Pneumothorax is the presence of air between the parietal and visceral pleura in the pleural cavity. It is caused most frequently by trauma or blunt or penetrating injury, which may be accidental or iatrogenic. If it is not caused by trauma, it is referred to as spontaneous; this may be primary (not associated with an underlying cause) or secondary to preexisting significant pulmonary disease. Pneumothorax may also occur after pneumomediastinum, with air-tracking into the pleural space.
Primary Spontaneous Pneumothorax
Etiology
A primary spontaneous pneumothorax occurs when air collects in the pleural space of a healthy individual without preceding trauma and without underlying lung disease. The etiology of primary spontaneous pneumothorax is a matter of debate. Traditionally, it is thought to result from the rupture of a small, thin-walled air-containing space (emphysema-like changes [ELCs]) within or deep to the visceral pleura into the pleural cavity. By convention ELCs are defined as blebs, when smaller than 1 cm in diameter, and bullae, which are larger than 1 cm in diameter ( Figs. 71.1 and 71.2 ). On pathology examination, pleural blebs are defined as emphysematous spaces occurring within the substance of the pleura ; bullae are in direct contact with the parenchyma. It is likely that most air-containing spaces associated with pneumothorax are, in fact, bullae. Smoking is an important risk factor for primary spontaneous pneumothorax. The lifetime risk for development of pneumothorax in smoking men is 12% compared with 0.1% in nonsmoking men. One study showed that smoking increased the relative risk of a first spontaneous pneumothorax approximately 9-fold among women and 22-fold among men, and that there is a striking dose-response relationship between smoking and the occurrence of spontaneous pneumothorax. Small airway inflammation (respiratory bronchiolitis) associated with smoking is likely responsible for the increased risk of pneumothorax in smokers. Cannabis smoking has also been linked with accelerated development of apical peripheral bullous disease, predisposing for pneumothorax, in the absence of parenchymal changes elsewhere in the lungs.
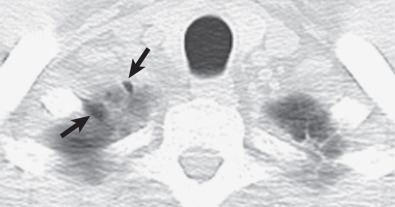
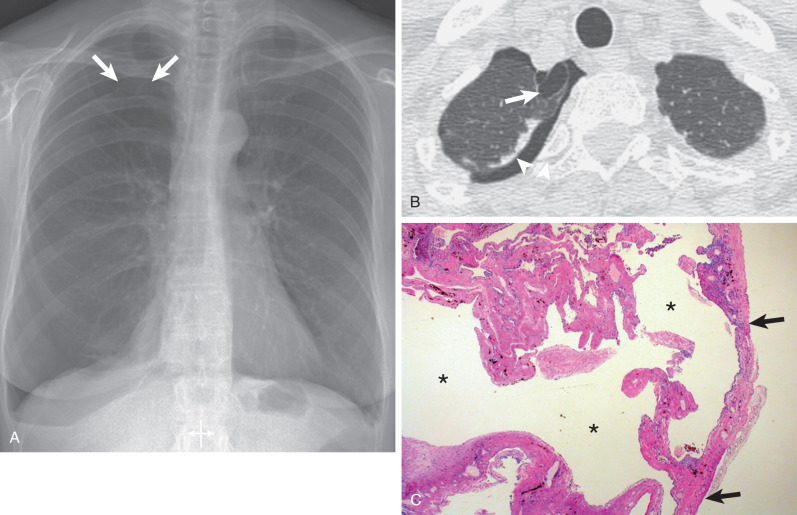
Prevalence and Epidemiology
Primary spontaneous pneumothorax occurs most commonly in patients between 18 and 40 years of age. The male-to-female ratio is about 4 : 1 to 5 : 1. The incidence of primary spontaneous pneumothorax is estimated to be approximately 7.4 to 18 per 100,000 per year for men and 1.2 to 6 per 100,000 per year for women. It has been observed that the typical person who presents with a primary spontaneous pneumothorax has an asthenic body habitus, being taller and thinner than the average person. An increased pleural pressure gradient between apex and base of lung is likely responsible for the increased risk in taller individuals.
Familial
More than 10% of patients with primary spontaneous pneumothorax report a family history of the disease, thought to be by autosomal-dominant inheritance. Other modes of inheritance have been suggested: more than one gene, with some cases inherited as an X-linked recessive disorder and others as an autosomal-dominant trait with incomplete penetrance. Recently, mutations in the gene encoding folliculin have been identified in individuals with familial spontaneous pneumothorax. Mutations in this gene were known previously to cause Birt-Hogg-Dubé syndrome ( Fig. 71.3 ), an autosomal-dominant inherited disease characterized by benign skin tumors, diverse types of renal cancer, pulmonary cysts, and spontaneous pneumothorax. The presence of thin-walled cysts in basilar and subpleural locations of the lung is a feature of this disease. The location of these cysts contrasts with the apical distribution of other more well-recognized causes of spontaneous pneumothorax, such as emphysematous bullae and idiopathic blebs. About 80% of patients with Birt-Hogg-Dubé syndrome manifest pulmonary cysts, and between 11% and 32% of patients develop pneumothorax. When large, the cysts may be multiseptated. A significant fraction of families with familial spontaneous pneumothorax have mutations in the folliculin gene; this should be considered a forme fruste of Birt-Hogg-Dubé syndrome.
