Optical coherence tomography is an imaging technique using low coherence light sources to produce high-resolution cross-sectional images. This article reviews pertinent anatomy and various pathologies causing optic atrophy (eg, compressive, infiltrating, demyelinating) versus optic nerve swelling (from increased intracranial pressure known as papilledema or other optic nerve intrinsic pathologies). On optical coherence tomography, optic atrophy is often associated with reduced average retinal nerve fiber layer thickness, whereas optic nerve swelling is usually associated with increased average retinal nerve fiber layer thickness.
Key points
- •
Optical Coherence Tomography (OCT) has revolutionized imaging of the retina and optic nerve and has enhanced our ability to diagnose and manage patients.
- •
OCT of the retina simulates to some degree an anatomic replica of a histological section of the retina treatment of macular degeneration, diabetic macular edema amongst other pathologies.
- •
OCT of the retina quantitates the thickness of the retinal nerve fiber layer (average retinal nerve fiber analysis), which is adjacent to the optic nerve (known as the circumpapillary retinal nerve fiber layer), and this is an indirect quantitative analysis of one’s complement of optic nerve fibers.
- •
The OCT average retinal nerve fiber analysis is very helpful in following optic nerve pathologies, for example glaucoma, MS, compressive optic neuropathies, however this technique does not distinguish between swollen and normal nerves, therefore, one may be given a false sense that the nerve is normal, when it fact it is swollen, thus missing that there is a reduced complement of retinal nerve fibers.
- •
The OCT average retinal nerve fiber analysis is a helpful technique in monitoring disc edema over time.
- •
OCT of the optic nerve gives a morphological view of the optic nerve as well as certain measurements that are helpful to follow over time.
Introduction
The eye is unique in that through its optics and utilization of devices that use these optics (eg, ophthalmoscope, retinal cameras, optical coherence tomography [OCT]), the neural and vascular tissues of the retina and optic nerve can be assessed by using noninvasive methods, making it ideal for detecting early changes associated with neural diseases. Although most information necessary for diagnosis can be obtained using ophthalmoscopy, imaging technologies with improved transverse and axial resolution are available to aid in the quantification of the morphology of the posterior pole (optic nerve and macula of the retina) yielding information that often is not appreciated solely with ophthalmoscopy. OCT offers good transverse and excellent axial resolution that has revolutionized eye care ( Fig. 1 ).
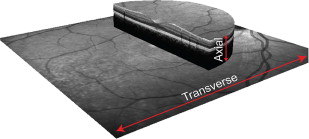
The basic principles of OCT technology can be compared with those of ultrasonography, in which the backscatter is used to generate images. In the late 1980s, Fujimoto and colleagues were the pioneers in using light energy to image biological tissues, using femtosecond pulsed lasers to image the cornea with 15 μm resolution. With modification and the use of superluminescent diodes, the technology was then applied to in vivo axial length measures (A-scans) with accuracy of 0.03 mm. However, it was not until 1991 that the first B-scans of human tissue were published using OCT technology. As with ultrasound technology, B-scans are composed of multiple aligned abutting A-scans, used to generate images that now assume a 2-dimensional form similar to the part of the eye imaged.
The first-generation clinical OCT instruments were time domain systems, with scan speeds of up to 400 Hz (A-scans per second) and axial resolution between 7 and 10 μm. However, with advances in technology, most clinical instruments are now spectral/Fourier domain with scan speeds greater than 25,000 Hz and axial resolutions up to 4 μm. The main difference between time domain and spectral domain (SD) systems is the use of a stationary reference arm and spectrometer system that results in faster, higher resolution images with the latter. Using multimodal imaging eye tracking is also available that aids in minimizing eye movement artifact and allows for accurate follow-up scan registration. Overall, with these scan speeds, 3-dimensional volume data of the anterior segment, optic nerve head, and macula regions ( Fig. 2 ) provide important information on the health of the eye. This article describes the current use of posterior segment imaging by OCT technology for the diagnosis and management of neuro-ophthalmic conditions.
