Pediatric Tumors
Torunn Yock
Thomas F. DeLaney
Brittany Esty
Nancy J. Tarbell
RADIATION THERAPY FOR PEDIATRIC TUMORS
Although the incidence of childhood cancer has stabilized over the last few years, approximately 12,000 new cases of cancer are diagnosed each year in the United States among individuals who are 19 years or younger.1,2 Common cancers in children include leukemia, lymphoma, central nervous system (CNS) tumors, neuroblastoma, Wilms’ tumor, and sarcomas.3 There have been statistically significant differences in the 5-year survival rates for children treated for cancer between the periods of 1974 to 1976 and 1992 to 1999, which are 56% and 78% respectively.4 The overall increase in the survival of these children has been attributed to advances in chemotherapeutic agents, refinements in bone marrow transplantation, improvements in surgical and radiation therapy techniques, and the centralization of care through cooperative oncology groups. Radiation therapy has played an important role in the treatment and cure of children diagnosed with malignant tumors. However, given that approximately 70% of children with malignancies are cured, the late effects of treatment have become a major focus for improvement of the chemotherapeutics, surgery, and radiotherapy.
Technologic advances in imaging5 and treatment planning have substantially changed radiation therapy for pediatric tumors. These advances have transformed the delivery of radiotherapy and were essential for the development of proton radiotherapy. Margins added around tumor for setup error have become much smaller due to improved immobilization devices6, 7, 8 and portal imaging ensures reproducibility of patient positioning on a daily basis and increased certainty about tumor location. Considering the profundity of these technologic advances, along with the sensitivity of pediatric tissues to radiation, protons are an excellent option when radiation therapy is needed. By eliminating exit dose, protons confine the high-dose areas to the target volume and minimize dose to surrounding normal tissue.
This chapter reviews the experiences and benefits of proton radiotherapy in the treatment of pediatric tumors. There is little experience to date with heavier charged particle radiotherapy for pediatric tumors with the exception of the osteosarcoma experience from National Institute of Radiological Sciences (NIRS) in Chiba, Japan, discussed in Chapter 15.
PROTON RADIOTHERAPY FOR PEDIATRIC TUMORS
Reduction of Acute Morbidity
Increasingly, the benefits of proton radiotherapy are being documented for both reduction of acute (within 90 days) and long-term morbidity (after 3 months). The acute toxicity depends on the location of the tumor and the area and volume of normal tissue treated. Treatment to the head and neck area can cause alopecia, dermatitis, folliculitis, conjunctivitis, otitis externa, otitis media, laryngitis, and pharyngitis. Acute toxicities from radiation to the thorax can include esophagitis, dysphagia, heartburn, pneumonitis, or pericarditis. Radiation to the abdomen and pelvis can cause anorexia, weight loss, nausea, vomiting, gastritis, enteritis, cystitis, nephritis, and/or hepatitis. Any neurologic symptoms produced by the tumor can worsen acutely with treatment but this is usually temporary. Acute symptoms from radiotherapy to tumors in the extremities are usually limited to skin reactions. If a large portion of the vertebral spine or pelvis is being treated, or if radiotherapy is combined with chemotherapy, bone marrow suppression can occur.
Proton radiotherapy reduces the acute morbidity by eliminating exit dose and thereby limits the dose to the
surrounding normal tissues. This is well illustrated in the case of craniospinal irradiation (CSI) for medulloblastoma (MB) (see Fig. 11.1). Because of the absence of exit dose, protons deliver no significant primary dose to bone marrow in the sternum and less dose to circulating hematopoietic cells, which should reduce myelosuppression. Exit dose to the heart, mediastinum, bowel, bladder, and other tissues anterior to the vertebrae is also eliminated. Absence of exit dose is especially relevant when only the thecal sac is treated (and not the whole vertebral body) in those patients who have completed the bulk of their bony growth.
surrounding normal tissues. This is well illustrated in the case of craniospinal irradiation (CSI) for medulloblastoma (MB) (see Fig. 11.1). Because of the absence of exit dose, protons deliver no significant primary dose to bone marrow in the sternum and less dose to circulating hematopoietic cells, which should reduce myelosuppression. Exit dose to the heart, mediastinum, bowel, bladder, and other tissues anterior to the vertebrae is also eliminated. Absence of exit dose is especially relevant when only the thecal sac is treated (and not the whole vertebral body) in those patients who have completed the bulk of their bony growth.