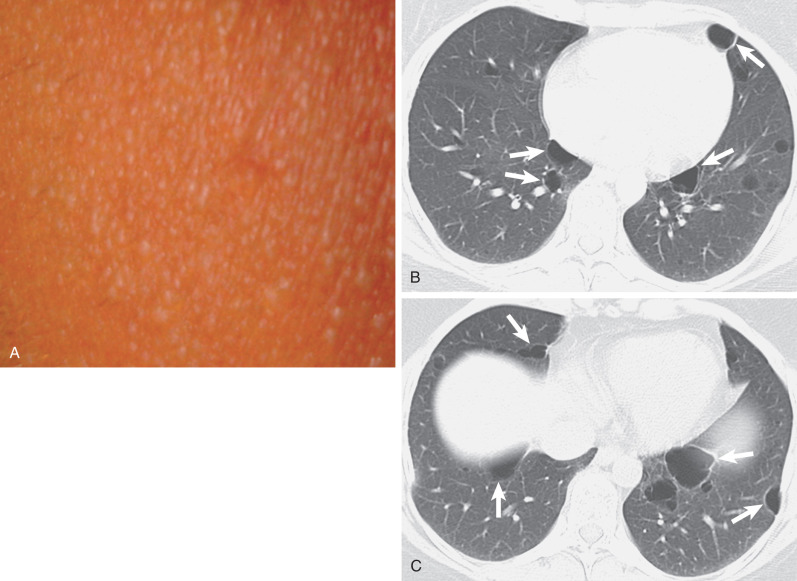
An intrinsic abnormality of connective tissue resulting in an increased tendency to bulla or bleb formation is also important. For example, spontaneous pneumothorax is a well-known complication in patients with Marfan syndrome—an autosomal-dominant disease with a frequency of spontaneous pneumothorax of 4.4%–11%, Ehlers-Danlos syndrome, homocystinuria, mitral valve prolapse, and α1-antitrypsin deficiency.
Pathophysiology
The cause of primary spontaneous pneumothorax is debatable. Although patients with primary spontaneous pneumothorax do not have clinically apparent lung disease, subpleural bullae are found in 76% to 100% of patients during video-assisted thoracoscopic surgery and in virtually all patients during thoracotomy. Even among nonsmokers with a history of pneumothorax, 81% have bullae, also called ELCs. Whereas it has generally been assumed that these bullae on the visceral pleura leak, causing the pneumothorax, there is a lack of evidence of actual rupture of the bullae at thoracoscopic surgery. Furthermore, simple surgical removal of the bullae does not prevent recurrence of spontaneous pneumothorax. It is more likely that small airway disease plays an important role, whereby inflammation-induced obstruction of the small airways increases alveolar pressure, which results in leaking of air into the lung interstitium. The air then moves to the hilum, causing pneumomediastinum (Hamman syndrome), or ruptures into the pleura, causing a pneumothorax. Some authors have suggested that the porosity of the visceral pleura is increased in patients with spontaneous pneumothorax.
Anatomy of the Pleura
The outer surface of the lung and the inner surface of the protective thoracic cage are covered by an elastic, serous, and lubricating membrane to form the pleural cavity. This is almost like inserting a sealed wet and stretchable plastic bag between the lung and the thoracic wall and diaphragm to decrease friction.
The anatomy of the pleura is complex. It covers the upper surface of the diaphragm and extends, in front, as low as the costal cartilage of the 7th rib; at the side of the chest, it extends to the lower border of the 10th rib on the left and to the upper border of the same rib on the right; and behind, it reaches as low as the 12th rib and sometimes even to the transverse process of the first lumbar vertebra.
The normal pleura is a thin translucent membrane and consists of five layers. These may be difficult to distinguish by light microscopy. These layers are (1) the mesothelium, flattened mesothelial cells joined primarily by tight junctions ( Fig. 71.4 ); (2) a thin layer of submesothelial connective tissue; (3) a superficial elastic tissue layer; (4) a second loose subpleural connective tissue layer rich in arteries, veins, nerves, and lymphatics; and finally, (5) a deep fibroelastic layer adherent to the underlying lung parenchyma, chest wall, diaphragm, or mediastinum.
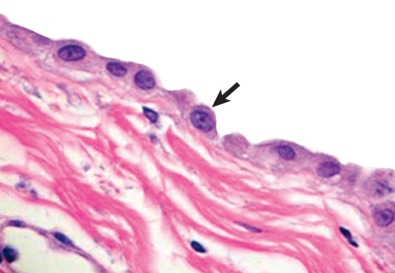
The lymphatic anatomy of the visceral and parietal pleura is important in the homeostasis of pleural fluid volume in the normal individual. The amount of fluid in the pleural cavity is regulated by the hydrostatic-osmotic pressure relationship and pleural-lymphatic drainage. Excess fluid, large particles, and cells in the pleural cavity are removed through preformed stomas assisted by respiratory movements. These naturally occurring pores exist in the caudal portions of the peripheral parietal and lower mediastinal parietal pleura. Most of the fluid that accumulates abnormally in the pleural space is derived from the lung through the visceral pleura and absorbed primarily through the parietal pleura.
The normal volume of pleural fluid in the adult is proportional to body weight (0.1–0.2 mL/kg). The normal pleural fluid has a protein concentration of approximately 1.5 g/dL. The pleural fluid has a few cells under normal conditions, including rare macrophages, mesothelial cells, and lymphocytes. The entire surface area of the pleura in an adult man is 2000 cm 2 .
The parietal pleura derives its blood supply from branches of the intercostal arteries. The mediastinal pleura is supplied by the pericardiophrenic artery, whereas the diaphragmatic parietal pleura derives its blood supply from the superior phrenic and musculophrenic arteries. The visceral pleura derives most of its blood supply from the bronchial arterial system.
