PET-CT and SPECT-CT of Other Gastrointestinal Tumors: Somatostatin Receptor–Positive and Gastrointestinal Stromal Tumors
Heiner Bihl
Gerald Antoch
Somatostatin receptor (SSTR)-positive tumors and gastrointestinal stromal tumors (GISTs) are relatively rare malignant tumors of the abdomen. SSTR-positive tumors typically are somewhat well differentiated and therefore are only amenable to fluorodeoxyglucose (FDG) positron emission tomography (PET) imaging in cases where dedifferentiation has occurred. However, with the introduction of somatostatin receptor analogs for single-photon emission computed tomography (SPECT) imaging, such as indium 111 [111In]pentetreotide, and PET imaging with gallium 68 (68Ga)-DOTATOC, functional imaging methods have become important in tumor staging (see Fig. 43.6) and follow-up. In the imaging of these tumors, it is rare that a head-on comparison of SPECT and PET imaging is possible. Mainly due to the better resolution of PET-CT compared with SPECT-CT, but also due to the favorable uptake kinetics of the PET tracers, PET-CT is the preferred method, as it can identify smaller lesions and is therefore more sensitive. However, the availability of 68Ga-DOTATOC is not yet widespread. It is unclear whether PET with fluorine 18 (18F)-labeled agents such as 18F-Dopa is more or less sensitive than 68Ga-DOTATOC imaging.
GISTs have been virtually unamenable to therapy other than surgery. With the introduction of tyrosine kinase inhibitors, a substantial fraction of these tumors have become treatable, and long-range remissions have occurred. PET plays an important role in therapy assessment, as a reduction of FDG uptake seems to correlate well with the therapy response of the tumor and its metastases. However, CT has a complementary role to play, as it has been reported to detect more GIST lesions than PET alone and will aid lesion localization with PET. Thus, FDG-PET-CT may be considered the modality of choice for GIST staging and for evaluating the therapy response of these tumors.
Somatostatin Receptor Positive Tumors
Somatostatin and the Somatostatin Receptors
The neuropeptide somatostatin was first identified in 1973 in ovine hypothalami (1) and has since been recognized as a major neurotransmitter and neuromodulator, with a mostly inhibitory capacity in that it regulates exocrine secretions, glandular secretions, neurotransmission (2), smooth muscle contractility, and absorption of nutrients. Of particular interest is the ability of somatostatin and somatostatin analogs to act as cytostatic agents in their effect on tumor cells (3), as evidenced in many animal tumor models and cultured tumor cell lines.
Human somatostatin, a 14-amino-acid peptide (somatostatin-14; Fig. 43.1A), appears in several organ systems, such as the central nervous system, the hypothalamopituitary system, the gastrointestinal tract, the exocrine and endocrine pancreas, and the immune system. The diverse actions of somatostatin are mediated through interaction with a family of different specific, high-affinity somatostatin receptors (SSTRs) located on the plasma membrane of the target cells. To date, five human SSTR subtypes (SSTR1 to SSTR5) have been cloned and partially characterized (4). All five SSTR subtypes bind the endogenous somatostatin-14 with high affinity (in the nanomolar range). However, the short biologic half-live of somatostatin in vivo (about 2 minutes) prevents its application in the clinic. To overcome this drawback, analogs of somatostatin-14 consisting of 8 amino acids were developed (5). These octapeptides (Fig. 43.1) exhibit a biological half-life on the order of several hours, and among them octreotide (Fig. 43.1B) is one of the clinically most relevant.
Although all five human SSTR subtypes bind somatostatin-14 with high affinity, there are differences in the binding affinities of the analogs; octreotide, for example, is bound with high affinity by the SSTR2 and SSTR5 receptor subtypes and with a modest affinity by SSTR3 but is not bound by subtypes SSTR1 and SSTR4.
Receptor-binding studies have identified SSTRs in the human brain as well as in numerous peripheral tissues, including the pituitary, pancreas, gut, and thyroid and the adrenal and immune systems, to name a few. The subtype most frequently expressed is usually SSTR2.