Figure 11.2 depicts the dose distribution on the planning computed tomography (CT) scan when only the thecal sac is treated, and demonstrates the fatty marrow replacement in the part of the vertebral body that is treated leaving the untreated marrow in the anterior aspect of the vertebral body capable of hematopoiesis. Prolonged myelosuppression and decreased bone marrow reserve caused by irradiation may limit the ability to give chemotherapy after radiation therapy. However, by reducing myelosuppression with proton radiotherapy, the child is less likely to require a treatment break in the chemotherapy. CSI treatment with protons also results in a reduction in gastrointestinal (GI) side effects, including nausea, vomiting, gastritis, and diarrhea, because of the absence of exit does through to gut.
Pelvic Ewing’s sarcoma is another example illustrating the benefits of proton radiotherapy in reducing acute morbidity. Proton radiotherapy allows for more precise conformation of the treatment volume to the target and therefore limits dose to critical structures. This is exemplified in Figure 11.3, which illustrates comparative dose distributions for treatment of a female patient with a pelvic Ewing’s sarcoma comparing protons and photons. The reduction of radiation given to normal pelvic structures ultimately decreases the acute toxicities including bladder and GI complications. However, the range modulation of the passively scattered proton beam to achieve optimal tumor coverage can produce higher skin doses with protons than photons, which can result in increased erythema and potentially moist desquamation This must be considered
when defining the target volumes (i.e., not including the skin in the target volume if it is not involved by tumor) and in choosing treatment portals. Increased skin toxicity is expected when the tumor is shallow and relatively thick.
when defining the target volumes (i.e., not including the skin in the target volume if it is not involved by tumor) and in choosing treatment portals. Increased skin toxicity is expected when the tumor is shallow and relatively thick.
Reduction of Long-term Morbidity
The developing tissues of children are at particular risk of long-term sequelae of radiation therapy. Long-term complications are specific to the normal tissues irradiated in the process of treating the tumor. Proton radiotherapy can dramatically decrease long-term complications associated with radiation treatment. CSI is, again, an excellent example. The potential long-term side effects from photon CSI include pulmonary, cardiovascular, ovarian, and GI toxicity, as well as potential effects on other normal tissues in the beam. Owing to the physical properties of protons, specifically the lack of exit dose, the proton beam delivers no dose to structures anterior to the target. As expected, the integral dose received by the lungs, heart, ovaries, bladder, and lower GI tract is decreased to zero, and therefore the long-term complications associated with conventional radiation therapy are no longer an issue in these organs. In other locations, although the dose cannot be reduced to zero, the dose to sensitive tissues can be minimized. To demonstrate the normal tissue/organ sparing that can be achieved using proton beams, we calculated treatment plans (for one patient) for an 18 Gy cranial posterior fossa boost using a three-field treatment technique (posterior obliques and a posteroanterior [PA] field). The results are shown in Table 11.1. Note the lack of exit dose to structures anterior to the posterior fossa using protons compared to the three-dimensional 3-D photon plan. This reduction in dose is expected to significantly reduce late radiotherapy-associated complications. These long-term complications including secondary malignancies, growth disturbance, neurocognitive dysfunction, and hearing loss will be discussed in greater detail in the following sections.