Clinical Presentation
Most episodes of primary spontaneous pneumothorax occur while the patient is at rest. All patients have ipsilateral pleuritic chest pain or acute dyspnea. Chest pain may be minimal or severe. Symptoms usually resolve within 24 hours, even if the pneumothorax remains untreated and does not resolve. Patients with a small pneumothorax (<15% of the hemithorax) may have normal findings on physical examination. Patients with a larger pneumothorax may have decreased movement of the chest wall, a positive “coin test” result, diminished fremitus, or decreased to absent breath sounds on the affected side. The Hamman sign, initially reported in 1937 as a sign of pneumomediastinum, is now thought to be more likely associated with left-sided pneumothorax. The sign results from the presence of gas in close contact with the heart, characterized by a peculiar crunching, bubbling, popping, or crackling sound that varies with the phase of the cardiac cycle and is best heard in the left lateral decubitus position.
Manifestations of the Disease
Radiography
Upright Chest Radiograph
The most helpful sign is a thin pleural line (<1 mm thick) parallel to the chest wall ( Fig. 71.5 ), with no lung markings projecting beyond it. Because the lung is partly collapsed by the pneumothorax, it might be anticipated that its density would be increased and that this altered density, compared with that of the normal lung, should be sufficient to suggest the diagnosis; in fact, this is often not the case. First, as the lung progressively collapses with the increasing size of the pneumothorax, blood flow through it diminishes; therefore the ratio of air to tissue and blood is not altered, and the overall density of the collapsing lung is not changed. Second, lung density is reduced in the region of pneumothorax because of the presence of air both anterior and posterior to it.
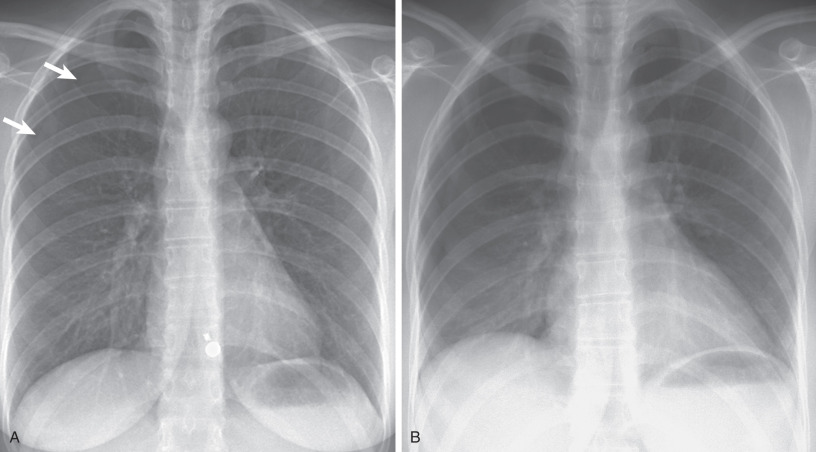
When pneumothorax is strongly suspected clinically but is not identified, gas in the pleural space may be detected on radiography by one of two methods: (1) chest radiograph in the erect position in full expiration, with the reason for this being that reducing the negative pleural pressure increases the relative size of the pleural space and thus allows easier detection of pneumothoraces, and (2) chest radiograph in the lateral decubitus position, with the involved side up, with the rationale being that air will be more visible over the lateral chest wall than at the apex. Compared with the erect chest radiograph, the lateral decubitus view is more sensitive. A study comparing inspiratory and expiratory upright films found that both were equally sensitive for pneumothorax detection. Given the limitations of expiratory films, inspiratory films are recommended as the initial examination of choice for pneumothorax detection.
Supine Chest Radiograph
In the supine patient, the main finding is the deep sulcus sign ( Fig. 71.6 ), a very deep radiolucent costophrenic sulcus. Note that the most nondependent portion of the pleural space in a supine patient is the inferior lateral hemithorax. It is important that the lateral costophrenic angles be included on the radiograph, as failure to diagnose pneumothorax may be life threatening because of the risk of tension. The supine view is much less sensitive for pneumothorax and grossly underestimates the true size of a pneumothorax. In one study of trauma patients, more than 50% of pneumothoraces were missed on the supine chest radiograph compared with computed tomography (CT). In a cadaver study the lateral decubitus film was most sensitive (88%), followed by the erect radiograph (59%), with the least sensitive being the supine view (37%).
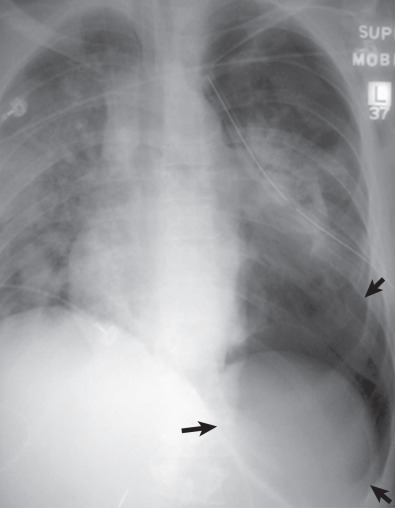
Other clues to the presence of a pneumothorax on a supine view are the following: relative hypolucency in the hypochondrial region or the entire hemithorax, depression of an ipsilateral hemidiaphragm, double-diaphragm appearance, sharp delineation of the cardiomediastinal and diaphragmatic borders, increased visualization of the mediastinal fat pads (floating cardiac pad sign), lobulated appearance of mediastinal fat pad, visible inferior border of the collapsed lower lobe or the heart, band of air in the minor fissure, and visible lateral edge of the right middle lobe.
