin light chain–derived amyloidosis from transthyretin-related amyloidosis. Similar results were also reported using [ 11 C]PiB in multiple studies. , However, in several recent [ 18 F]flutemetamol studies in patients with cardiac amyloidosis, results were mixed, with 1 group reporting that PET/CT could be used to differentiate between patients with transthyretin amyloidosis and another reporting that the diagnostic yield from images acquired was low. , Additional clinical trials in this area are ongoing ( NCT01683825 , NCT04105634 , NCT02641145 , NCT04392960 ).
Other targets in atherosclerosis
Macrophages and other inflammatory cells are known to be associated with atherosclerotic processes. Existing agents targeting TSPO and somatostatin receptors have been investigated for imaging of inflammatory processes with some success in preclinical models and several clinical trials. For example, in a study involving 42 patients with atherosclerosis [ 68 Ga]DOTATATE outperformed [ 18 F]FDG for the evaluation of high-risk versus low-risk coronary lesions ( NCT02021188 ). Newer agents such as [ 64 Cu]DOTA-ECL1i, which targets CCR2, are currently in clinical trials ( NCT04537403 ) in patients with carotid and femoral artery disease.
Prominent and novel tracers, along with their current status, are listed in Table 1 .
Abbreviation | Chemical Name |
---|---|
FAPI | Fibroblast activation protein inhibitor |
FLT | 3′-Fluoro-3′ deoxythymidine |
FDG | Fluorodeoxyglucose |
PSMA | Prostate-specific membrane antigen |
[ 18 F]DCFPyL | 2-(3-{1-carboxy-5-[(6-[ 18 F]fluoro-pyridine-3-carbonyl)-amino]-pentyl}-ureido)-pentanedioic acid |
