PET and PET-CT in Breast Carcinoma
Richard L. Wahl
Although breast cancers accumulate fluorodeoxyglucose (FDG) relatively avidly, as well as a variety of other positron emitter–labeled metabolic tracers, the FDG uptake is somewhat lower in breast cancer than in other common cancers. With conventional whole-body positron emission tomography (PET) scanners, small primary tumors under 1 cm in diameter commonly escape detection. Evolving higher-resolution dedicated breast PET imaging devices may offer greater detection capability for these small lesions. Nonetheless, many primary breast cancers can be imaged, and intense focal uptake in the breast incidentally detected on PET or PET–computed tomography (CT) warrants careful evaluation for possible cancer. PET and PET-CT can detect metastatic breast cancer to axillary lymph nodes and internal mammary nodes, but the sensitivity is typically about 75% to 85%, less than either axillary dissection or sentinel node sampling for axillary metastases (see Fig. 45.2).
PET is excellent for detecting soft-tissue and systemic metastatic disease, including lytic bone metastases, but it can be falsely negative in blastic bone metastases. Using PET to monitor treatment response is an attractive method and allows individualization of therapy. The glycolytic metabolic rate of breast cancers falls rapidly with effective chemotherapy, at a rate considerably faster than the decline in tumor size with treatment. A lack of decline in FDG uptake with treatment suggests that the therapy with be ineffective in the long-run.
At present, PET and PET-CT are used most commonly to determine the extent of systemic disease and to quickly monitor the response of primary and metastatic cancers to treatment. PET-CT generally is preferred to PET alone, as it appears somewhat more accurate and provides superior diagnostic certainty. This chapter reviews the current status of PET and PET-CT for the detection and characterization of primary breast cancers, for locoregional and systemic tumor staging and restaging, for predicting and assessing tumor response to therapy, and for changing patient management.
Introduction
As discussed elsewhere in this book, cancers differ functionally and to some extent anatomically from normal tissues, allowing detection by functional and anatomic as well as hybrid imaging methods. Like many epithelial cancers, breast cancers have a variety of phenotypic deviations from normal breast tissue. These include but are by no means limited to the following:
Increased tumor blood flow and increased vascular permeability compared with normal breast tissue (the physiology by which gadolinium contrast magnetic resonance imaging (MRI) of breast cancer appears to produce its signal);
Increased levels of glucose metabolism;
Increased amino acid transport and protein synthesis;
Increased receptor expression (such as overexpression of the estrogen receptor);
Increased DNA proliferation rates; and
Hypoxia in many of tumors.
All of these processes can be imaged with positron emission tomography (PET). Although PET tracers targeting these processes have been evaluated to some extent in breast cancer, most clinical work using PET in breast cancer
imaging has been performed with the tracer fluorodeoxyglucose (FDG) (1,2).
imaging has been performed with the tracer fluorodeoxyglucose (FDG) (1,2).
We and others have shown that the vast majority of breast cancers overexpress the glucose transporter molecule GLUT-1 and that there is a general relationship between the viable cancer cell number and the FDG accumulation in primary breast cancers. There is a correlation between the GLUT-1 levels, the number of viable cancer cells, and the FDG accumulation in these tumors (3,4,5). In addition, there is a positive relationship between the intensity of FDG uptake and the degree of aggressiveness of breast cancers (6). By contrast, measured total hexokinase levels are not well correlated with the extent of FDG uptake into breast cancers (7).
Initial human study of breast cancer imaging with PET evaluated tumor blood flow, oxygen extraction, and oxygen utilization in nine patients using carbon 11 (11C)- and oxygen 15 (15O)-labeled tracers. In these studies, regional blood flow was higher in the tumors than in surrounding normal breast. Although oxygen utilization was slightly higher in the tumors than in normal breast, the oxygen extraction ratio was significantly lower (8). A subsequent study in 20 patients showed mean tumor blood flow to be about five to six times higher than flow in normal breast tissue (9). While PET can image tumor blood flow, this sort of tracer application has only limited clinical application. The short half-life of 15O (2 minutes) is only practical at sites located near a medical cyclotron, and it remains a research tool for imaging breast cancer at the present time.