In suspected pneumothorax, if only a single chest radiograph is taken, it should be done upright, on inspiration. When a pneumothorax is suspected in a supine patient, confirmation can be readily obtained with a lateral decubitus view. CT may also be useful and has been shown to identify 50% to 64% of occult pneumothoraces in the trauma setting.
Cause of Pneumothorax on the Chest Radiograph
Aside from the presence of a pneumothorax, the radiograph is often normal. However, apical blebs or bullae (see Fig. 71.2 ), apical scarring, and diffuse emphysema may be identified. In a study of 116 patients with surgically treated pneumothorax, parenchymal abnormalities were found in 68% and pleural thickening in 4%. The most common abnormalities were apical bullae, seen in 44% of patients. A study of chest radiographs of 100 patients presenting with first episode of primary spontaneous pneumothorax, followed for 57 months, revealed an increased likelihood of recurrence in the presence of certain radiographic abnormalities, including pleural thickening, blebs/bullae, pleural irregularities, and pleural adhesions. Another study found an increased likelihood of recurrence of pneumothorax after surgery in patients with a residual apical space on postoperative radiographs.
Computed Tomography
Several studies have shown that thin-section CT is a reliable method of detecting blebs and bullae in patients with spontaneous pneumothorax. The most common parenchymal abnormality associated with spontaneous pneumothorax is emphysema, which may be readily diagnosed on thin-section CT ( Fig. 71.7 ). If emphysema is not present, most patients will have an isolated bulla or bleb (see Fig. 71.2 ). In one study evidence of emphysema was seen on CT in 16 of 20 (80%) patients with spontaneous pneumothorax. In another investigation localized areas of emphysema were identified in 22 of 27 (81%) lifelong nonsmokers who had a previous spontaneous pneumothorax. The emphysema involved mainly the upper lobes and the peripheral rather than the central lung regions. The role of thin-section CT in predicting recurrence of pneumothorax is controversial. Some studies have found a significant relationship between the presence of blebs and bullae at CT after a first episode of primary spontaneous pneumothorax and the development of an ipsilateral recurrence or a contralateral episode of pneumothorax. Some researchers have questioned its role in preoperative assessment and predicting recurrence. A CT-based scoring system (dystrophic severity score) has been proposed based on size, number, and distribution of apical air-containing lesions. This score has been shown to predict ipsilateral recurrence and contralateral pneumothorax after the first episode in patients treated conservatively.
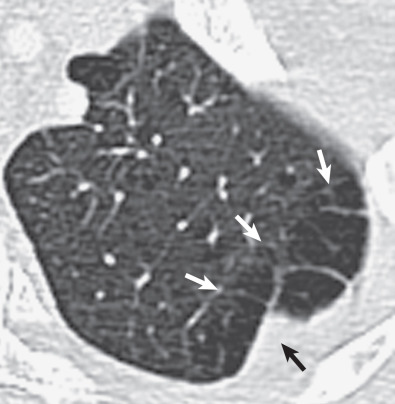
Ultrasonography
Ultrasonography is a sensitive and reliable method for diagnosis of a pneumothorax. Findings of pneumothorax on ultrasound have been well described, such as the absence of visceral pleural sliding movements during respiration—called disappearance of the “gliding sign,” absence of comet tail artifacts in the supine patient, absence of lung pulse—lung sliding resulting from transmitted cardiac pulsations, and identification of lung point—identification of the edge of pneumothorax by reappearance of the normal respiratory pattern (lung sliding and B lines). The presence of B lines (echogenic vertical lines moving with respiration, reaching the lower portion of field without fading), lung sliding, and lung pulse are helpful in ruling out pneumothorax. Among these factors, lung point is reported to be 100% specific when identified.
In a study of 183 patients after percutaneous needle biopsy, pneumothorax was identified in 46 patients (25%) by CT, in 44 by ultrasonography, and in 19 by chest radiography. The sensitivity of ultrasonography was 95.7%, and its specificity was 100%. Most investigations of pleural ultrasound otherwise have been limited to the trauma setting. Several meta-analyses comparing ultrasound with chest radiography, in the acute care setting, have shown the superiority of ultrasound for the diagnosis of pneumothorax. Ultrasound can also allow semiquantitative assessment of pneumothorax size by assessing the position of the lung point.
Differential Diagnosis
The thin white line of the visceral pleura in pneumothorax must be distinguished from the black line of a skin fold, which is accentuated by the Mach effect ( Fig. 71.8 ). Skin folds are usually seen to pass outside the chest cavity, are straight or only minimally curved, and usually do not run parallel to the chest wall as with a true visceral pleural line. The superolateral portion of the major fissures is a shadow identified in the upper hemithorax that appears as a curvilinear opacity of a curving edge, seen close to the lateral chest wall in proximity to the sixth posterior rib ( Fig. 71.9 ). This was reported in about 15% of cases in a study of 1068 normal subjects and is attributed to extrapleural fat intruding into the major fissure. A large bulla may sometimes be mistaken for a pneumothorax; it is critical to differentiate this from pneumothorax ( Fig. 71.10 ). Sometimes internal lung markings are visible in a bulla. A clue to bullous lesions is that those abutting the chest wall have a concave appearance. If there is any doubt, CT of the chest should be obtained. A huge bronchogenic cyst has also been mistaken for a pneumothorax. The scapula may sometimes be mistaken for a pneumothorax, but once considered, it can be traced in continuity with the rest of the bone. Hair, extraneous monitoring or support lines, and even the subcostal groove of the rib may mimic a pneumothorax. On occasion, pneumomediastinum may be confused with pneumothorax.
