Chapter Outline
Patient Preparation and Scanning
Positron emission tomography (PET) is a molecular imaging modality that has been increasingly used in clinical practice worldwide, especially in the oncologic population. It has gained widespread use for the diagnosis, staging, restaging, and monitoring treatment response of various malignancies. For years, computed tomography (CT), ultrasound (US), and magnetic resonance imaging (MRI) have been used for the detection and follow-up of tumors that grossly rely on the morphology or attenuation characteristics of pathologic tissue. PET has the ability to provide physiologic information by determining metabolic activity at a cellular level at an early phase, which is a shortcoming of cross-sectional imaging modalities. Thus, distinction of malignant versus benign lesions, detection of metabolic activity in organs that are not yet morphologically abnormal, and evaluation of the response of neoplasms to various treatments such as chemotherapy, radiation, or surgery have become possible with the use of PET.
PET imaging uses positron-emitting radionuclides produced in cyclotrons. Once the radiotracer is injected, the positron is emitted into the body. It travels a short distance, loses its energy, and interacts with electrons in tissue. This causes an annihilation reaction, which produces two 511-keV gamma rays that are emitted in opposite directions, approximately 180 degrees from each other. It is the localizing information provided by the coincidence of gamma photons, detected in opposite detectors in the ring of the PET scanner, that helps generate the image.
The most commonly used radiopharmaceutical in PET imaging is the glucose analogue fluorine-18-deoxyglucose (fluoro-2-deoxyglucose; 18 F-FDG). Just as with glucose, FDG is actively transported into the cell, mediated by glucose transporters (GLUTs). Once intracellular, FDG is phosphorylated. However, in contrast to glucose, it cannot be dephosphorylated and remains trapped in the cell. This allows sufficient time for imaging before FDG is metabolized. The use of FDG for oncologic imaging relies mainly on increased glucose consumption and overexpression of GLUT proteins in neoplastic cells.
FDG distribution occurs in normal structures such as the brain and the heart and is excreted in the urinary system. There are regions in the body with variable physiologic FDG uptake, such as the digestive and genitourinary tracts. As a consequence, differentiating physiologic uptake from pathologic uptake may at times prove to be a diagnostic challenge. Increased FDG uptake is not specific to malignancy and can also be seen in various inflammatory or infectious benign pathologic processes as a result of the underlying hypermetabolism. It is therefore important to be aware of the various pitfalls and physiologic and varying FDG distribution patterns in the body during the interpretation of imaging findings so as not to confuse them with malignancy.
PET not only offers qualitative information but also offers quantification by measurement of radioactivity in a given voxel. SUV (standardized uptake value) is a semiquantitative index of FDG metabolism that is calculated and normalized for injected radiotracer amount and body weight or body surface area. SUV measurements are obtained by placing a region of interest (ROI) or cursor on the area of interest. The values are calculated and displayed by the software of a PET workstation. When repeated PET scans are obtained in the same scanner, with the same acquisition parameters, SUV can serve as a means to assess response to therapy. Maximum or mean values of SUV can be obtained; however, maximum SUV values are more commonly used in clinical practice because of their reliability and reproducibility compared with mean values. If FDG were distributed evenly throughout the body, the SUV would be 1.0. Traditionally, an SUV maximum cutoff of 2.5 or greater has been used to differentiate between benign and malignant lesions. However, the organ in which the measurement is obtained and its comparison to background should be taken into account when determining abnormality of SUV values.
The functional information provided by the PET scan is complementary to the anatomic and morphologic data obtained by the CT scan. PET is limited in regard to accurate anatomic localization of detected abnormal activity, which is especially more of a challenge for abdominal and pelvic imaging. Initial PET scanners provided anatomic registration—that is, superimposition of FDG activity from the PET scan with the corresponding anatomic structure—from CT scans via separately acquired datasets fused with the aid of software. This method was easier and more accurate in organs such as the brain, but the same success was not achieved with body imaging, in which bowel peristalsis and motion from breathing caused frequent misregistration artifacts, complicating interpretation. Most of the current PET scans are hybrid units with built-in CT scans in the same hardware, providing immediately sequential acquisition and subsequent coregistration of data without moving the patient. Once the scan is completed, datasets from the PET and CT scans can be reviewed separately or as fused images. The first PET/CT scan became commercially available in 2001 and, since then, PET-only scanners have almost disappeared from the market. Currently, almost all manufacturers offer only hybrid PET/CT scanners.