Several other studies of the use of PET for imaging tumor blood flow were reported recently. Mankoff et al. reported that changes in tumor blood flow using [15O]water were predictive of an early response to therapy (10,11). Zasadny et al. showed that FDG uptake and [15O]water are somewhat correlated (12).
Wahl and colleagues have preliminarily reported on the use of the generator-produced agent 62C-PTSM for breast cancer imaging. They demonstrated the feasibility of using this radiotracer, which can be substituted for 15O to measure blood flow, for imaging some primary and systemically metastatic breast cancers (13).
PET imaging of estrogen receptors in breast cancer has been performed for some time, mainly in the research setting. Thirteen women with primary breast masses were imaged with 16 alpha-[18F]-fluoro-17 beta-estradiol (FES) (14). PET demonstrated uptake of the radiotracer in the primary breast mass and the diseased axillary nodes in several cases. An excellent correlation (r = 0.96) was reported in this study between the estrogen-receptor concentration measured in the tumors and the extent of FES uptake into the tumors. A larger follow-up study by the same investigators evaluated in 16 patients with 57 known foci of metastatic disease and demonstrated 93% sensitivity for lesion detection (15). Patients who received antiestrogen therapy showed a decline in the fraction of FES reaching the tumors post-treatment (15). Imaging tumors that were estrogen receptor–negative was not successful with this approach. Despite the early and promising work with this agent, it has not achieved widespread clinical utilization, in part because it obviously does not target estrogen receptor–negative tumors and because treatment trials with antiestrogen agents have a relatively low risk. Fluoroestradiol-based tracers have been used most extensively in the research setting. Recent data on aromatase inhibitors suggest that such treatments might be quite useful in breast cancer therapy.
Tracers of proliferation, such as (18F)-3′-deoxy-3′-fluoro-l-thymidine (18F-FLT), have recently been applied to a limited extent in breast cancer imaging. In a recent pilot study of eight primary tumors imaged before treatment, all eight tumors were detected by 18F-FLT-PET, with a mean standardized uptake value (SUVmean) of only 1.7 and a mean tumor-nontumor ratio (TNT) of 5.0 (16). FLT only detected two or seven patients with axillary metastases, and these two patients’ axillary metastases were quite large lesions and clearly palpable. FLT does appear to image the proliferation rate of breast cancers quite accurately. However, as in a study of tumor, K(i) and fractional retention of radiotracer determined by spectral analysis correlated with the Ki-67 labeling (proliferation) index (r = 0.92, p < 0.0001 and r = 0.92, p < 0.0001, respectively). In this study, primary, axillary, and systemic metastases could be detected in many cases (17). While FLT is an interesting tracer, it has relatively low uptake into breast cancers, which may limit its clinical utility as a detection agent for smaller tumors, although more study is needed.
FDG is currently by far the most commonly used PET tracer to image breast cancer. As discussed elsewhere in this book in detail, this radiopharmaceutical is transported into breast cancer cells, most likely substantially by the facilitative glucose transporter protein GLUT-1, which is typically overexpressed in many breast cancers. It is then phosphorylated by hexokinases, especially HKII, in cancer cells to FDG-6-phosphate, a polar material that is retained within the cell (1). PET imaging detects the intracellular FDG-6-phosphate in tumors. l8F has a half-life of 109 minutes, so it is more practical for use in a clinical setting than the very short lived positron-emitting isotopes. Much of the FDG used in the United States and to some extent in the rest of the world is at medical centers that are remote from a medical cyclotron, and it sometimes comes from a manufacturing site hundreds of miles away from the PET camera.
FDG was first introduced for breast cancer imaging by a planar imaging (non-PET) technique by Minn et al. in 1989 (18). Using a specially collimated gamma camera (non-PET, nontomographic), the investigators studied 17 patients with breast cancer and were able to detect the tumor in 14 (82%), including 6 of 8 known lymph node metastases. FDG was also able to detect bone metastases and was more sensitive for detecting lytic or mixed lesions than purely sclerotic lesions. In assessing treatment response in ten patients, increased FDG uptake was consistently associated with disease progression, while declines
in FDG uptake were often, but not invariably, associated with resolving or stable disease (18). However, planar imaging is a very insensitive technique, and images are limited by low resolution and sensitivity.
in FDG uptake were often, but not invariably, associated with resolving or stable disease (18). However, planar imaging is a very insensitive technique, and images are limited by low resolution and sensitivity.