Neuroendocrine Tumors of the Gastroenteropancreatic System
Phenotypically, the cells of GEP-NETs belong to the system of disseminated neuroendocrine cells. This system is composed of cells that are scattered throughout the mucosa of the gastrointestinal tract or form the islets in the pancreas. These cells have many histologic similarities to neural cells such as secretory granules and the markers chromogranin A, synaptophysin, and neuron-specific enolase. These features led
to the designation “neuroendocrine cells.” They constitute the so-called diffuse endocrine system (8).
to the designation “neuroendocrine cells.” They constitute the so-called diffuse endocrine system (8).
Misunderstanding was perpetuated for the clinician by the application of different classifications and terminologies to the many varied types of neuroendocrine tumors of the gastrointestinal tract. In the past, pathologists called these tumors carcinoids because their histology is quite similar without special staining. This practice still is continued sometimes. In 1963, these carcinoids were subclassified (9) according to their embryologic site of origin as foregut carcinoids (lung, stomach, duodenum, biliary system, and pancreas), midgut carcinoids (small intestine, appendix, cecum, and proximal colon), and hindgut carcinoids (distal colon and rectum).
This classification was the first to emphasize clinicopathological differences between the tumor groups composing the gastroenteropancreatic neuroendocrine tumors but was not generally accepted by clinicians, because it proved too imprecise in distinguishing between the (biologically) different GEP-NET entities. Therefore, instead of “carcinoid,” the neutral terms “neuroendocrine tumor” and “neuroendocrine carcinoma” were chosen for use in the new World Health Organization (WHO) classification of 2000 (10). It relates histopathology to biological behavior (i.e. prognosis). Three major categories of GEP-NETs have been defined: (a) well-differentiated neuroendocrine tumors (benign or low-grade malignancy), (b) well-differentiated neuroendocrine carcinomas, and (c) poorly differentiated neuroendocrine carcinomas. The criteria on which categorization of the tumors is based are, for example, size, presence of metastases, and proliferation index. The proliferation index is determined by the percentage of cells staining positively with a monoclonal antibody directed against a nuclear antigen in proliferating cells (Ki-67/MIB-1) (greater than 2% indicates increased degree of malignancy).
The term “carcinoid” is not completely abandoned. For gastroenteric NETs, it is still used synonymously with the term “well-differentiated neuroendocrine tumor.” The term “malignant carcinoid” is still used synonymously with the term “well-differentiated neuroendocrine carcinoma” (11).
Somatostatin Receptor–Positive GI Tumors
GEP-NETs are among the tumors that most frequently express SSTRs with high density and homogeneous distribution (others include medullary thyroid cancer, small cell lung cancer, meningioma, medulloblastoma, glioma, breast cancer, lymphoma, and renal cell cancer). Pancreatic NETs (e.g., gastrinomas, glucagonomas, and VIPomas) and gut NETs (foregut, midgut, and hindgut NETs) usually express SSTRs in 80% to 100% of the cases. Insulinomas, however, have a lower incidence of SSTR expression (about 50%).
Among the five SSTR subtypes, the SSTR2 subtype is the most frequently expressed in GEP-NETs, while SSTR4 is rarely detected. It is well known that undifferentiated GEP-NETs express SSTRs less often (and, if expressed, in lower densities) than well-differentiated GEP-NETs.
Therapy of GEP-NETs
Management of GEP-NETs is primarily based on surgical removal of the primary lesion and the metastases (12), as these slow-growing tumors are only minimally responsive to systemic chemotherapy.
Neuroendocrine tumors of the gastrointestinal tract and pancreas may present with hormone-related symptoms or without hormonal symptoms (so-called nonfunctioning tumors). Therefore, the treatment might have different aims, such as to control excessive hormonal secretion or retard tumor growth. Surgery is generally considered as the first-line treatment for patients with localized disease, because it can be curative. In patients with metastatic disease, surgery is palliative, directed toward a reduction of the tumor burden and a consequent decrease in hormone secretion.
Cytotoxic treatment has been applied to a number of GEP-NETs, particularly those with high proliferation capacity, with minor beneficial effect.
Interferon alfa has been used for the treatment of classic midgut carcinoids for almost 2 decades and has generated biochemical and symptomatic improvement in about 50% of patients but significant tumor reduction in only 10% to 15%. Stabilization of the disease has been obtained in 60% to 80% of patients up to 3 to 4 years (13,14).