DFO | Deferoxamine |
DLL3 | Deltalike protein 3 |
PARP1 | Poly(ADP-ribose) polymerase 1 |
[ 18 F]F-AraG | [ 18 F]fluoro-9-(β- d -arabinofuranosyl) guanine |
pHLIP | pH low insertion peptide |
[ 18 F]FMISO | [ 18 F]fluoro-misonidazole |
[ 18 F]FAZA | [ 18 F]fluoroazomycin arabinoside |
[ 64 Cu]Cu-ATSM | [ 64 Cu]Cu-diacetyl-bis(N -methylthiosemicarbazone) |
[ 11 C]MET | L-[ 11 C]methionine |
[ 18 F]FET | O -(2-[ 18 F]fluoroethyl)- l -tyrosine |
[ 18 F]FSPG | (4 S )-4-(3[ 18 F]fluoropropyl)- l -glutamic acid |
[ 18 F]FES | 16α-[ 18 F]fluoroestradiol |
[ 18 F]FFNP | 21-[ 18 F]F-fluoro-furanyl-norprogesterone |
[ 18 F]FDHT | 16β-[ 18 F]F-fluoro-5α-dihydrotestosterone |
[ 11 C]PiB | 2-[4-([ 11 C]methylamino)phenyl]-1,3-benzothiazol-6-ol |
[ 18 F]Florbetapir | 4-[( E )-2-[6-[2-[2-(2-[ 18 F]fluoranylethoxy)ethoxy]ethoxy]pyridin-3-yl]ethenyl]- N -methylaniline |
[ 18 F]Florbetaben | 4-[( E )-2-[4-[2-[2-(2-[ 18 F]fluoranylethoxy)ethoxy]ethoxy]phenyl]ethenyl]- N -methylaniline |
[ 18 F]Flutemetamol | 2-[3-[ 18 F]fluoranyl-4-(methylamino)phenyl]-1,3-benzothiazol-6-ol |
[ 18 F]FIBT | 2-( p -Methylaminophenyl)-7-(2-[ 18 F]fluoroethoxy)imidazo-[2,1-b]benzothiazole |
[ 18 F]NAV4694 | 2-[2- 18 F-fluoro-6-(methylamino)-3-pyridinyl]-1-benzofuran-5-ol |
[ 18 F]THK5351 | (2 S )-1-[ 18 F]fluoranyl-3-[2-[6-(methylamino)pyridin-3-yl]quinolin-6-yl]oxypropan-2-ol |
7-(6-fluoropyridine-3-yl)-5H-pyrido[4,3-b]indole | |
[ 11 C]PBB3 | 2-((1 E ,3 E )-4-(6-( 11 C-methylamino)pyridine-3-yl)buta-1,3-dienyl)benzo[d]thiazol-6-ol |
[ 18 F]MK-6240 | 6-[ 18 F]fluoranyl-3-pyrrolo[2,3-c]pyridin-1-ylisoquinolin-5-amine |