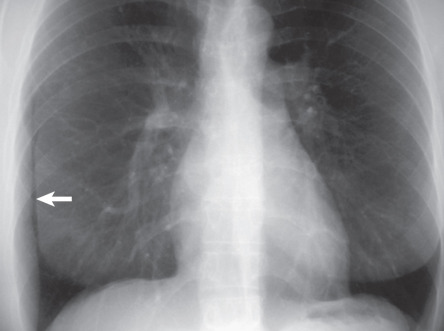
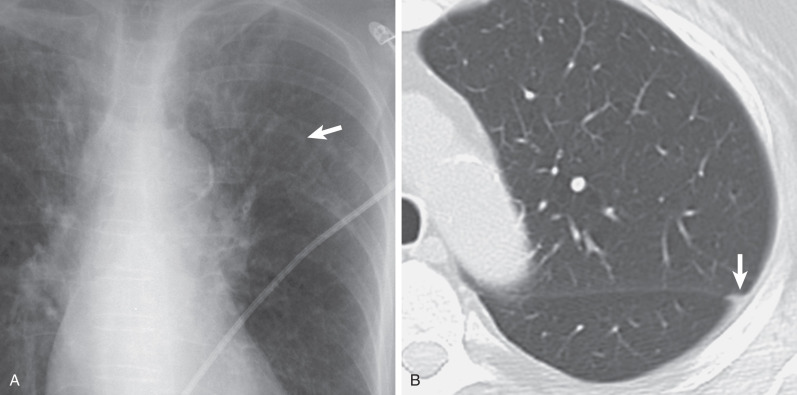
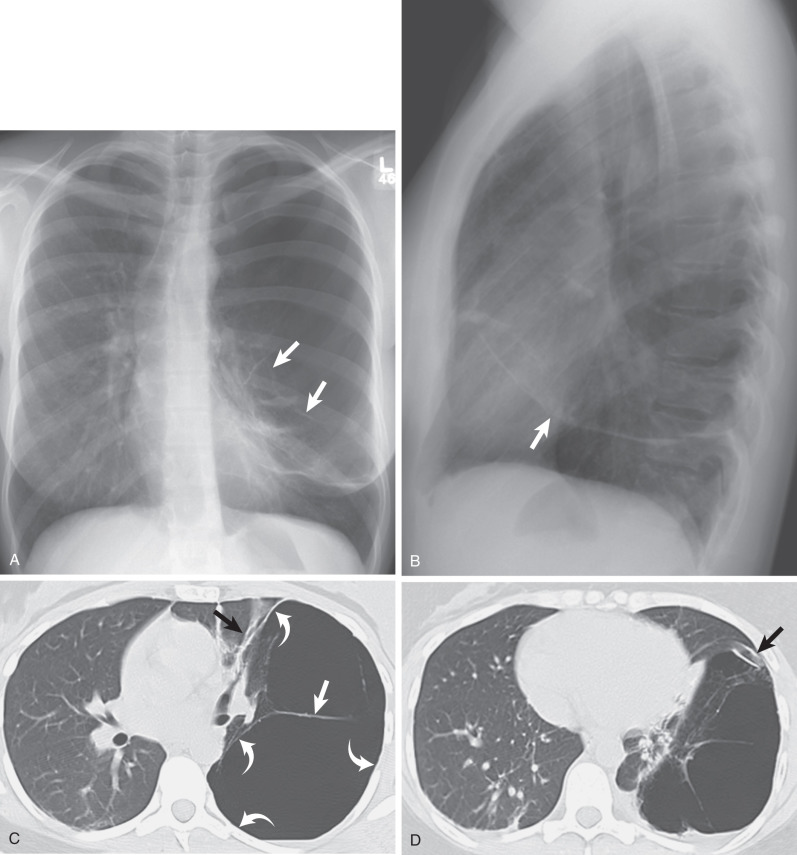
In the erect individual a pneumothorax is first evident near the apex of the chest (see Figs. 71.2 and 71.5 ); subpulmonic pneumothoraces have been reported occasionally ( Fig. 71.11 ). Free air under the diaphragm, colonic interposition (Chilaiditi syndrome) if it is right sided ( Fig. 71.12 ), and gastric distention on the left have been confused with a subpulmonic pneumothorax. Lateral decubitus views may help differentiate these entities from pneumothoraces.