In preliminary reports in 1989, Wahl and Kubota separately reported on the feasibility of imaging breast cancer using PET with FDG in several patients (19,20). Subsequently, in a larger series, Wahl et al. showed the feasibility of imaging the primary tumor and the regional and systemic metastases of breast cancer using FDG-PET (21). The FDG-PET method detected 25 of 25 known foci of breast cancer, including primary lesions (10), soft-tissue lesions (5), and bone metastases (10). Four additional nodal lesions that had not been previously identified were also detected. Several of the primary cancers were detected in women with radiographically dense breasts. It must be noted, however, that the primary lesions evaluated were all greater than 2 cm in size and therefore larger than most cancers detected by screening mammography.
In preclinical studies, FDG uptake in vitro and in vivo in breast cancer declined with rising glucose levels, suggesting strongly that PET imaging should be performed in the fasted state (22). These results have been confirmed clinically in various human cancers, and all PET centers perform breast cancer imaging in fasting patients (23). Uptake of the tracer is lower in diabetics than in euglycemic patients.
In general terms, the use of FDG-PET in breast cancer imaging can be discussed in terms of evaluation of the primary lesion, evaluation for regional and distant metastatic disease, and evaluation of treatment response and overall in the context of patient management approaches. Unless otherwise stated, the following results were obtained with whole-body dedicated PET scanners and not with the more recent technologies of dedicated PET breast-imaging devices or PET-CT, which are discussed at the end of this chapter.
Evaluation of Primary Lesions
Early changes in the molecular characteristics of breast tissues result in physiological changes, which then induce the anatomic alterations we recognize as “cancer”; imaging with mammography has long been the standard method of detecting primary breast cancers. Mammography, however, detects the mass or the calcifications produced by the genetic changes that caused the tumor to develop. In many instances, the appearance of benign and the appearance of malignant masses and calcifications on anatomic imaging overlap with one another. This overlap in anatomic appearance often requires that biopsies be performed to determine the nature of the lesion. In the United States, about 70% to 80% of breast biopsies result in benign histological samples and thus represent false-positive anatomic imaging tests. Compared with the simple anatomic imaging approaches, functional imaging of tumor biology holds the promise of substantially reducing the number of false-positive biopsies and increasing the overall accuracy of imaging. Mammography is sometimes falsely negative as well, especially in women with radiodense breast tissues (2).
While the study of Wahl et al. succeeded in detecting untreated primary lesions of breast cancer by FDG-PET (as well as locoregional and systemic metastases), this small study demonstrating proof of the concept was restricted to large primary lesions and did not address the more critical and clinically relevant issue of detection of smaller lesions in the breast (21). Since imaging devices for PET have been gradually improving, past results may not always be indicative of the current state of the art in PET. Higher resolution systems are now available, and thus smaller lesions should be detectable.
Using whole-body PET imaging without attenuation correction, Hoh et al. were able to detect 15 of 17 foci of primary breast carcinoma as well as regional and systemic metastases (24). In a study of transverse PET with attenuation correction in 11 patients with primary breast cancer, 10 of 11 primary lesions were identified by FDG-PET (25). Only modest uptake was seen in patients with fibrocystic disease. The tumor-to-normal-tissue uptake ratio was 4.9:1. In a larger prospective study, Adler et al. reported FDG-PET results using attenuation correction and PET in 28 patients with 35 suspicious breast masses. Twenty-six out of 27 malignant lesions were identified (sensitivity, 96%) and separated (in retrospect) from the 8 benign breast lesions (26). The separation was based on the FDG uptake levels in the primary lesions. In this study, there was a modest but significant correlation between the pathological nuclear grade of the tumors and the quantity of FDG uptake.
Dehdashti et al. evaluated 32 breast masses, of which 24 were malignant, using FDG-PET. They found FDG uptake to be much greater, on average, in the breast cancers than in the benign lesions (SUV = 4.5 ± 2.8 versus 1.05 ± .41), and using a 2.0 SUV cutoff value for diagnosis of malignancy, they achieved an 88% sensitivity and a 100% specificity for detection of primary breast lesions (27).