Secondary Malignancies
The risk of developing secondary malignancies in children treated for pediatric tumors is of great concern, and that risk is increased following radiation therapy. Radiotherapy can be very effective at eradicating tumor, but it is also a carcinogen and pediatric patients are more susceptible to radiation-induced tumors than adults.10 There is excellent evidence that the risk of a radiation-induced second malignancy can be reduced when less normal tissue is irradiated and when the normal tissue that is irradiated receives lower doses.10 Although intensity-modulated radiotherapy (IMRT) is a significant advance in radiation therapy because of the shaping of the high-dose contours off sensitive normal tissues (i.e., selective sparing of certain normal tissues), this is at the cost of a low/intermediate radiation dose bath to a generally larger volume of normal tissue. Therefore, IMRT is estimated to carry a greater risk of radiation-induced second malignancies.11,12 Proton radiotherapy, especially with scanned proton beams with reduced neutron exposure, is projected to reduce the risk
of secondary malignancies in children. Little has been reported to date on the projected risk of second malignancies with heavier charged particles.
of secondary malignancies in children. Little has been reported to date on the projected risk of second malignancies with heavier charged particles.
TABLE 11.1 COMPARISON OF DOSE TO NORMAL STRUCTURES FROM AN 18 GY POSTERIOR FOSSA BOOST WITH PROTONS OR 3-D PHOTONS | |||||||||||||||||||||||||||||||||||||||||||||||||||||||||||||||||||||||||||||||||||||||||||||||
---|---|---|---|---|---|---|---|---|---|---|---|---|---|---|---|---|---|---|---|---|---|---|---|---|---|---|---|---|---|---|---|---|---|---|---|---|---|---|---|---|---|---|---|---|---|---|---|---|---|---|---|---|---|---|---|---|---|---|---|---|---|---|---|---|---|---|---|---|---|---|---|---|---|---|---|---|---|---|---|---|---|---|---|---|---|---|---|---|---|---|---|---|---|---|---|
|
The projected reduction in secondary malignancies with proton radiotherapy results from the decrease in normal tissue receiving radiation. Yock et al.13 recently reported on seven patients treated with proton radiotherapy for an orbital rhabdomyosarcoma (RMS) at Massachusetts General Hospital (MGH) and the Harvard Cyclotron Laboratory (HCL) from 1995 to 2001 who received a median dose of 45.8 Gy (RBE).14 This study generated a proton and photon plan for each of the seven patients, from which dose-volume histograms (DVHs) were obtained for comparison purposes. For every patient, the proton plan irradiated less normal tissue compared to the photon plan. On the basis of this reduction in normal tissue irradiation, we expect that patients treated with proton radiation therapy will be at a lower risk for developing second malignancies than patients treated with photons. According to one modeling study, the projected risk of a second malignancy for children irradiated for orbital RMS was 25% to 50% of the risk with photon techniques.11
Recently, a conflicting hypothesis regarding the reduction in secondary malignancies with proton radiotherapy has been presented, which relates to the secondary neutrons from the scattering foils used to spread out passively scattered protons as well as other beam-modifying devices. The scattered neutrons deliver dose to the patient outside of the primary proton treatment fields. This additional dose and volume treated could result in an increase in the risk of secondary malignancies, given the higher quality factor of neutrons.15 This hypothesis has generated considerable controversy, but has raised several very important points. Magnetically scanned protons can avoid the neutrons produced by scattering foils and other beam-modifying devices and therefore should be employed preferentially over passively scanned protons whenever technically feasible. The secondary neutrons generated by a passively scattered beam line will be highly dependent upon the particular features of a given beam line as well as beam-modifying devices.16,17 The neutron figures for a passively scattered proton beam line used in the projections by Hall15 were modified from measurements obtained at the old Harvard Cyclotron Laboratory (HCL); recent measurements taken at the University of Indiana facility16 documented neutron doses that were 2 orders of magnitude lower than those cited from the HCL, suggesting that this may not be as large a potential problem as originally proposed.