- •
White visceral pleural line
- •
Absence of lung markings beyond white line of visceral pleura
- •
Deep sulcus sign (supine radiograph)
- •
Prominent mediastinal fat tags
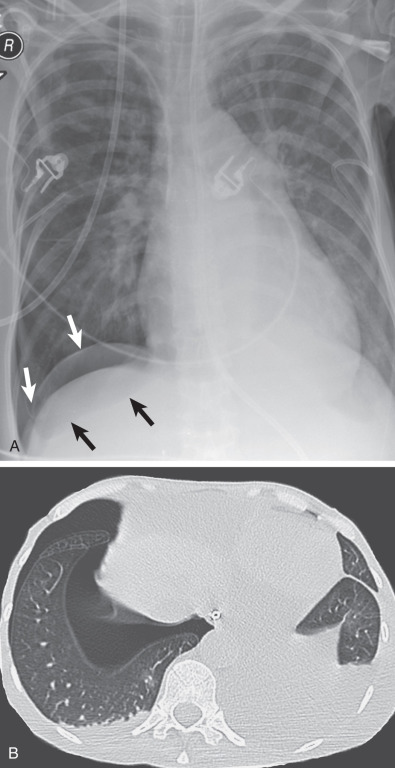
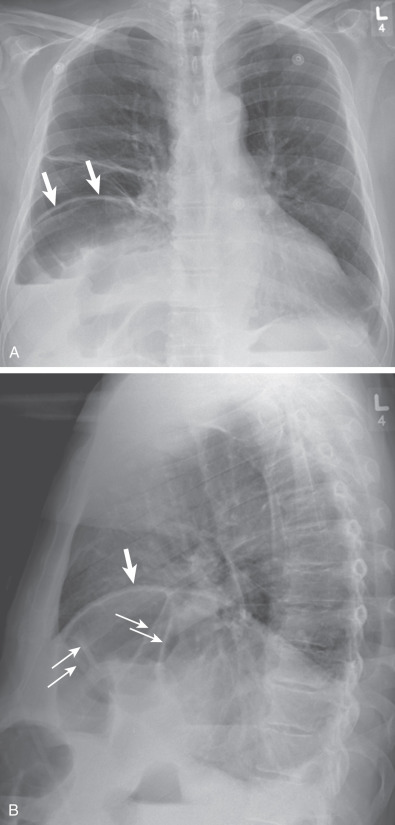
Tension Pneumothorax
Tension pneumothorax is a potentially fatal medical emergency that requires high degree of suspicion, immediate recognition, and treatment because affected patients rapidly become severely hypoxic and acidotic and often die. It is mostly seen in the setting of trauma, emergency resuscitation, or in ventilated patients. Its pathophysiology is trapping of inspired air in the pleural space, presumably on the basis of a bronchopleural ball-valve mechanism, leading to tension. Definitions for tension physiology differ in patients with a pneumothorax. Tension may be defined by mediastinal shift on the chest radiograph, by hemodynamic compromise, or by increased intrapleural pressure that remains positive throughout the respiratory cycle. The last definition is preferred as the more objective definition and clearly explains the hiss of air heard on thoracic needle decompression of tension pneumothorax. Clinical presentation depends on the ventilatory status of patients. Patients with unassisted breathing present with predominantly respiratory signs and symptoms and seldom with hemodynamic compromise. On the other hand, patients on ventilator-assisted breathing frequently present with sudden hemodynamic compromise and cardiac arrest.
Radiologic findings that may suggest tension physiology in the setting of pneumothorax include mediastinal shift, ipsilateral flattening of the heart border, hemidiaphragm depression, increased rib separation, and increased thoracic volume. Mediastinal shift is not a reliable finding because it may occur in the absence of tension physiology ( Fig. 71.13 ). Mediastinal mobility is observed more commonly in younger patients. The majority of patients with mediastinal shift do not have tension pneumothorax. In a study of 176 patients presenting to the emergency department, 30 patients had radiologic evidence of mediastinal shift, whereas only 2 had clinical signs of tension pneumothorax. Tachycardia, hypotension, and cyanosis should suggest a tension pneumothorax. The use of chest radiography for the diagnosis of tension pneumothorax can be problematic; it has been associated with a fourfold increase in mortality in two studies of ventilated patients who waited 30 minutes to 8 hours for the chest radiograph. If the patient is hemodynamically unstable (e.g., partial pressure of oxygen <92%, blood pressure <90 mm Hg, respiratory rate >10, decreased level of consciousness), immediate chest decompression must always precede a chest radiograph. However, in the awake, stable patient, a chest radiograph is the first test of choice.
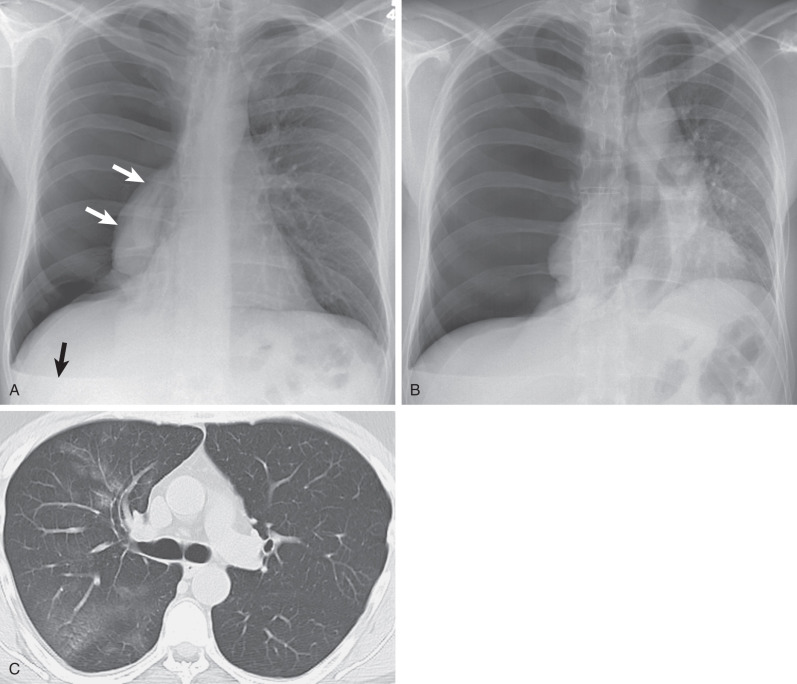
Tension pneumothorax is treated with high-concentration oxygen and emergency needle decompression, followed by chest tube insertion. Needle decompression is typically performed in the second to third intercostal space in the midclavicular line by a large-bore (10–16 gauge) needle. A recent meta-analysis recommends a catheter of at least 6.44 cm in length to ensure that 95% of the patients would have penetration of the pleural space at the site of needle decompression and therefore a successful procedure. Diagnosis is confirmed by egress of air through the needle in most of the respiratory cycle.