Attenuation is the loss of the gamma photons emitted from the positron in the patient before they are detected by the scanner. Attenuation is a greater problem with PET imaging because both photons have to travel through the patient for greater distances compared with the traditional single-photon imaging used in nuclear medicine. Only approximately 10% of the photon pairs survive from a given source in the patient. As a result, attenuation correction is necessary to obtain high count, good-quality images. Earlier PET scans used external, long-lived radionuclide sources, such as germanium 68 ( Ge) or cesium 137, and measured transmission using the same detectors to provide an attenuation map of the patient’s body. However, obtaining transmission scans accounted for a significant portion of the scan time in PET-only units, and the length of the scan caused misalignment artifacts. Current PET/CT scans provide not only anatomic coregistration, but also attenuation correction, which reduces the statistical noise associated with Ge transmission maps. They also enable a significant scan time reduction, down from 45 to 60 minutes with the PET-alone units to 20 to 30 minutes, thus enhancing patient throughput.
Protocol for Scanning
An agreed-on universal protocol for PET/CT scanning does not yet exist, given that it is still a relatively new and developing modality. Institutions have developed their own modifications to existing protocols to fit their own preferences. There are three main options regarding the CT component while performing a PET scan—to obtain a low-dose CT scan for attenuation correction and image coregistration, a standard-dose CT scan, or a combined standard-dose CT scan with intravenous (IV) and oral contrast. The advantage of the latter options is that they provide diagnostic quality CT scans at the expense of increased radiation dose. Use of a fully diagnostic CT scan using IV contrast is especially important in the evaluation of vascular structures, organ enhancement, and bowel disease, as well as for distinguishing lymphadenopathy and masses from small bowel loops. In our institution, we first obtain a low-dose, noncontrast CT scan followed by the whole-body FDG PET emission scan. Subsequently, a diagnostic quality transmission CT scan is obtained using IV and oral contrast agents, which maximizes diagnostic information and minimizes the risk of artifacts.
In CT imaging, contrast agents are administered to enhance tissue contrast and differentiation and improve lesion localization and characterization. However, protocol modifications are necessary to avoid artifacts and ensure accurate attenuation correction on PET scans. Attenuation can be overestimated in PET in the presence of positive contrast agents. The resulting overestimation of attenuation may lead to potential artifacts, with images demonstrating increased FDG uptake in areas of high contrast concentration. IV contrast causes artifacts that are mainly limited to venous vessels close to the injected bolus, whereas positive oral contrast agents may cause an overestimation of FDG uptake up to 20%; this may be even more problematic when the patient has poor peristalsis or an obstruction causing accumulation of contrast in the bowel. The same applies to metallic implants in the body, which frequently causes attenuation correction artifacts. Non–attenuation-corrected images and the CT scan can serve as problem-solving tools in these cases, because artifacts will be found only on the attenuation-corrected data.
Although much of the misregistration caused by bowel peristalsis and patient motion has been alleviated by the use of CT, respiratory misregistration represents a persistent problem in precluding optimal image fusion. The PET scan is acquired over approximately 30 minutes during shallow respiration, whereas the attenuation-corrected CT datasets are acquired in seconds, during which the patient is instructed to breathe quietly or perform a breath-hold maneuver. The ideal breathing protocol remains to be determined. Several investigators have suggested that optimal fusion occurs with limited expiratory breath holding, whereas others have suggested fusion images of equal quality with shallow breathing.
Patient Preparation and Scanning
Our institutional protocol will be discussed here. Our patients are asked to fast for a minimum of 6 hours prior to PET/CT to optimize FDG uptake by tumors and minimize cardiac uptake. Instructions are given to avoid caffeinated beverages. However, water consumption and bladder emptying is encouraged, which aid in the urinary excretion of FDG and minimizes radiation dose to the bladder. FDG is a glucose analogue with a similar metabolism, as discussed earlier. Its uptake is affected by insulin and glucose levels in the blood. Hyperglycemia competes with and decreases FDG uptake by tumors; therefore, before the injection, a blood sugar measurement is obtained. The desired glucose level is below 200 mg/dL and the optimal level is below 150 mg/dL. Diabetic patients are instructed to avoid insulin injection within the 4 hours prior to the scan. In cases of higher glucose levels, measurement is repeated subsequent to light exercise, or sometimes even an injection of insulin is administered after an endocrine consultation.
After the IV injection of FDG (140 µCi/kg), patients are kept in a darkened room and encouraged to avoid excessive physical activity, as well as chewing and talking, until the time of the scan. Neutral oral contrast is given at the same time of injection for the subsequent diagnostic CT scan. We typically use two to three bottles (450 mL) of VoLumen (E-Z-EM, Lake Success, NY) for our neutral attenuation oral contrast agent. This is a low-density barium sulfate suspension that distends the bowel without increasing intraluminal attenuation. One hour after the administration of FDG, a static and emission PET scan is obtained. A transmission nonattenuation CT scan is obtained at the same time for attenuation correction at 120 kVp and 60 mAs during quiet breathing, with a slice thickness of 5 mm and overlap of 1.5 mm. A fully diagnostic helical CT scan using IV contrast is obtained subsequent to the emission PET scan during quiet breathing at approximately 140 kVp and 200 mAs, with a slice thickness and overlap of 5 mm.