For FDG-PET, Bassa et al. reported a sensitivity of 100% for the detection of locally advanced primary breast cancers and a sensitivity of 77% for the detection of nodal metastases prior to treatment (28). These results were better than those obtained by anatomic imaging methods. The detection of some primary breast cancers that are locally advanced may be challenging anatomically, but this high sensitivity value must not be considered representative of the performance of PET across a full range of primary lesion sizes. Small lesions will clearly be more difficult to detect than larger lesions due to the size-based resolution and sensitivity limitations of dedicated PET scanners, which typically have approximately 10 mm FWHM (full width at half maximum) reconstructed resolution. Evaluation of the method in patients with suspected but undiagnosed primary lesions of all sizes is more clinically relevant.
Avril et al. initially reported findings in 51 patients with 72 suspicious breast masses, 57% of which proved to be malignant. In this study, malignant lesions had a mean SUV 2.5 times that of benign lesions (29). By correcting for partial-volume effects, sensitivity was increased from 75% to 92%, while specificity was decreased from 100% to 97%. The detection of lesions less than 1 cm in diameter was not optimal, however, due to resolution limitations of the whole-body camera used in the study.
This same group subsequently reported findings in 185 patients with breast cancer using FDG. These included 132 cancers and 53 benign lesions. The overall sensitivity was much lower, only 64%, and the specificity was 94%, using standard visual analysis. When a lower visual threshold of malignancy was used in interpreting these same studies, the sensitivity increased to 80% and the specificity declined to 76% (30). Nearly two thirds of invasive lobular cancers were FDG-PET negative, compared with about 24% of invasive ductal cancers. In this study, there was a clear relationship between tumor size and lesion detectability. Using the most sensitive visual detection approach, only 68% sensitivity was obtained in primary tumors less than 2 cm in diameter. Sensitivity was higher for larger lesions, reaching 92% for 2- to 5-cm lesions. All three lesions over 5 cm in diameter were detected (30). Lobular carcinomas have low GLUT-1 expression, likely accounting for the lower sensitivity of PET in these types of tumors (30).
Results similar to those obtained in this large series were reported by Yutani et al., who found a 79% sensitivity for detecting primary breast cancer (31). They also showed that FDG clearly achieved higher target-to-background ratios than technetium 99m [99mTc]sestamibi (MIBI) (6:1 vs. 3.5:1), again indicating that not all tracers are equivalent for breast imaging (31). Both lesion histology and size affect the rate of primary cancer detection using FDG-PET. Certainly, a technique with low sensitivity is not suitable for screening, so negative PET findings in lesions less than 2 cm in diameter have limited negative predictive value.
Positive PET results must always be considered of serious concern, with very high and focal FDG uptake having a strong positive predictive value for cancer. Ishimori reported the discovery of two incidental breast cancers out of 1,912 patients studied with FDG-PET-CT for recurrent tumors of other types (32). Thus, PET and PET-CT can detect some breast cancers that are not detected by other methods and that are incidental. Recent reports have shown that FDG-PET can detect nonbreast primary malignant disease in the breast in diseases such as synovial sarcoma (33) and non-Hodgkin lymphoma (34).
The low sensitivity of current whole-body imaging devices for detecting smaller primary breast cancers makes PET less than optimal for screening, even in high-risk populations. However, the performance characteristics of PET compare very favorably with those of other noninvasive imaging modalities and are better than those of planar scintillation camera imaging of MIBI when they have been directly compared. FDG-PET has also been able to detect primary lesions in patients with silicone breast prostheses, which are opaque to standard low-energy mammographic x-rays (35,36).
The small studies directly comparing FDG-PET and MRI have shown that FDG-PET is typically less sensitive than MRI for primary lesion detection but more specific than MRI. As an example, in 42 breast lesions, MRI was 89% sensitive and 63% specific for cancer. By contrast, PET was 74% sensitive and 91% specific (37). Similarly, Goerres et al. showed PET to be 79% sensitive, 94% specific, and 84% accurate, compared with MRI, which was 94% sensitive, 94% specific, and 88% accurate, in detecting possible recurrent tumor in the breast following a known diagnosis of breast cancer (38). FDG-PET using whole-body imaging devices appears to be more specific but less sensitive than MRI.
The relationship between the MRI signal and the FDG-PET signal was studied in 20 patients with large or locally advanced primary breast cancer. A significant association (p < 0.05) was observed between the calculated exchange rate constants of both pharmacokinetic models and SUV determined by FDG-PET. This was a modest relationship, accounting for less than half of the findings. Thus, the SUV and the MRI enhancement rate are related but not equivalent (39). As with most tracers, delivery does have a role in the total signal detected.