Secondary Pneumothorax
Etiology, Prevalence, and Epidemiology
The annual incidence of secondary spontaneous pneumothorax is 6.3 per 100,000 population in men and 2.0 per 100,000 in women. The incidence varies over time, as with the acquired immune deficiency syndrome (AIDS)-related Pneumocystis jirovecii pneumonia of the 1980s and 1990s. Many cases are associated with the formation of subpleural bullae or cystic spaces related to emphysema or interstitial fibrosis; rupture of one of these is likely to be the immediate cause of pneumothorax ( Fig. 71.14 ). As opposed to primary pneumothorax, the patient’s height and body habitus are not as important in the etiology of the secondary form. The most common cause of secondary pneumothorax is chronic obstructive pulmonary disease (COPD); with increasing age, the incidence of secondary spontaneous pneumothorax also increases. In addition to COPD and AIDS, many other underlying lung diseases, such as cystic fibrosis, aggressive or cavitating pneumonia, interstitial pulmonary fibrosis, and cystic lung diseases, such as pulmonary Langerhans cell histiocytosis and lymphangioleiomyomatosis, may cause secondary pneumothorax ( Box 71.1 ). In women, who are less commonly affected by secondary pneumothorax, specific causes are catamenial pneumothorax and pneumothorax from lymphangioleiomyomatosis.
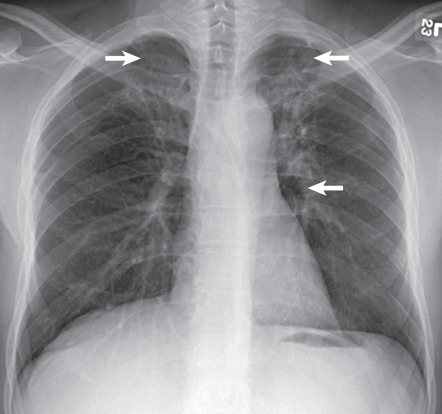
Developmental disease
Congenital pulmonary airway malformation
Connective tissue disease
Lymphangioleiomyomatosis
Tuberous sclerosis
Neurofibromatosis
Marfan syndrome
Ehlers-Danlos syndrome
Mitral valve prolapse
Infection
Fungal pneumonia (especially Pneumocystis jirovecii pneumonia in patients with acquired immune deficiency virus [AIDS])
Bacterial pneumonia
Miliary tuberculosis
Hydatid disease
Neoplasms
Primary lung cancer
Carcinoid tumor
Mesothelioma
Metastatic disease (carcinoma, sarcoma, germ cell tumors)
Drugs and toxins
Chemotherapy for malignant disease
Paraquat poisoning
Hyperbaric oxygen therapy
Radiation therapy
Aerosolized pentamidine therapy for AIDS
Immunologic disease
Granulomatosis with polyangiitis
Idiopathic pulmonary hemorrhage
Idiopathic pulmonary fibrosis
Langerhans cell histiocytosis
Sarcoidosis
Rheumatoid arthritis
Ankylosing spondylitis
Polymyositis or dermatomyositis
Scleroderma
Chronic obstructive pulmonary disease
Asthma
Emphysema
Cystic fibrosis
Pneumoconiosis
Silicoproteinosis
Berylliosis
Bauxite pneumoconiosis
Vascular disease
Pulmonary infarction
Metabolic disease
Pulmonary alveolar proteinosis
Intraabdominal disease
Gastropleural fistula
Colopleural fistula
Pneumothorax frequently is the presenting event that leads to the diagnosis of lymphangioleiomyomatosis in affected patients ( Fig. 71.15 ). The reported incidence of pneumothorax in women with lymphangioleiomyomatosis is 39% to 76% on the basis of a review of nine studies; the largest study showed an incidence of 66%. Many patients average 2.6 pneumothoraces before the diagnosis of lymphangioleiomyomatosis is established. In pulmonary Langerhans cell histiocytosis, pneumothorax precedes or complicates the clinical course of 25% of patients ( Fig. 71.16 ). Pneumothorax is a rare manifestation of sarcoidosis, usually occurring late in the course of the disease. There are sporadic reports of cases of pneumothorax as the initial manifestation of sarcoidosis. In these patients thoracotomy showed extensive pleural infiltration by noncaseating granulomas. High-resolution CT scans showed cavitated subpleural nodules and subpleural bullae in one case.
