© Springer-Verlag Berlin Heidelberg 2015
Andor W.J.M. Glaudemans, Rudi A.J.O. Dierckx, Jan L.M.A. Gielen and Johannes (Hans) Zwerver (eds.)Nuclear Medicine and Radiologic Imaging in Sports Injuries10.1007/978-3-662-46491-5_99. Nuclear Medicine Imaging of Head and Face Injuries
(1)
Department of Radiology and Nuclear Medicine, Martini Hospital Groningen, Van Swietenplein 1, Groningen, 9728 NT, The Netherlands
Abstract
Head injuries, which form approximately 10 % of all sports-related trauma in high school sports activities, can have devastating effects on the individual and society.
Nuclear medicine has thus far not been playing an important role in trauma to the bony structures of the head, but this might change due to the higher resolution and sensitivity of 18F-NaF PET for ossal pathology. Especially in the follow-up, 18F-NaF may become more important, but research is warranted.
Concussion is mainly a clinical diagnosis, and imaging techniques do not play an important role. However, new techniques in both cerebral blood flow measurements with SPECT and cerebral metabolism with PET are emerging. These techniques not only give a better insight in the underlying pathological mechanisms of concussion but can also aid in the diagnosis and follow-up of patients suspected of concussion. Actually, the position of nuclear medicine techniques in the diagnosis of concussion has not been determined, so more research is warranted.
9.1 Introduction
Participating in sports has many advantages, since it can increase one’s strength, endurance, and flexibility. Furthermore, it has a beneficial effect on weight management and on self-esteem (Darrow et al. 2009). In the USA, approximately 50 % of all children participate in organized sports programs (Adirim and Cheng 2003). However, the downside of the participation in sports is the potential risks caused by sports trauma. Generally, patients with sports-related injuries tend to be younger than the average population (Bensch et al. 2011). Children seem to be more vulnerable to sports-related injuries due to both physical and psychological factors (Adirim and Cheng 2003). It has been calculated that in young athletes, approximately 10 % of all high school sports-related trauma was inflicted to the head (Rechel et al. 2011). Conversely, in 3–25 % of all patients, head and neck trauma was caused by accidents occurring during sports activities (Kraus 1991). The majority of these injuries consist of fractures of maxillofacial structures and brain concussions. Moreover, the incidence of head and neck injuries is related to the violence and aggression related to the type of sport practiced (Proctor and Cantu 2000).
The potential consequences, both economical and personal, of potentially disabling injuries to the head have a very high impact on the individual and the society (Bensch et al. 2011). Since concussions and repeated concussions may have long-term neuropsychological effects, correct understanding, diagnosing, and imaging are essential (Broglio et al. 2011; Marshall 2012; Su and Ramirez 2012).
9.2 Bone Injuries to the Skull in Sports Medicine
In contrary to the other skeletal structures, athletes almost never sustain stress fractures of the skull due to sports-related activities. The most common sports-related traumata to the skull are caused by blunt trauma in an acute setting. In patients with suspected fractures of the skull and maxillofacial structures, it is essential to perform radiological imaging to assess the extent of the damage and evaluate treatment options (Darrow et al. 2009; Kaur and Chopra 2010; Zemper 2010). In most cases, nuclear medicine has no role for the diagnosis of fractures of the skull and maxillofacial structures. However, when radiological imaging shows no abnormalities, but fractures are still suspected based on trauma mechanism and patient complaints, bone scintigraphy and 18F sodium fluoride (18F-NaF) PET scanning may be used to rule out fractures since these techniques are able to detect minimal changes in osteoblastic activity, before radiographic changes become evident (Even-Sapir et al. 2007; Zukotynski et al. 2010).
In the follow-up period after the diagnosis of maxillofacial and skull trauma, nuclear medicine may have added value, especially to assess the fracture healing. Only few studies have thus far been published on fracture healing in the skull. Bone scintigraphy with SPECT has been proven useful for the follow-up of complex midfacial fractures, in particular for the diagnosis of complications after initial repositioning of the midfacial ossal structures (Top et al. 2004). PET/CT scanning with 18F-NaF PET could theoretically be more useful in the follow-up period, since it has a higher resolution compared to SPECT. 18F-NaF uptake corresponds to osteoblastic activity and also for accompanying reactive osteoblastic changes, even when minimal (Even-Sapir et al. 2007). For example, in studies concerning child abuse, 18F-NaF PET was shown to have a potential advantage over 99mTc-labeled methylene diphosphonate (MDP) based upon superior image contrast and spatial resolution (Drubach et al. 2008, 2010; Grant et al. 2008).