FDG is currently the dominant tracer in breast cancer imaging, as it is in most cancers, but the use of alternative tracers has been explored to some extent in breast cancer, as discussed briefly earlier in this chapter. The studies have generally included a small number of patients. [11C]-L-methyl-methionine PET has been used in the imaging of primary breast cancers and in the assessment of their response to chemotherapy. In a pilot study of primary and metastatic breast cancers, [11C]-L-methionine uptake in primary breast cancer was less than in the liver but more than in most normal tissues. All tumors over 3 cm in size were detected, but three smaller tumors were not detected. Increased uptake of [11C]-L-methionine was associated with a large S-phase fraction (SPF) measured with flow cytometry (r = 0.77, p = 0.01). These data indicate that [11C]-L-methionine can be used to image breast cancers but weigh against its being useful in small lesions or in the liver due to high background uptake (40). The need for an onsite cyclotron for use of [11C]-L-methionine makes its widespread clinical application even less likely. However, other tracers with higher uptake into breast tumors and lower background activity could be developed, making the PET method more potent for assessing primary lesions even with existing imaging systems.
At present, FDG-PET is an excellent method for helping to detect primary breast lesions over 2 cm in diameter and to characterize such lesions. The exact clinical role of PET in breast imaging continues to evolve. The performance of PET will likely improve as better resolution scanners
increasingly become available (both dedicated to the breast and for whole-body applications). It should be noted that in the United States the use of noninvasive diagnostic tests to help characterize a lesion as malignant or benign is especially challenging because a missed diagnosis of cancer often leads to litigation. For this reason, biopsy and excision remain widely used as the primary approach to the diagnosis of breast cancer in the United States following lesion detection by anatomic methods.
increasingly become available (both dedicated to the breast and for whole-body applications). It should be noted that in the United States the use of noninvasive diagnostic tests to help characterize a lesion as malignant or benign is especially challenging because a missed diagnosis of cancer often leads to litigation. For this reason, biopsy and excision remain widely used as the primary approach to the diagnosis of breast cancer in the United States following lesion detection by anatomic methods.
Detection of Primary Lesions with Positron Cameras Dedicated to Breast Imaging
Although FDG is the most common tracer used in breast cancer imaging with PET, it should be noted that nearly all PET scanners used in breast imaging to date have been designed as whole-body scanning devices, not optimized to image the breast alone but rather constructed to function as all-purpose devices. In performance, they represent a compromise as compared with dedicated breast-imaging instruments, analogous to using a chest x-ray device to perform mammography and thus obtaining less than optimal breast images. Dedicated breast-imaging devices have more potential for successful imaging of small lesions in the breast than whole-body PET scanners.
This area is in rapid development, and to date only studies with a small number of patients have been performed. In principle, a smaller PET imaging device devoted to imaging the breast would avoid much of the soft-tissue attenuation faced by whole-body PET scanners. The devices could be less expensive yet more sensitive than whole-body PET scanners and could lead to development of radionuclide-guided biopsies (41). They are, however, limited by partial-volume effects and the degree of the localization of FDG or other PET tracers in the tumor compared with normal breast (42).
Raylman reviewed issues of detectability with FDG positron emission mammography (PEM) in phantom studies. In brief, in the absence of breast background, simulated breast lesions as small as 5 mm could be detected, However, as background activity was increased to the levels expected in women with more metabolically active breasts, and as breast thickness also was increased, only lesions 12 mm in diameter and larger were detected. This indicates that dedicated breast devices will likely do better than whole-body tomographs and that the role of FDG and PET in evaluating primary breast lesions will require continued re-evaluation as improved instruments are developed (42). The reliable detection of lesions smaller than 12 mm is essential for any method to achieve clinical utilization.