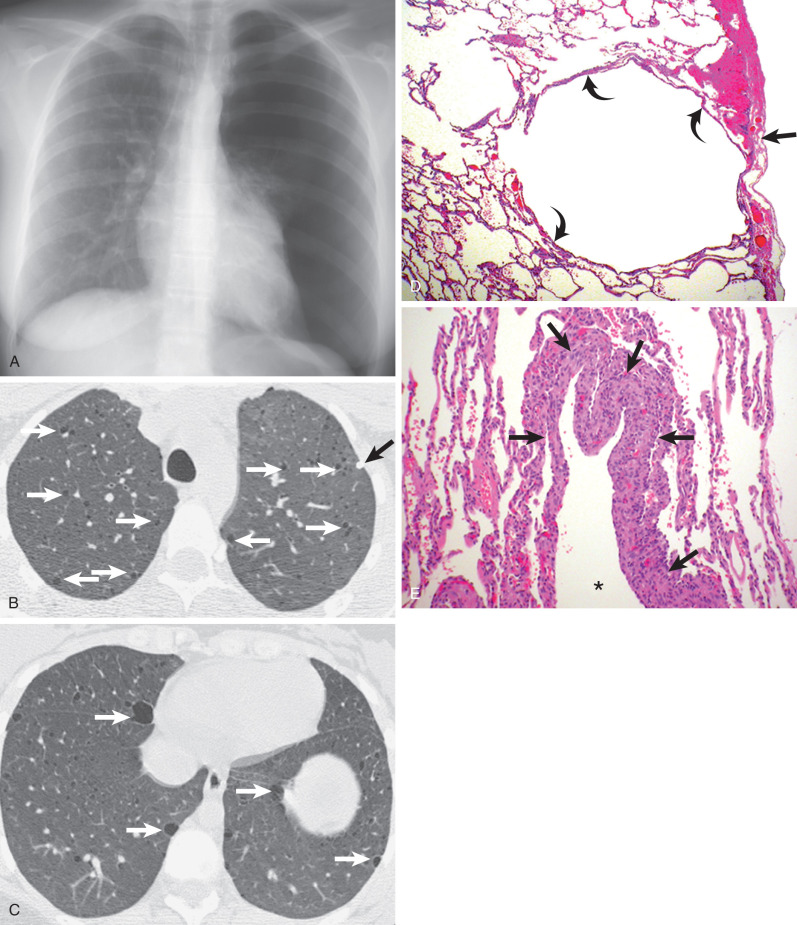
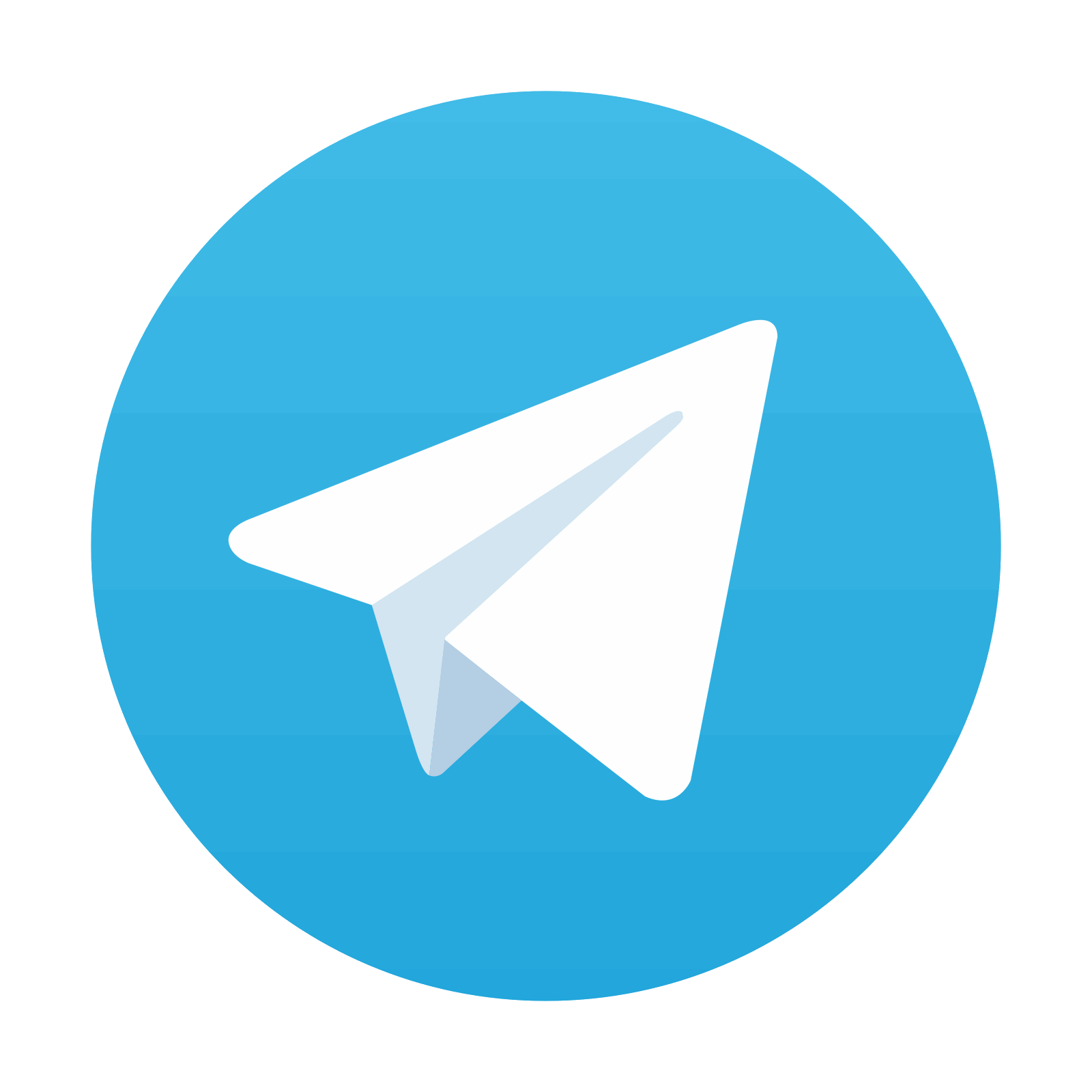
Stay updated, free articles. Join our Telegram channel

Full access? Get Clinical Tree
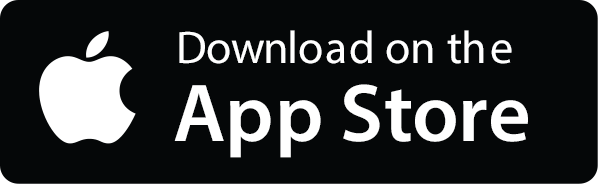
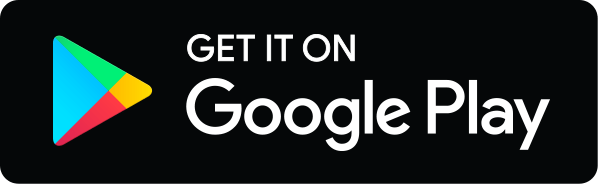