Growth
Growth disturbances are another long-term complication associated with radiation therapy in pediatric patients. Tissues such as muscle, bone, and organs irradiated in a growing child often fail to develop normally, which can result in asymmetries and significant cosmetic and/or functional consequences. The degree of growth and developmental stunting is related to the particular tissue, as well as the volume of tissue treated and dose delivered. Proton radiotherapy has less effect on growth and development due to the reduction in the volume of normal tissue irradiated. In contrast, IMRT may have some worrisome consequences in this context because it does irradiate markedly greater volume of normal tissue, albeit to low or moderate doses.12
Neurocognitive Toxicity
A large number of studies in the literature have documented a decline in intellectual and cognitive function in children who have received whole brain radiotherapy (WBRT) for conditions including MB, other pediatric brain tumors and acute lymphoblastic leukemias.18 The decline in function can be significant, often necessitating placement in special education programs and other therapeutic interventions. The effect of radiation depends upon the age of the child, with younger children significantly more susceptible to the effects of radiation than older children. The effects of
radiation also depend on the dose of radiation, with higher doses having more deleterious effects. The major radiation insult to the CNS is demyelination with subsequent white matter necrosis and leukoencephalopathy seen on magnetic resonance imaging (MRI). However, often no structural changes are noted on standard MRIs even when there has been a decline in neurocognitive functioning. Most CNS development occurs during the first 3 years of life, but myelination continues through puberty, and disturbances in the myelination process are linked to neurocognitive effects.19
radiation also depend on the dose of radiation, with higher doses having more deleterious effects. The major radiation insult to the CNS is demyelination with subsequent white matter necrosis and leukoencephalopathy seen on magnetic resonance imaging (MRI). However, often no structural changes are noted on standard MRIs even when there has been a decline in neurocognitive functioning. Most CNS development occurs during the first 3 years of life, but myelination continues through puberty, and disturbances in the myelination process are linked to neurocognitive effects.19
Neurocognitive deficits following cranial irradiation can be significant. The Children’s Hospital of Philadelphia (CHOP) analysis of children treated there for cerebellar astrocytoma compared 26 patients receiving WBRT with 21 patients receiving no radiotherapy (RT). Intelligence quotient (IQ) declined by a median of 13 points in the irradiated group versus none in the observed group.20 Hoppe-Hirsch et al. compared 59 children with MB who received WBRT to 37 children who received posterior fossa RT for ependymoma.21 In this retrospective study, all patients underwent irradiation of the posterior fossa (45 to 55Gy) after surgery, with an age distribution where 38% of the children with ependymoma and 13.5% of those with MB were younger than age 3. The children treated for MB received additional prophylactic whole brain irradiation in addition to the posterior fossa boost to either 38 Gy or 25 Gy. Only 20% and 10% respectively of the MB patients who received whole brain radiation had an IQ above 90 at 5 and 10 years after radiotherapy whereas 60% of the ependymoma patients retained IQs above 90 at 5 and 10 years from treatment. This progressive deterioration in the MB patients was most likely secondary to the radiotherapy to the cerebral hemispheres.21 For MB treatment, the use of proton irradiation to boost the posterior fossa or involved field significantly reduces the volume of normal supratentorial brain tissue irradiated, and this reduction is expected to translate into less drastic neurocognitive deficits due to treatment, as the volume of brain irradiated correlates with deterioration of intellectual performance.22
Pediatric brain tumors are commonly treated at the Francis H. Burr Proton Therapy Center (FHBPTC), and treatment details and follow-up, including neuropsychologic evaluations, are documented in the Pediatric Brain Tumor Database at MGH. The database tracks all pediatric patients treated with proton radiotherapy for brain tumors. Eventually, once enough follow-up data is obtained, we will have the ability to directly measure the neurocognitive benefits of proton radiotherapy for various tumors, volumes, and doses. We expect to see less neurocognitive complications associated with radiation therapy with proton treatment compared to photon techniques.