The process of fracture healing can be evaluated using plain radiography, CT, ultrasonography, fluoroscopy, bone scan, and MRI (Bishop et al. 2012). Although the role these different imaging techniques have in the diagnostic process of evaluating fracture healing is not yet clear, 18F-NaF PET could become an important tool for this purpose based on animal experiments. In a study in rats, 18F-NaF PET was able to identify fracture nonunions already at early time points and may therefore have a major role in the assessment of fracture healing (Hsu et al. 2007).
9.2.1 Contusions of the Maxillofacial Ossal Structures
Bone bruises arise together with soft tissue injuries after physical trauma and are usually presented as bleedings, edemas, or infarctions. Bone bruises are characterized by micro-trabecular fractures which leave the bone itself macroscopically intact (Rangger et al. 1998). MRI has, already early after recognition of this disease entity, an important role in diagnosis of bone bruises since it provides the clinician with a clear insight into the extent and severity of the bone lesion (Ucar et al. 2012). Historically, nuclear medicine has not played an important role in the detection of bone bruises. Bone scintigraphy is able to detect bone lesions but with a lower sensitivity and specificity than MRI (Soudry and Mannting 1995; Even-Sapir et al. 2002). In bone scintigraphy, bone bruises are characterized by focal increased osseous uptake. The intensity of uptake might relate to the extent of microfractures, i.e., more microfractures lead to an increased uptake. In combination with SPECT/CT or with 18F-NaF PET, bone scintigraphy may possibly be used as a total body screening method for bone bruises.
9.3 Concussion
Concussion is defined as “a traumatically induced transient disturbance of brain function” and involves a complex pathophysiological process. Concussion is a subset of mild traumatic brain injury (MTBI) which is generally self-limited and at the less severe end of the brain injury spectrum (Harmon et al. 2013; McCrory et al. 2013). In the United States, each year, an estimated 1.6–3.8 million sports-related concussions occur (Langlois et al. 2006). These brain injuries may lead to immediate (headaches, dizziness) problems and to long-term changes (i.e., behavioral changes, problems with attention) (Iverson et al. 2004). Imaging is essential in gaining insight in the extent of the occurring changes in concussion.
Concussion results from rotational or angular brain accelerations. These accelerations result in shear strains on the soft tissues of the brain, which results in disruption of membrane potentials, followed by an efflux of potassium into the extracellular space. Glutamate and other excitatory amino acids are then released, leading to a further depolarization of neuronal cells. This in turn leads to a widespread potential depression in the brain. To restore homeostasis, energy is needed in the form of ATP. ATP is formed by an increased glycolysis, which in turn leads to intracellular lactate accumulation (Bigler and Maxwell 2012; Lin et al. 2012). These metabolic changes occur before morphologic changes can be seen on CT or MRI. Therefore, early after sustaining a concussion, CT and MRI often show a normal brain structure. To evaluate the underlying metabolic changes, MRI spectroscopy and nuclear medicine techniques can be used. MRI and functional MRI can be used to show activation patterns which can be related to extend and severity of concussion, but these techniques have been described in Chap. 8 (Harmon et al. 2013; Virji-Babul et al. 2013). Nuclear medicine techniques (SPECT and PET) for evaluating concussion have mainly been used in a clinical setting. Generally, in concussion, nuclear medicine imaging is to this time point restricted to athletes who sustained serious injuries and in whom possible structural anomalies are expected.
9.3.1 SPECT
The metabolic changes occurring after brain injury have lead to the assumption that cerebral blood flow changes must take place. To evaluate this, several tracers for SPECT imaging are readily available. The most commonly used tracer is 99mTc-hexamethylpropyleneamine oxime (99mTc-HMPAO) (Lin et al. 2012). 99mTc-HMPAO crosses the blood-brain-barrier easily and is distributed in the brain proportional to the regional blood flow. The rapid brain uptake and prolonged retention of 99mTc-HMPAO in brain structures makes this tracer very suitable for evaluation of regional blood flow changes in the brain (Leonard et al. 1986). Detectable concentrations can be measured for up to 24 h after injection. Image analysis requires selecting different regions of interest (ROI) within the brain, and comparing these with a reference region, preferably the cerebellum, to determine the existence of regions with abnormal blood flow (Abdel-Dayem et al. 1998; Lin et al. 2012).