Nonradiolabeled somatostatin analogs have been used for the management of clinical symptoms in patients with GEP-NETs, and significant biochemical and symptomatic improvement has been obtained in 50% to 60% of patients, but tumor reduction has been seen in only 3% to 5%. (14). Beta particle–labeled somatostatin analogs are well-established radionuclide therapy modalities for GEP-NETs (15). Yttrium 90 (90Y)- and lutetium 177 (177Lu)-radiolabeled somatostatin analogs have been used for the treatment of GEP-NETs. The physical characteristics of 90Y and 177Lu suggest that 90Y-labeled analogs are more suitable for radiotherapy of larger tumors, 177Lu-labeled analogs more suitable for smaller tumors (16,17).
Imaging
In practice, imaging of GEP-NETs is routinely performed with standard imaging techniques such as ultrasonography, CT, and MRI. There is little difference in sensitivity between CT and MRI, although the former is probably superior for localizing the primary tumor and thoracic lesions, whereas the latter may be superior in characterizing liver lesions. However, it is occasionally difficult to visualize GEP-NETs with CT or MRI because such tumors are frequently small and can be disseminated over several organ systems. Visualization of SSTRs in GEP-NETs is therefore considered an alternative approach, provided that the density of SSTRs is high enough for this type of imaging.
Somatostatin-Receptor Imaging
As stated above, human somatostatin and SSTRs are found in the cells of neuroendocrine organs and in some non-neuroendocrine cells (6). In addition, tumors that arise from these tissues also contain SSTRs. GEP-NETs possess an especially high density of SSTRs (7,18).
In comparison to nontumoral tissue, tumor cells, especially GEP-NET cells, express a significant higher amount of SSTRs for somatostatin and their analogs. This observation provided the basis for the development of various radiolabeled somatostatin analogs as imaging agents. Since native somatostatin has only limited clinical usefulness due to its short biologic half-life (less than 3 minutes), synthetic somatostatin analogs were developed. Octreotide was the first such analog (5). Native somatostatin-14 binds with high affinity to all SSTR subtypes 1 to 5 (IC50 mean value = 0.93, 0.15, 0.56, 1.5, and 0.29 nmol, respectively), whereas octreotide binds with high affinity only to SSTR2 (IC50 = 0.38 nmol) and with a somewhat lower affinity to SSTR3 and SSTR5 (IC50 = 7.1 and 6.3 nmol, respectively).
Novel chelators, DTPA and DOTA, were designed for labeling with radiometals. The major step forward was achieved by the development of DOTA (19), a universal ligand for labeling with trivalent metal ions (indium, gallium, yttrium, lutetium), and the availability of new radiolabeled somatostatin analogs for scintigraphy (Fig. 43.1C), PET imaging (Fig. 43.1D), and radiopeptide therapy (Fig. 43.1E,F).
Somatostatin Receptor Scintigraphy with Indium 111 Pentetreotide (OctreoScan) SPECT/CT
In the late 1980s, Krenning et al. developed indium 111 [111In]pentetreotide (111In-diethlenetriamine penta-acetic [DTPA]-D-Phe1)-octreotide. It became the first commercially available radiopeptide (OctreoScan) containing a chelator-bound radiometal (20,21). Since this radiolabeled peptide exhibits a high affinity for the SSTR2 subtype, and because most GEP-NETs predominantly express the SSTR2 subtype, [111In]pentetreotide appeared to be a suitable tracer for the visualization of GEP-NETs, especially when combined with SPECT (Figs. 43.2 and 43.3).
The diagnostic use of [111In]pentetreotide (i.e., somatostatin-receptor scintigraphy [SRS]) has been extensively reviewed (22). It is commonsense that SRS is the “gold standard” for a cost-effective diagnosis of GEP-NETs, and its sensitivity ranges from 60% to 90%; it can be beneficial not only for diagnosis but also for staging and follow-up of patients with GEP-NETs. The variation observed in the sensitivity of SRS may be due to the loss of SSTRs in some tumors (SSTR2 expression in insulinomas is about 50%). SRS is usually performed following the guidelines of the European Society of Nuclear Medicine (23) and consists in general of the intravenous administration of 100 to 200 MBq of [111In]pentetreotide and subsequent planar whole-body and spot scans and SPECT scans 4 to 6 hours, 24 hours, and 48 hours postinjection. After intravenous administration, [111In]pentetreotide initially localizes in the thyroid, pituitary gland, liver, spleen, kidney, and bladder. It is rapidly cleared from the plasma and is excreted predominantly into the urine.