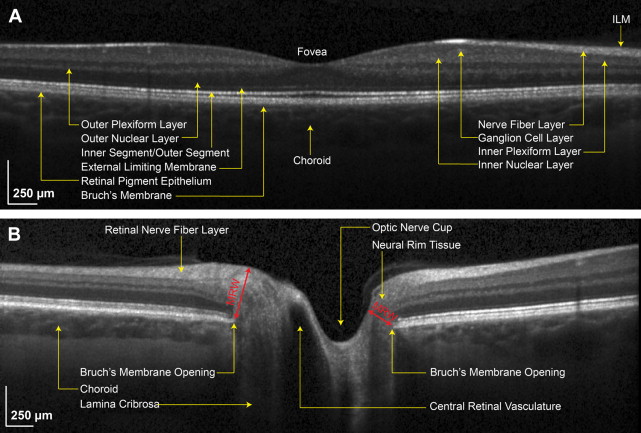
Introduction
The eye is unique in that through its optics and utilization of devices that use these optics (eg, ophthalmoscope, retinal cameras, optical coherence tomography [OCT]), the neural and vascular tissues of the retina and optic nerve can be assessed by using noninvasive methods, making it ideal for detecting early changes associated with neural diseases. Although most information necessary for diagnosis can be obtained using ophthalmoscopy, imaging technologies with improved transverse and axial resolution are available to aid in the quantification of the morphology of the posterior pole (optic nerve and macula of the retina) yielding information that often is not appreciated solely with ophthalmoscopy. OCT offers good transverse and excellent axial resolution that has revolutionized eye care ( Fig. 1 ).
The basic principles of OCT technology can be compared with those of ultrasonography, in which the backscatter is used to generate images. In the late 1980s, Fujimoto and colleagues were the pioneers in using light energy to image biological tissues, using femtosecond pulsed lasers to image the cornea with 15 μm resolution. With modification and the use of superluminescent diodes, the technology was then applied to in vivo axial length measures (A-scans) with accuracy of 0.03 mm. However, it was not until 1991 that the first B-scans of human tissue were published using OCT technology. As with ultrasound technology, B-scans are composed of multiple aligned abutting A-scans, used to generate images that now assume a 2-dimensional form similar to the part of the eye imaged.
The first-generation clinical OCT instruments were time domain systems, with scan speeds of up to 400 Hz (A-scans per second) and axial resolution between 7 and 10 μm. However, with advances in technology, most clinical instruments are now spectral/Fourier domain with scan speeds greater than 25,000 Hz and axial resolutions up to 4 μm. The main difference between time domain and spectral domain (SD) systems is the use of a stationary reference arm and spectrometer system that results in faster, higher resolution images with the latter. Using multimodal imaging eye tracking is also available that aids in minimizing eye movement artifact and allows for accurate follow-up scan registration. Overall, with these scan speeds, 3-dimensional volume data of the anterior segment, optic nerve head, and macula regions ( Fig. 2 ) provide important information on the health of the eye. This article describes the current use of posterior segment imaging by OCT technology for the diagnosis and management of neuro-ophthalmic conditions.
Normal anatomy through the macula and optic nerve with optical coherence tomography
Most commonly OCT is used to image the posterior pole. The posterior pole includes the macula (which subserves 8° of central vision) and the optic nerve. One may choose between 2 types of OCT scans: (1) retinal OCT and (2) optic nerve OCT.
- 1.
The retinal OCT typically can be centered on 2 areas: (a) the fovea and (b) the optic nerve with scans of the peripapillary retina.
- a.
The fovea (see Fig. 2 A; Fig. 3 A, B).When the retinal OCT is centered on the fovea, it yields a cross-section of the fovea (thinnest point of the retina that has the best central vision and accounts for 20/20 or better vision) and the surrounding retina with a typical scan width of 20°. One concentrates on all the retinal layers in this mode (see Fig. 2 A).
Fig. 3
( A ) Multiple horizontal lines through the foveal region (raster scan), all can produce individual B-scans per raster as shown in ( B ), which is a horizontal raster directly though the center of the fovea ( red line in 3A). ( C ) Multiple radial lines through the optic nerve show the morphology of the nerve at each radial line. ( D ) A radial line horizontally through the optic nerve shows the morphology through that particular section of the optic nerve. Double asterisk represents the optic cup depression. ( E ) The red circle indicates the area in which a 360° cross-section is taken at the retina adjacent to the optic nerve (peripapillary retina). See Fig. 6 A–C to see how this protocol translates into the retinal nerve fiber protocol. ( F ) The image shows the cross-section of peripapillary retina to yield a 360° picture portrayed in a linear fashion, with the far left and far right being the temporal aspect.