Ototoxicity
Sensorineural hearing loss (SNHL) caused by damage to the cochleae and auditory nerves is a late complication from RT and can result in permanent hearing loss. Evidence suggests a dose response relation between the radiation dose received and resulting hearing loss. Grai et al. observed a higher incidence of severe SNHL in nasopharyngeal carcinoma patients receiving >50 Gy to the inner ear.23 Chemotherapy drugs such as cisplatin and carboplatin can cause high frequency SNHL, and recent data suggest that the combination of chemotherapy and RT can cause more serious ototoxicity. Packer et al. reviewed their pediatric MB patients treated with concurrent vincristine plus CSI followed by adjuvant cisplatin, lomustine (CCNU), and vincristine. They found that 47.6% of patients developed grade 3 to 4 ototoxicity.24 Therefore, radiation oncologists have become even more focused on sparing the cochleae from radiation. This was not as well done with conventional 3-D plans but is more readily achievable with IMRT;25,26 however, proton radiation conforms more tightly around the posterior fossa both to spare the cochleae and the rest of the brain.27,28 Through sparing of the cochlea and auditory nerves, it is expected that proton radiotherapy will decrease the ototoxicity associated with radiation therapy.
Intensity-Modulated Proton Radiation Therapy
Intensity-modulated proton radiation therapy (IMPT) (see Chapter 8) can improve the conformality of treatment and sparing of normal tissue. Miralbell et al. assessed the potential influence of improved dose distribution with proton beam radiation as compared to 3-D conformal photon radiation and IMRT on the induction of second malignancies. The authors also looked at IMPT, which is currently available only on a limited basis for clinical treatment but likely will be available more widely in the near future. Treatment plans were compared for one patient with a parameningeal RMS (paranasal sinus) and for one patient with MB.11 The expected