Several groups have been carrying out studies with dedicated high-resolution PET breast-imaging devices. Gagnon et al. have shown the feasibility of PEM devices in patient studies (43), and more sensitive devices are being made using different detector materials (44). One of the other potential benefits of dedicated devices is that their images could be coregistered with those of a mammographic imaging device to display both anatomic and PET data simultaneously (45). Recently, using dedicated breast-imaging devices, sensitivities of about 86% and specificities of 91% were reported for a dedicated positron breast-imaging device that has a resolution of less than 2 mm (46). Using this dedicated breast-imaging PET device, “PEM,” in a prospective multicenter study, 44 women with confirmed breast cancers were imaged with a high-resolution PEM scanner (Naviscan PET Systems, Rockville, MD) with FDG. The sensitivity of PEM was 89% for the primary cancer, and an additional 4 incidental primary breast cancers were identified with PEM. Of the 19 patients undergoing breast-conserving surgery, PEM correctly predicted 6 of 8 patients (75%) with positive margins and 11 of 11 (100%) with negative margins (47).
Using a newer large-field-of-view, high-sensitivity detector system, positron emission mammography demonstrated 20 focal abnormalities, of which 18 were proven to be malignant and two benign by histology. Three of 20 histologically malignant lesions detected by conventional mammography were not demonstrated by PET mammography. The overall sensitivity of PET mammography for malignancy was 86%, with a positive predictive value of 90% in this small series (48).
PET and positron emission mammography must also be considered in the context of other imaging modalities used for the diagnosis of breast cancer. In addition to the relative insensitivity whole-body PET for small lesions, PET is not a realistic candidate for use in cancer screening of low-risk populations due to its high current cost per study. As FDG prices decline and dedicated instruments are developed that are less expensive, this could change, but it is unlikely that FDG-PET costs could drop to those of mammograms in the near future. This is in contrast to screening mammography, which has been shown to save lives and is the key method for breast cancer detection. Mammography is, however, of somewhat less value for detecting cancers in women with dense breasts (especially younger women), assessing the breast after biopsy, characterizing lesions as malignant or benign, determining whether disease is unifocal or multifocal, and predicting or assessing response to treatment. Mammography cannot determine whether the tumor is localized or metastatic. It is in these groups of patients that PET may have its greatest potential role in the assessment of primary lesions.
Detection of Regional and Systemic Metastatic Disease
Clinical studies have supported preclinical findings in rodents of high ratios of tumor tracer uptake to normal lymph node uptake, which suggested that FDG might permit detection of lymph node metastases. It was noted that when FDG was injected subcutaneously, there was intense
tracer uptake in the lymph nodes proximal to the injection site (49). In a preliminary study, Wahl et al. were able to correctly characterize 8 of 9 axillae with FDG-PET in the preoperative setting using tracer injection in the arm opposite to the side of the tumor (50). No false-positive findings occurred, but there was one false-negative result.
tracer uptake in the lymph nodes proximal to the injection site (49). In a preliminary study, Wahl et al. were able to correctly characterize 8 of 9 axillae with FDG-PET in the preoperative setting using tracer injection in the arm opposite to the side of the tumor (50). No false-positive findings occurred, but there was one false-negative result.
Several other reports of imaging axillary nodal metastases from breast cancer with PET have now appeared. In a report by Tse, using the whole-body PET technique, 11 of 14 axillae were accurately characterized as to the presence or absence of metastases (51). In 5 cases, there was uptake of FDG in the axillary nodal region, and in each instance there was tumor involvement of the axilla. In a prospective study of 20 patients with newly diagnosed breast cancer who underwent axillary lymph node dissections following PET scans, 9 of 10 patients with nodal metastases were correctly diagnosed, while 10 of 10 disease-free axillae did not show increased uptake (52).
Figure 45.1 shows a whole-body FDG-PET scan in a patient with a primary left breast cancer and palpable axillary lymph nodes. The PET scan confirms FDG uptake in the primary tumor and axillary metastases and also demonstrates high axillary and supraclavicular lymph node metastases, which were not clinically apparent. Figure 45.2 demonstrates the potential usefulness of fused PET-CT images for anatomic localization of focal uptake in the axillary or chest wall region.