The signal strength along each OCT A-scan depends on tissue reflectivity and the corresponding interference pattern from that location. Resulting B-scans from this technology have a layered appearance representing that of retinal layers. However, the elements of each retinal layer are not homogenous and are represented as intensity changes in OCT scans. Hence, there are additional intensity bands of morphologic information in OCT scans. For accurate correspondence to histology, several studies in various species have reported using both time domain and SD OCT systems. In general, there is good agreement between these studies with similar identification of retinal and optic nerve head features.
- b.
The optic nerve with scans of the peripapillary retina (see Figs. 3 E, F and 6 ). The most common scan protocol used to evaluate the health of the optic nerve is a 12° diameter circumpapillary scan that is used to quantify the retinal nerve fiber layer thickness.
- a.
- 2.
The optic nerve OCT (see Figs. 2 B and 3 C, D) is centered to take cross-sections of the optic nerve head. The cross-sectional scan shows the morphology of the optic nerve (flat, elevated, or recessed) and the optic cup (see Fig. 3 D; double asterisk).
Fig. 3 is a comparison of the various protocols used to image the macula (retina) and optic nerve.
Qualitative versus quantitative analysis of macula and optic nerve
Qualitative and quantitative analyses of OCT provide useful information in the clinical setting for accurate diagnosis and management of patients. Qualitative scans (see Figs. 2 and 3 ; Fig. 4 ) provide visualization of the anatomy without distinct numbers or thickness. Quantitative scans provide measurements that make distinction between normal findings and diseases and assist in determining if there are any changes over time.
Qualitative scans
Most OCT devices allow for custom scan capture of single B-scans that can be positioned in the regions of interest with specified lengths and tilt. For example, a single line scan through the foveal pit (see Fig. 2 ), with averaging, is often used to determine inner versus outer retinal conditions. In addition, horizontal and vertical scans through the center of the optic nerve head are shown to be beneficial in determining optic nerve head and circumpapillary region changes (see Fig. 3 ).
Line scans can be acquired with or without enhanced depth imaging. To achieve this, instruments have incorporated enhanced depth imaging into their protocol to decrease the time delay of deeper structures with the zero reference. Fig. 4 shows enhancement in the depths to the choroid compared with Fig. 2 which does not show depth enhancement.
Quantitative scans
Retina
The macula thickness (see Fig. 2 ) can be quantified in micrometers in various areas. Fig. 5 shows an example of a patient with macula thickening caused by excessive fluid. The thickness decreases after fluid resorption.
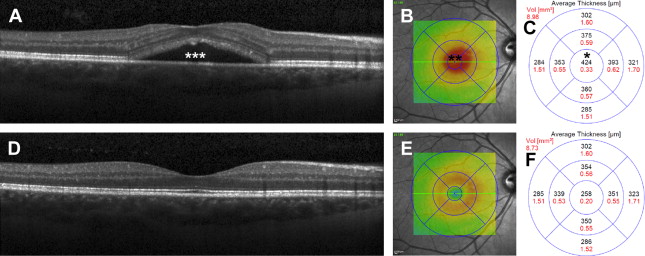
Optic Nerve
The optic nerve images, as illustrated in Fig. 3 C–D, have limited automated quantification that includes the cup-to-disc ratio and parameters of the neural rim tissue. Many clinicians will use instrument-based calipers to determine additional parameters including the height and width of the neural rim tissue and the elevation of the Bruch’s membrane opening that are monitored in conditions resulting in disc edema. Similarly, the rim tissue at the optic nerve head can be monitored for small changes through measures of the minimum rim width (MRW; see Fig. 2 B). However the circumpapillary retinal nerve fiber analysis below is an indirect way to quantitatively measure the optic disc swelling and optic atrophy.