risk of radiation-induced malignancy for the child treated for parameningeal RMS with IMPT was almost 2.4 times lower than the conformal photon plan and about half the risk expected for IMRT. For the MB patient, only three treatment plans were generated using conformal photons, IMRT, and conformal protons. The second malignancy risk with protons was reduced >8-fold compared to IMRT and >14-fold compared to conformal photons, also emphasizing that the relative advantage for protons generally increases as the target volume increases. Protons (with or without intensity modulation) decreased the estimated risk compared to photons (with or without intensity modulation).
In addition, Mu et al.29 performed a comparison study to explore different spinal irradiation techniques with respect to the long-term side effects associated with them. Their study determined that the lowest lifetime risk of secondary cancers was found using IMPT. The risk associated with IMPT was 4%, compared to conventional photon therapy,
electron therapy, and intensity-modulated electron therapy, which were 20%, 21%, and 15% respectively.29
electron therapy, and intensity-modulated electron therapy, which were 20%, 21%, and 15% respectively.29
SPECIFIC DIAGNOSES
Medulloblastoma
MB is the most common malignant pediatric brain tumor, accounting for 15% to 20% of all pediatric brain tumors, with a median age at presentation of 6 to 7 years. The tumors, composed of immature small round blue cells, arise in the posterior fossa and have a predisposition to subarachnoid dissemination in the CNS.30 The cure rate with conventional radiotherapy and chemotherapy for patients who have standard-risk disease (see criteria in the following text) is approximately 80%, but for those with high-risk disease it is lower.24,31,32
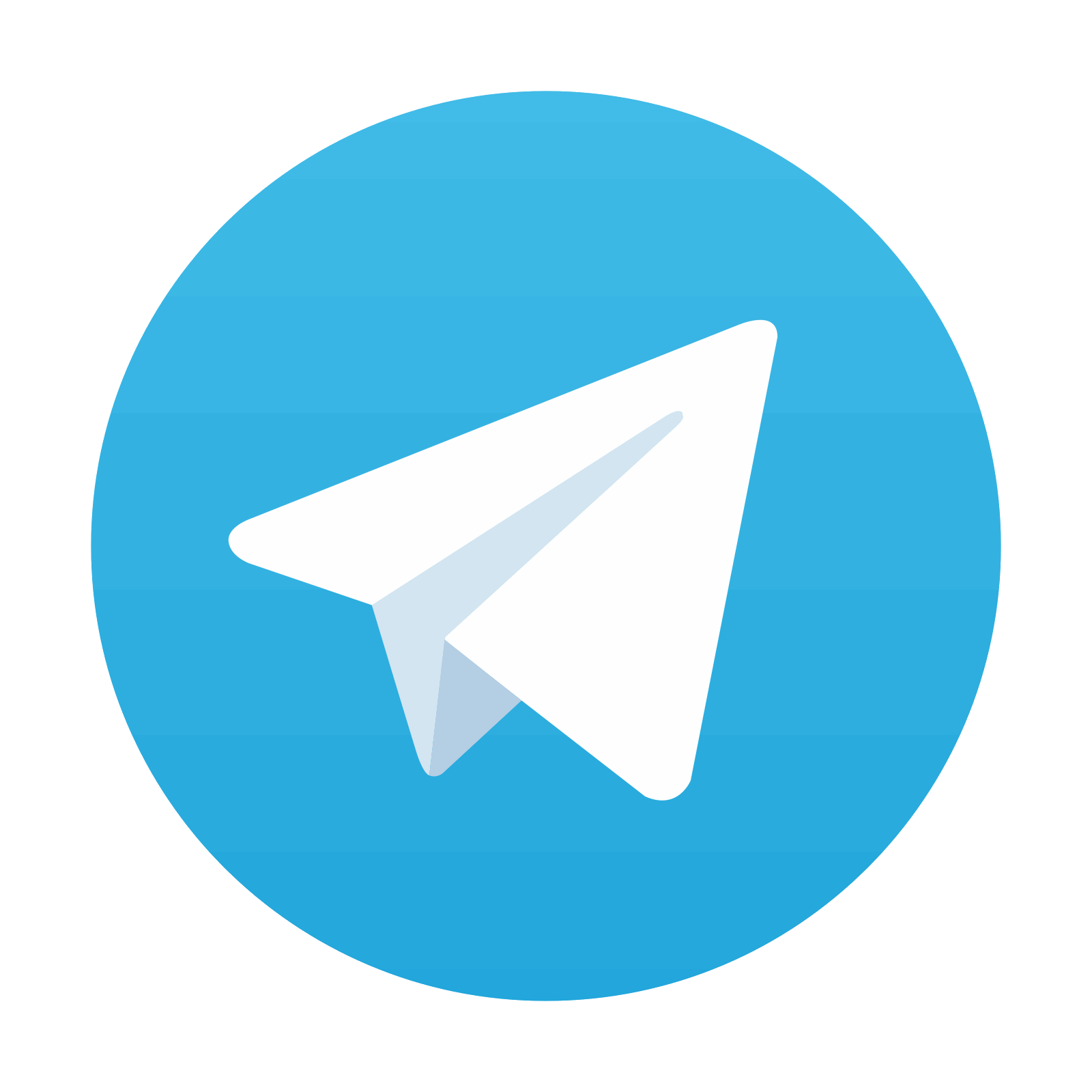
Stay updated, free articles. Join our Telegram channel

Full access? Get Clinical Tree
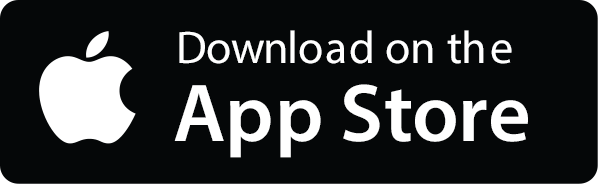
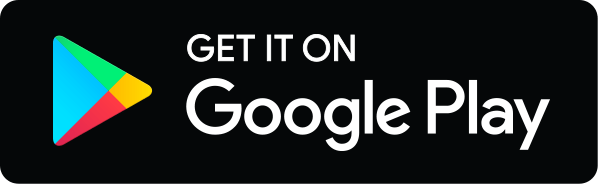