[ 18 F]Ro-6958948 | 2-(6-[ 18 F]fluoro-pyridin-3-yl)-9H-1,6,9-triaza-fluorene |
[ 18 F]FDOPA | (2 S )-2-amino-3-(2-[ 18 F]fluoro-4,5-dihydroxyphenyl)propanoic acid |
[ 18 F]FP-CIT | Methyl(1 R ,2 S ,3 S ,5 S )-8-(3-fluoropropyl)-3-(4-[ 18 F]fluorophenyl)-8-azabicyclo[3.2.1]octane2-carboxylate |
[ 18 F]FE-PE2I | ( E )-N-(3-iodoprop-2-enyl)-2β-carbo[ 18 F]fluoroethoxy-3β-(4′-methyl-phenyl)nortropane). |
[ 18 F]FEOBV | (2 R ,3 R )-5-[ 18 F]fluoroethoxybenzovesamicol |
[ 11 C]CPK-11195 | N – sec -Butyl-1-(2-chlorophenyl)- N -[ 11 C]methyl-3-isoquinolinecarboxamide |
[ 11 C]DAA1106 | N -(5-Fluoro-2-phenoxyphenyl)-N-[(5-methoxy-2-[ 11 C]methoxyphenyl)methyl]acetamide |
[ 11 C]PBR28 | N -[(2-[ 11 C]methoxyphenyl)methyl]- N -(6-phenoxypyridin-3-yl)acetamide |
[ 18 F]FEPPA | N -[[2-(2-[ 18 F]fluoranylethoxy)phenyl]methyl]- N -(4-phenoxypyridin-3-yl)acetamide |
[ 18 F]PBR06 | N -[(2,5-Dimethoxyphenyl)methyl]-2-[ 18 F]fluoranyl- N -(2-phenoxyphenyl)acetamide |
[ 18 F]PBR11 | 2-(6-Chloro-2-(4-(3-[ 18 F]fluoropropoxy)phenyl)imidazo[1,2-a]pyridin-3-yl)- N , N -diethylacetamide |
[ 18 F]DPA714 | [N,N-diethyl-2-(2-(4-(2[18F]fluoroethoxy)phenyl)5,7dimethylpyrazolo[1,5a]pyrimidin-3-yl)acetamide] |
[ 11 C]UCB-J | (4R)-1-((3-([11C]methylpyridin-4-yl)methyl)-4-(3,4,5-trifluorophenyl)pyrrolidin-2-one |
[ 18 F]SynVesT-1 | (4R)-1-((3-([18F]methylpyridin-4-yl)methyl)-4-(3,4,5-trifluorophenyl)pyrrolidin-2-one |
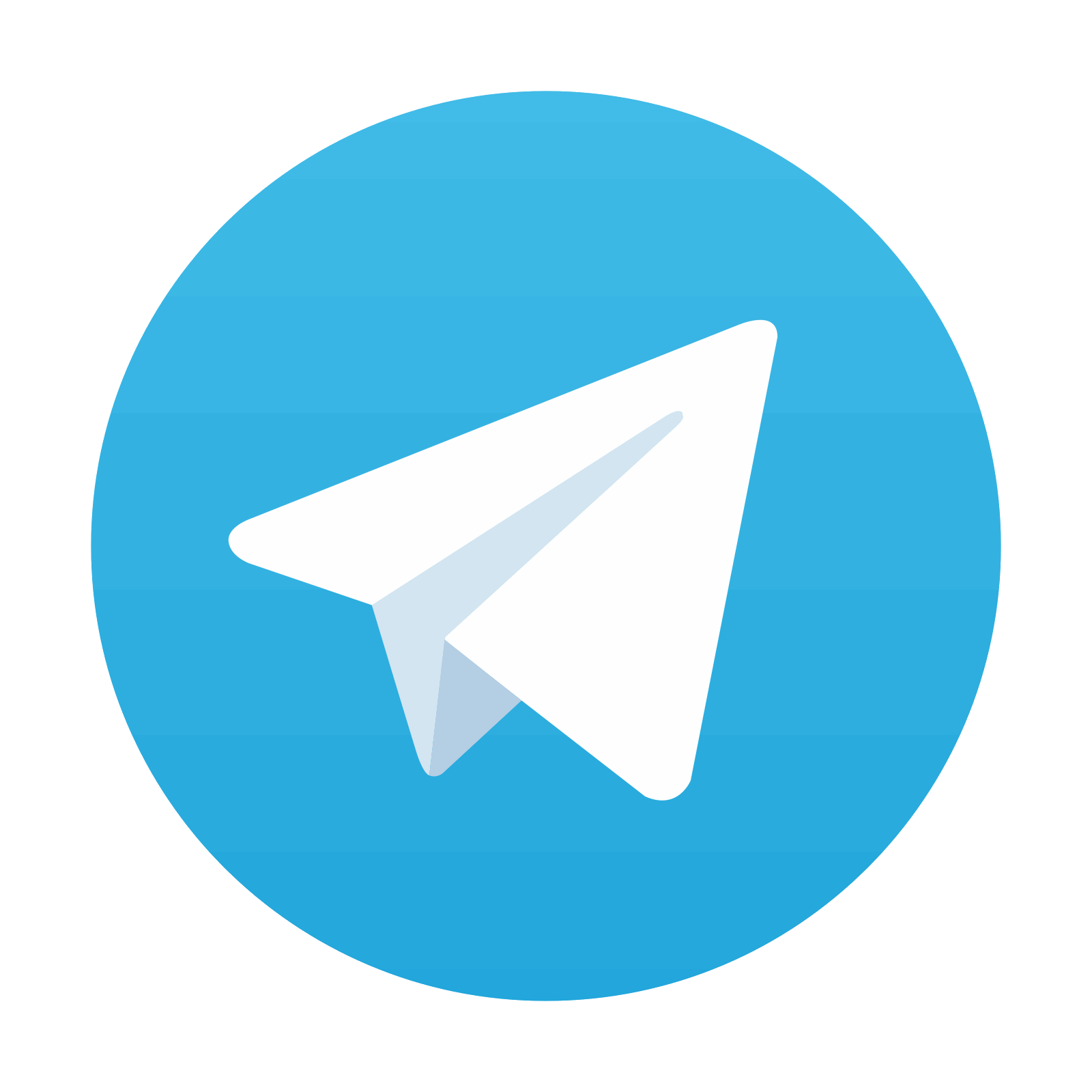
Stay updated, free articles. Join our Telegram channel

Full access? Get Clinical Tree
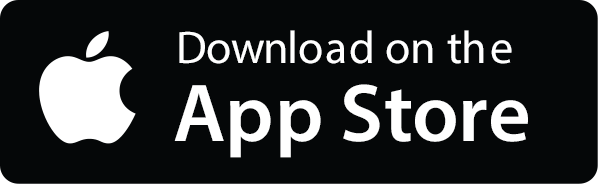
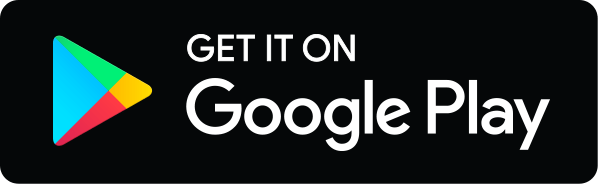