Circumpapillary retinal nerve fiber layer analysis
The optic nerve can appear normal, swollen, or atrophic on OCT. The circumpapillary retinal nerve fiber layer (RNFL) represents the axons of the optic nerve that are adjacent to the optic disc before their entering the nerve. These can indirectly provide useful information about the state of the optic nerve. If the optic nerve is swollen, the RNFL analysis will show thickening, and if the optic nerve is atrophic, the RNFL analysis will show thinning. It may take time for the optic nerve fibers to reflect the damage that occurs acutely; therefore, normal-appearing optic nerve on OCT in the acute phase does not entirely exclude pathologies.
The OCT protocol that measures the thickness of the optic nerve axons (in a healthy eye) can be used as an indirect measurement and determination of optic nerve swelling or atrophy. This is the concept of the circumpapillary retinal nerve fiber layer analysis as shown in Fig. 6 (see Fig. 6 A–C, qualitative view, and D–F, quantitative analysis).
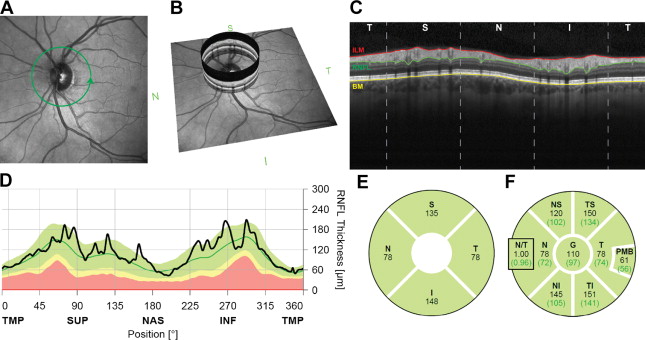
The mainstay for the retinal ganglion cell associated nerve fiber layer analysis is a 12° diameter circular scan centered on the optic nerve head (see Fig. 6 A, green circle) that are shown to have good repeatability. This scan shows a nominal circumference of 10.9 mm in the emmetropic eye and samples most of the retinal ganglion cell axons entering the optic nerve. To quantify the RNFL, an instrument-based segmentation algorithm identifies the inner limiting membrane and the junction between the nerve fiber layer and ganglion cell layer. RNFL thickness is traditionally reported as a TSNIT plot (A-scan thickness covering the temporal, superior, nasal, inferior, and back to temporal aspects in order). Fig. 6 C and D show the view in which the extreme left and right parts of the diagram represent the temporal aspect of the optic nerve (C is qualitative view and D–F is quantitative view).
The retinal ganglion cells are the neurons, which receive the input from the deeper retina (photoreceptors), and they deliver the visual information to the brain. The retinal ganglion cell axons form the retinal nerve fiber layer surrounding the optic disc. These axons migrate toward the optic nerve and bend nearly 90° to enter the nerve. The axons and the supporting cells/tissues (glia, oligodendrocytes, myelin, and blood vessels) form the optic nerve. Most of the optic nerve fibers synapse in the lateral geniculate body. Pathologies that affect the optic nerve before the lateral geniculate body will result in atrophy of the retinal nerve fiber layer. Usually, but not always (eg, with transsynaptic degeneration), pathologies that affect the visual pathway beyond the lateral geniculate body (eg, optic radiations and the occipital cortex) do not result in retinal nerve fiber changes, unless present at birth or very longstanding.
Most commonly, as previously stated, a normal average retinal nerve fiber layer (ARNFL) is associated with a normal complement of healthy optic nerve fibers. A normal ARNFL number is approximately 100, and as one ages this number reduces. A normal young person may have measurements of 80 to 120. However, a normal examination is not equal to a normal average nerve fiber, as there are several factors that determine if the retinal nerve fiber is normal for a particular person, to include history, prior OCTs, prior optic nerve edema, fundoscopic examination findings, congenital variations, right and left comparisons, and reliability of the study with signal strength.
Because the OCT comes with software that has age-matched normative data regarding the ARNFL, it automatically displays a color-coded analysis. The color code is displayed in a TSNIT plot (see Fig. 6 D; Fig. 7 A ), which is a graphic representation of the neuroretinal rim and RNFL thickness in micrometers that positions the obtained measurements of the right and left eye among the average normal (green), borderline (yellow), decreased (red), or increased (white) thickness subsets.