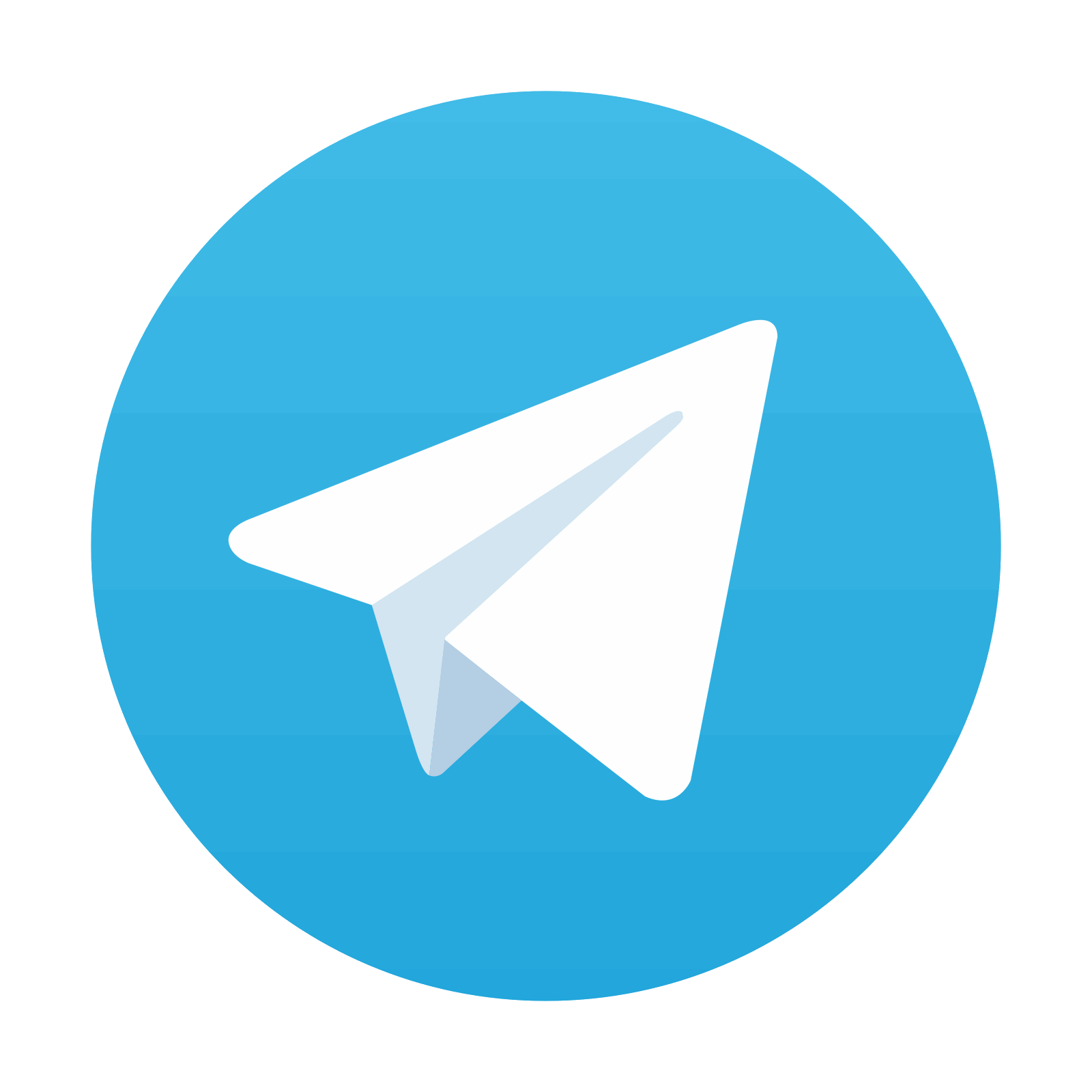
Stay updated, free articles. Join our Telegram channel

Full access? Get Clinical Tree
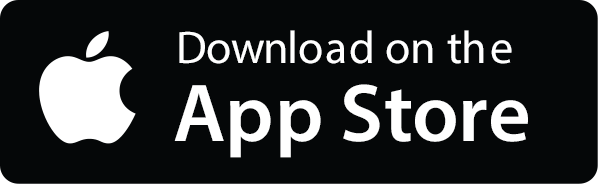
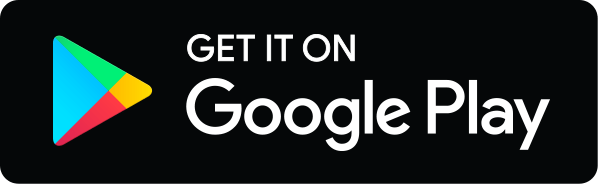