There have been a number of direct comparisons between conventional imaging methods and SRS in which SRS demonstrated substantially better performance and also the ability to detect more abnormal lesions (24,25,26). This was true in patients with an unknown location of primary tumor or metastases, with metastases confined to the liver, and with widespread neoplastic disease. Importantly, SRS had a positive impact in patient management: in the study by Lebtahi et al., SRS changed the surgical strategy in 25% of 160 patients (26). SRS also changed the staging classification of 24% of the patients, reflecting increased detection of metastatic lesions with SRS compared with conventional imaging.
Gibril et al. prospectively evaluated the effect of so-called false-positive findings detected by SRS on patient
management (25). Most of the false-positive images occurred in the imaging of physiologic conditions or of benign diseases where up-regulation of SSTRs could be anticipated (e.g., in thyroid, pituitary, and granulomatous lung disease).
management (25). Most of the false-positive images occurred in the imaging of physiologic conditions or of benign diseases where up-regulation of SSTRs could be anticipated (e.g., in thyroid, pituitary, and granulomatous lung disease).
Recent developments in helical CT have enhanced resolution beyond that of nonhelical CT. In one study with endocrine tumors of the gastrointestinal tract, helical CT correctly staged 92% of patients, compared with 75% staged correctly with SRS (27). This difference is probably due to the higher sensitivity of modern helical CT technology in liver lesion detection. SPECT increases the sensitivity in detecting SSTR-positive tissue, but SRS (planar together with SPECT) continues to be limited in its ability to determine the precise anatomic location of detected foci and often requires correlation with high-resolution imaging modalities for better topographic definition.
SPECT-CT (28,29) provides hardware-coregistered functional and anatomical images. When coupled with a low-dose CT, the images obtained are occasionally not diagnostic. Nevertheless, this system provides adequate information for the precise assessment of SPECT findings in most studies (Fig. 43.4), especially in patients with GEP-NETs. Pfannenberg et al. showed that SPECT could improve the specificity of SRS (30). Thirty-one lesions originally interpreted as benign or equivocal by SRS were reclassified as malignant, and 27 lesions originally interpreted as malignant by SPECT-CT or equivocal by SRS were reclassified as benign. Thus, the two imaging techniques proved to be synergistic and to complement each other.
Krausz et al., in a series of 72 patients with neuroendocrine tumors, reported that hybrid SPECT-CT imaging improved localization of SPECT-detected lesions in 23 out of 44 positive studies (31). These findings affected the diagnostic interpretation of SRS in 32% of the patients and induced changes in management in 14% of cases.
68Ga-DOTATOC PET-CT
There is only a limited number of clinical studies with 68Ga-DOTATOC PET in GEP-NETs. In a small series of four patients with GEP-NETs, Kowalski et al. evaluated 68Ga-DOTATOC PET in comparison with [111In]pentetreotide
SPECT. These authors stated that, like [111In]pentetreotide, 68Ga-DOTATOC showed the highest uptake in the spleen, followed by the kidney and the liver (32) (Fig. 43.5A). The highest uptake values in tumor tissue are found about 60 minutes postinjection, but for clinical interpretation, even 40 minutes postinjection are sufficient to depict all tumor manifestations due to the high tumor-to-background ratio.
SPECT. These authors stated that, like [111In]pentetreotide, 68Ga-DOTATOC showed the highest uptake in the spleen, followed by the kidney and the liver (32) (Fig. 43.5A). The highest uptake values in tumor tissue are found about 60 minutes postinjection, but for clinical interpretation, even 40 minutes postinjection are sufficient to depict all tumor manifestations due to the high tumor-to-background ratio.