Other tracers which have been used for the evaluation of contusion are, for example, 99mTc -ethyl cysteinate dimer (99mTc-ECD) (Catafau 2001), 123I-iomazenil (123I-IMZ), 123I-N-isopropyl-p-iodoaphetamine (123I-IMP) (Leonard et al. 1986; Hashimoto and Abo 2009), and 57cobalt-chloride (57CoCl2) (Audenaert et al. 2003). However, 99mTc-HMPAO SPECT has been used most extensively.
Studies have been performed within 24 h after injury (acute phase), within 72 h (subacute phase) and in patients with chronic concussions (Lin et al. 2012). However, studies relating to sports-induced concussions are rare.
Only one case report described regional blood flow changes in the acute phase of concussion. In this case study, 99mTc-HMPAO was used to assess regional blood flow changes in a patient with a concussion after a motor vehicle accident. Within 24 h 99mTc-HMPAO SPECT scan was performed, showing hypoperfusion of the frontal and bilateral parietal lobes (Abu-Judeh et al. 1998).
SPECT studies for changes in regional blood flow in the subacute (<72 h) phase of concussion has been performed in larger patient groups. In a study using 99mTc-ECD SPECT in a patient group of 92 patients (28 adults and 64 children), the most commonly found perfusion abnormality was hypoperfusion in the frontal lobe(s) in adults and the temporal lobes in children. Furthermore, in patients with posttraumatic amnesia, postconcussion syndrome, and loss of consciousness, a significantly higher number of perfusion abnormalities were seen than in patients without these symptoms. Compared to CT, SPECT had a higher sensitivity for detecting an organic basis in patients with posttraumatic amnesia, postconcussion syndrome, and loss of consciousness (Gowda et al. 2006). Another study compared 99mTc-HMPAO SPECT with 57CoCl2 SPECT in 8 patients. 57CoCl2 was used as a marker for calcium accumulation. This study found a similar pattern of hypoperfusion in the frontal lobes for 99mTc-HMPAO SPECT with additional accumulation of 57CoCl2 in these areas, but also additional accumulation of 57CoCl2 was found in the parietal lobes. This may indicate that additional brain regions show dysfunctioning, which are not detected by blood flow changes only (Audenaert et al. 2003).
Two studies have been performed by Amen et al. (2011a, b) in which the effects of repetitive sports-induced head injury were evaluated with SPECT. In the first study, a group of 100 retired NFL players was evaluated in the chronic stage of their head injury. A significant decrease in regional cerebral blood flow was found in the whole brain, especially in the prefrontal lobes, temporal lobes, occipital lobes, anterior cingulate gyrus, and cerebellum. Furthermore, they found significant decreases in the posterior cingulate gyrus and hippocampus, areas which are also involved in dementia. The second group consisted of 30 retired NFL players with brain damage and cognitive impairment. Rehabilitation methods included weight loss (if appropriate), fish oil, a high-potency multiple vitamin, and a brain enhancement supplement that included nutrients to enhance blood flow, acetylcholine, and antioxidant activity. They were evaluated before and after six months of rehabilitation methods. After therapy, an increased perfusion in the prefrontal cortex, anterior cingulate gyrus, parietal and occipital lobes, and cerebellum was found, thereby demonstrating that cognitive and cerebral blood flow improvements are possible in this group with multiple interventions. Unfortunately, this study was not randomized, all patients received all interventional methods, and SPECT results were taken all together. Therefore, no conclusions can be made whether this was a real improvement or a medication effect on blood flow.
