Fig. 1.1
Depth dose characteristics of Photons Versus Protons showing the “bargg peak” in protons which is much closer to the ideal beam in external beam radiotherapy.
. Much before the clinical success of today’s megavoltage X-rays (photons) in precision radiotherapy, it was pointed out as early as in 1974 that on the basis of the physical factors such as higher LET (Fig. 1.2)
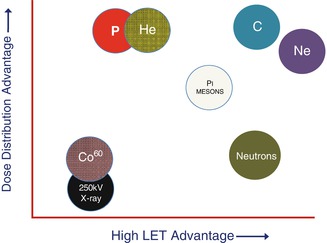
Fig. 1.2
Dr Raju’s idea demonstrating the relative advantage of particles proposed in 1974 representing the relative merits of high Linear Energy Transfer (High LET) particles
1.3 Facilities and Patients Statistics
Exact up-to-date statistics are available from PTCOG for each of the treating facilities all over the world. The numbers for each type of particle treated so far in the world are as follows. Patients treated with He are 2054 (1957–1992), with pions are 1100 (1974–1994), with C-ions are 15,736 (1994 to present), with other ions are 433 (1975–1992), and with protons are 118,195 (1954 to present) which makes the grand total as 137,179 as of the end of 2014. Of the total of about 137,000 patients treated with particle therapy worldwide from 1954 to 2014, 15,400 were treated in 2014 alone making it more than 10 % being treated only in last 1 year. In 2014, about 10 % of patients were pediatric and another 10 % were treated for ocular melanomas. Forty-eight particle therapy facilities were in clinical operation at the end of 2014. Of the total cases treated worldwide with particles, 86 % are treated with protons and 14 % are treated with carbon ions and with other particles. Five new particle therapy centers started patient treatments in 2014. This again is more than 10 % increase in facilities in 1 year. Further as on date, more than 30 more new particle therapy centers are under construction that will add about 80 treatment rooms in the near future. They are spread out all over the world with half of these are in the USA and one-third are in Asia. In 2015 about 15 centers were expected to start commissioning, and about half of them were planning to start treating patients before the end of 2015.
These figures are impressive and growth story of particle radiotherapy is truly exponential. Now there is a school of thought that as the ancillary technology for particle therapy is maturing now these data will further get strengthened and as the integrations become better we will have more success stories following in the near future. But there is another school of thought that we are having only incremental benefits which are very small and do not justify the kind of cost that a particle therapy facility requires. Though the growth story is impressive, will particle therapy be a viable alternative to photons if not replace it completely is a question that is still to be answered. The physics of particle radiotherapy has been a promising one since the beginning, but in an era of cost consciousness, the debate is should the limited resources of healthcare be spent on a technology which is expensive and may be the most expensive in medical sciences ever. Several of the authors in this book have pointed out as part of their discussions by quoting several studies of theirs as well as others that there are promises and pitfalls in particle radiotherapy. The real challenge today is to make this theoretically superior technology accessible and affordable to millions of cancer patients worldwide.
1.4 Physical Advantages Versus Clinical Realities
High LET radiation from particles as a more effective alternative to photons for routine clinical treatment of resistant tumors has not yet been addressed to the satisfaction of the medical fraternity. The reason for this is that though the physics of particles and their dose deposition capabilities are well understood, delivering them safely to the desired target area in a patient, the dose response and complications thereof are not fully understood. A considerable progress though has been made in understanding the radiobiology of the different particles that includes proton, carbon ions, helium ions, pi mesons, and neutrons initially proposed as choice of particles for radiotherapy [8]. High-energy X-rays (photons) have reached a point of saturation in radiotherapy; it is evident that they cannot further be improved only on the basis of dose distribution advantages. It is only with protons and particles (light and heavy ions) that we will be able to get further dose distribution advantages. Increasing radiation dose to tumor increases tumor control probability leading to higher cure rates in cancer. This was well recognized almost half a century ago that there is no “tumoricidal threshold dose,” and the higher the dose, the higher is the probability of radiation-induced cell killing [9]. Recent data from dose escalation studies with protons [10] in a randomized controlled trial shows superior long-term cancer control for men with localized prostate cancer receiving high-dose versus conventional-dose radiation. This was achieved without an increase in grade ≥3 late urinary or rectal morbidity. Similarly treatments with carbon ion have already shown significant improvements in local control as well as improved survival rates as has been reported by the working Group for Lung Cancer in Japan with carbon ion radiotherapy for stage I non-small cell lung cancer study [11] more than a decade ago.