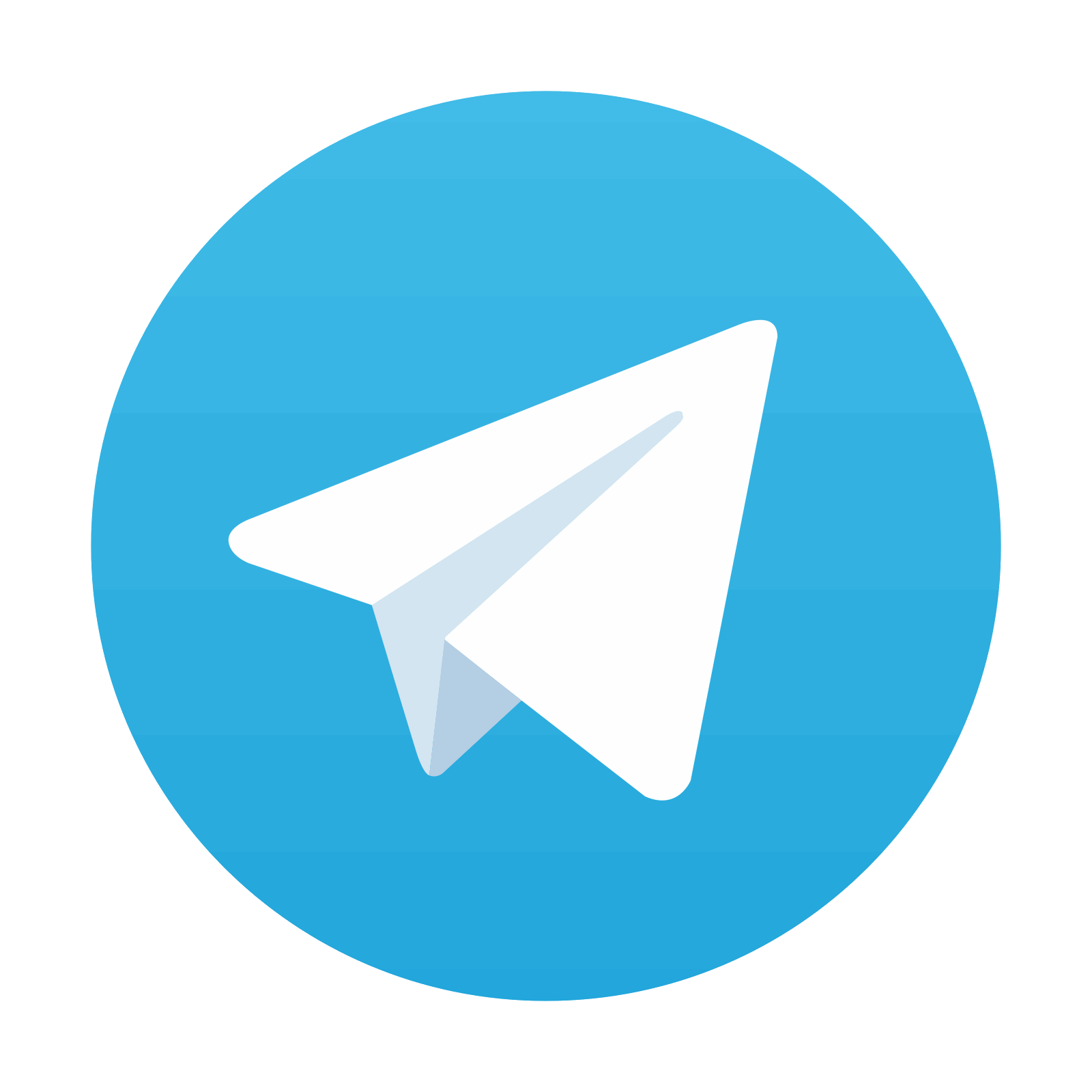
Stay updated, free articles. Join our Telegram channel

Full access? Get Clinical Tree
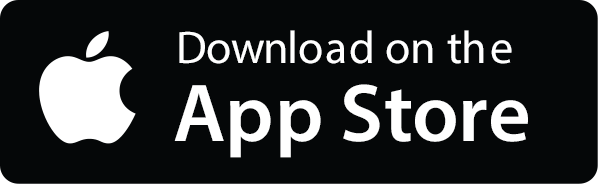
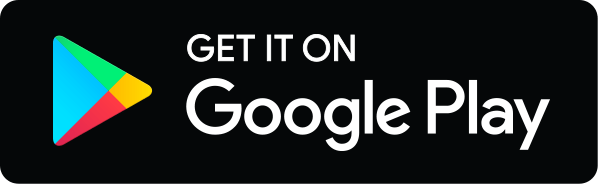