Esophagus
Esophageal cancer is a leading cause of worldwide cancer mortality. The American Cancer Society (ACS) estimated 17,990 new diagnoses and 15,210 deaths from esophageal cancer in 2013. The lifetime risk of esophageal cancer in the United States is about 1 in 125 in men and about 1 in 435 in women. Complete resection of the tumor is the only potentially curative treatment in patients who do not have distant metastases or locally advanced disease. Depending on the stage of the disease, various treatment options exist, ranging from endoscopic resection to primary surgical esophagectomy to chemotherapy and radiotherapy. These treatment modalities lead to substantial morbidity and mortality, which makes accurate staging and pretreatment planning crucial. PET is widely used in clinical practice in the management of patients with esophageal cancer. In the United States, the Centers for Medicare & Medicaid Services (CMS) have approved the use of PET and PET/CT in the diagnosis, staging, and restaging of esophageal cancer.
In the proximal and midesophagus, squamous cell carcinoma predominates, whereas adenocarcinoma is mostly seen in the distal esophagus and esophagogastric junction. In recent years, there has been a dramatic increase in the incidence of adenocarcinoma of the esophagus, but the incidence of squamous carcinoma has remained relatively stable. Whether squamous or adenocarcinoma, PET scanning does not indicate a distinct difference between the two as a diagnostic tool. The most significant risk factor for adenocarcinoma is gastroesophageal reflux disease, whereas alcohol consumption and smoking are the two major risk factors for squamous cell carcinoma.
PET has limited value as a screening modality and problem-solving tool in disease exclusion prompted by abnormal findings in other diagnostic modalities because of its low specificity. A variety of physiologic or benign entities can cause uptake of FDG in the esophagus. Low-intensity, linear physiologic uptake has been described and is thought to represent swallowed secretions or smooth muscle activity. In cases of reflux esophagitis, mild to moderate intensity linear uptake can be seen predominantly in the distal esophagus. Radiation esophagitis can also lead to uptake of varying intensity, limited to the zone of radiation. Barrett’s esophagus, a precursor to adenocarcinoma, is columnar metaplasia of the distal esophagus. It is a complication of reflux esophagitis and has been associated with more intense uptake in the distal third of the esophagus. Other pitfalls include uptake in paraesophageal brown fat, atherosclerotic plaque in the adjacent aorta, and asymmetric uptake in the vocal cords. Recognizing these patterns because they are commonly seen pitfalls and correlating PET findings with the CT images to avoid misdiagnoses are important. However, when uptake is distinctly focal and intense, especially when a corresponding focal mass is seen on the CT scan, diagnosis is highly suspicious for esophageal cancer. In patients for whom the diagnosis is unknown or the PET scan is obtained for other reasons, it is reasonable to recommend endoscopic correlation.
Staging
Accurate disease staging is critical in choosing the appropriate treatment option and determining the prognosis of esophageal cancer. PET/CT has been widely used for staging of esophageal cancer, especially for the detection of distant metastasis and to determine whether patients are candidates for surgery. The clinical staging of esophageal cancer is assessed with the TNM staging system developed by the American Joint Committee on Cancer (AJCC) staging guidelines. The depth of tumor invasion through the layers of the esophagus determines the T (tumor) status of the disease, and the N (node) status of disease is determined by the presence (N1) or absence (N0) of regional lymph node metastasis. Detection of distant metastasis (M) determines the M status (M1 or M0) of disease. Lymph node metastasis to the cervical lymph nodes in upper esophageal cancer and to the celiac axis lymph nodes in lower esophageal cancer is designated as an M1a status. All other distant lymph node metastases fall into the M1b category. This categorization has prognostic value because patients with M1a disease have a worse prognosis compared with those with N1 disease.
Detection rates of PET for primary tumor is greater compared with CT, ranging from 83% to 96% in various studies. Patients with T1 (invasion of the lamina propria or submucosa) or T2 (invasion of the muscularis propria) disease primarily undergo surgical resection for curative treatment, whereas patients with T3 (invasion of the adventitia) or T4 (invasion of adjacent structures) disease usually require neoadjuvant chemotherapy or combined chemotherapy and radiation therapy. Although PET has a higher sensitivity in the detection rate of a primary tumor compared with CT, it is of limited value in T staging because it provides no data about the depth of invasion. PET is also limited in spatial resolution; thus, false-negative results occur in small tumors and in tumors that are well differentiated. In a study evaluating the usefulness of PET in superficial esophageal cancers, Little and colleagues found that PET was able to detect only 45% of in situ lesions (Tis) and 69% of T1 lesions and was unable to differentiate between the two. Endoscopic ultrasound (EUS), however, can accurately determine the T stage. In one meta-analysis, which included 49 studies and 2558 patients, the pooled sensitivity and specificity of EUS for diagnosing the T stage were 81.6% and 99.4% in T1, 81.4% and 96.3% in T2, 91.4% and 94.4% in T3, and 92.4% and 97.4% in T4 disease, respectively.