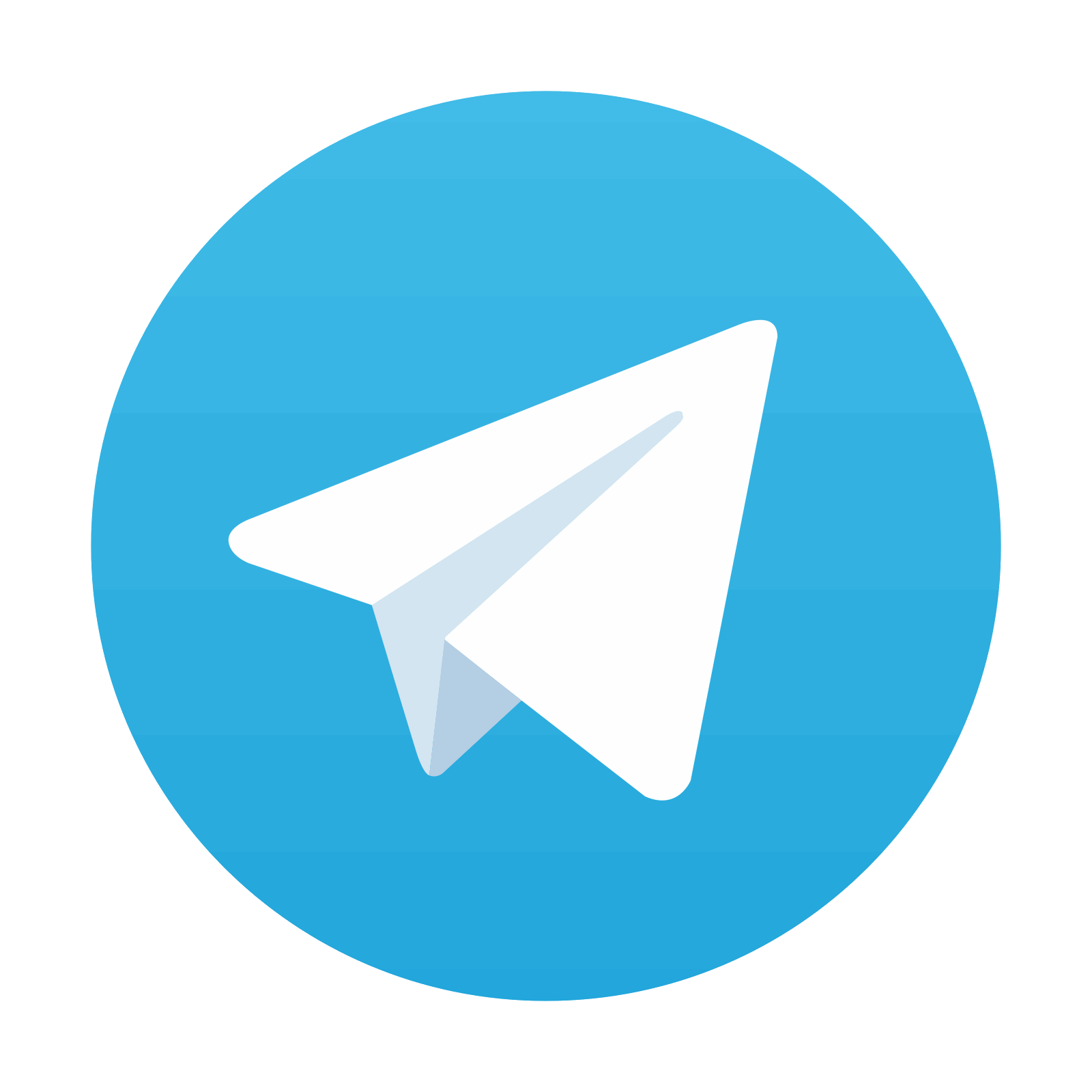
Stay updated, free articles. Join our Telegram channel

Full access? Get Clinical Tree
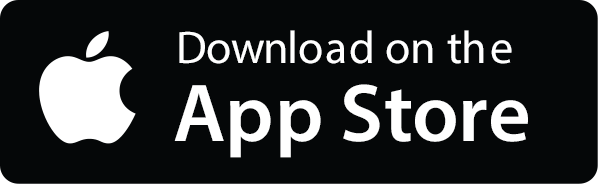
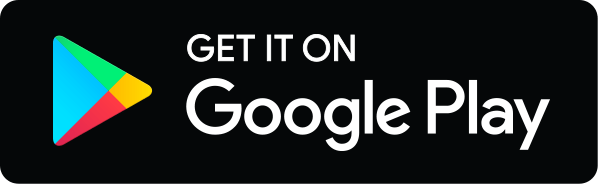