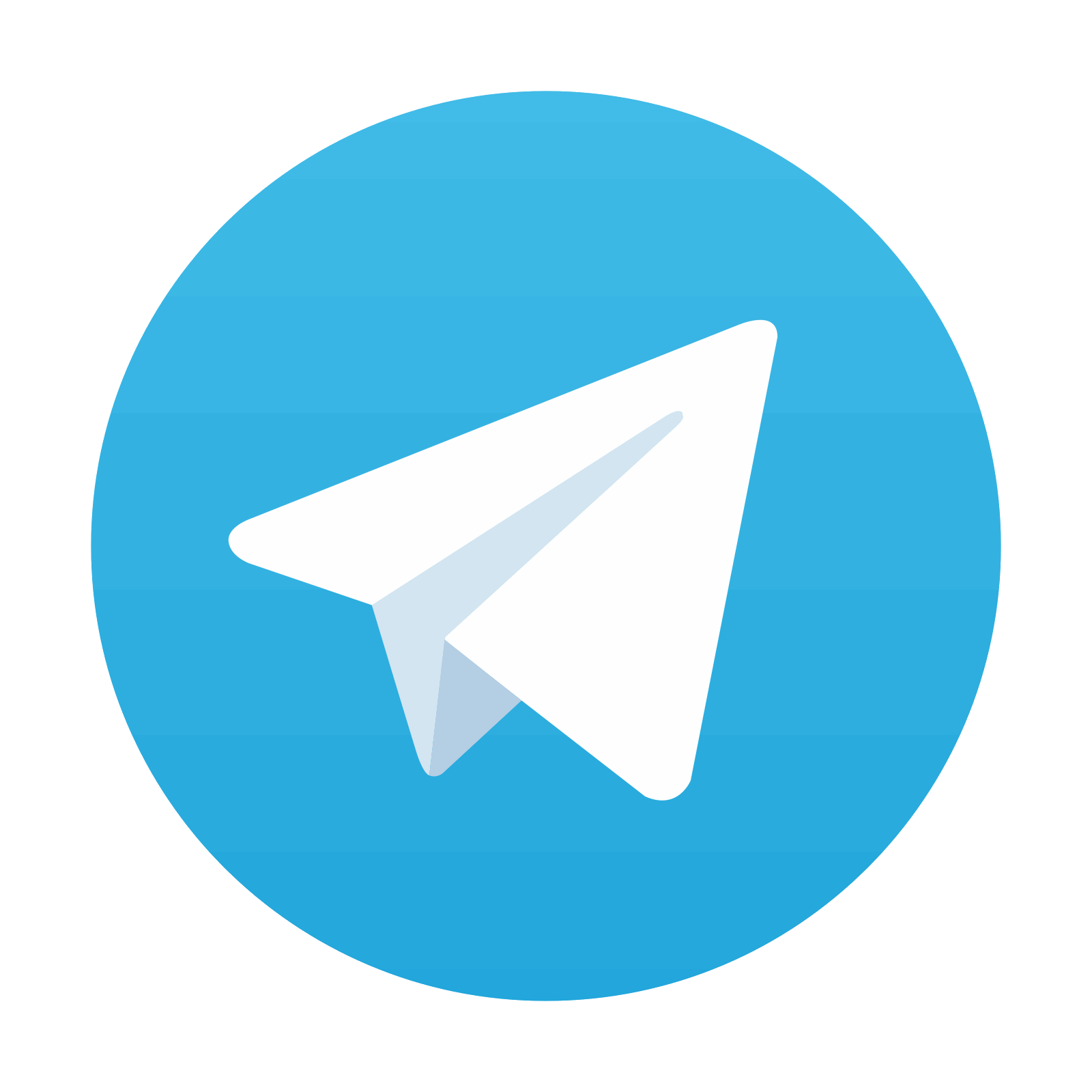
Stay updated, free articles. Join our Telegram channel

Full access? Get Clinical Tree
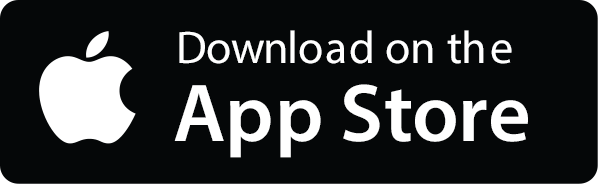
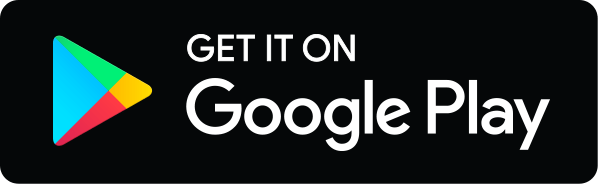