Various studies have not shown a substantial added benefit of PET for the detection of regional lymph nodes, for which EUS is the standard diagnostic tool. In a prospective study conducted by Flamen and associates, PET had a sensitivity of only 33% in the detection of regional lymph node metastases compared with 81% for endoscopic ultrasound. In the meta-analysis by Puli and co-workers, the sensitivity of EUS for diagnosing the N stage was 84.7%, which improved to 96.7% with the use of fine-needle aspiration (FNA). In another prospective study of 42 patients, Lerut and colleagues found that PET had a lower accuracy for the diagnosis of locoregional nodes compared with combined CT and EUS (48% vs. 69%), with a significantly lower sensitivity (22% vs. 83%). According to a meta-analysis of 12 studies conducted by van Westreenen and associates, the pooled sensitivity and specificity of PET for the detection of locoregional lymph node metastases were 51% and 84%, respectively. Unlike other tumors, locoregional lymph node metastases of esophageal cancer are in close proximity to the primary tumor. It is believed that intense FDG uptake of the primary tumor can obscure the uptake of these immediately adjacent lymph nodes, thus creating difficulty in the differentiation of a primary tumor from locoregional lymph node metastasis.
The additional value of PET in the evaluation of nodal status may lie in its high specificity. In the study by Flamen and co-workers, the specificity of PET was 89% compared with 67% with EUS. In another prospective study by Kato and colleagues, PET had an incremental value over CT in the initial staging of esophageal carcinoma in 14% of patients by accurately showing nodal metastasis in six patients with no positive CT findings and absence of disease in six other patients with positive CT findings. With PET/CT, anatomic and morphologic data obtained from CT are combined with functional information obtained from PET, thus improving detection rates of metastases. In esophageal cancer, metastatic involvement can be seen by histologic analysis in small lymph nodes and, conversely, benign causes of lymph node enlargement are commonly seen in the mediastinum. A morphologically abnormal or enlarged lymph node may show no FDG uptake; conversely, a small, normal-appearing lymph node may demonstrate FDG uptake. In this sense, PET and CT are supplemental to one another. When PET/CT is compared with PET in the assessment of locoregional lymph node metastasis, Yuan and colleagues showed an improved sensitivity of 94%, accuracy of 92%, and negative predictive value of 92% with PET/CT compared with 82%, 87%, and 86%, respectively, with PET only.
The current sensitivity rates in detection of locoregional lymph nodes with PET deem its use for the classification of the N stage of disease not sufficient for accurate presurgical planning. A multimodality, multidisciplinary approach using PET, CT, and EUS should be followed for accurate staging of nodal status in patients with esophageal cancer.
The primary role of PET in the management of esophageal cancer is in its value for detecting distant lymph node or solid organ metastasis ( Fig. 7-1 ). Although patients with M1a disease may benefit from neoadjuvant chemoradiation, radical surgery is contraindicated in patients with M1b disease. Patients with M1b disease are only offered palliative treatments and systemic chemotherapy. PET is very useful for the detection of common sites of distant nodal metastases, such as cervical lymph node metastases in upper esophageal cancer and celiac axis lymph node metastases in lower esophageal cancer. In the study by Lerut and associates, the accuracy and specificity of FDG-PET for distant nodal metastasis (M1a) was significantly higher compared with the combined use of CT and EUS (86% vs. 62% and 90% vs. 69%, respectively). In the same study, FDG-PET scanning correctly upstaged 12% of the patients.
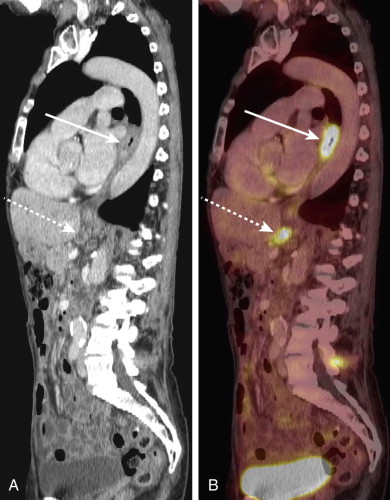
The most common solid organ metastasis of esophageal cancer is to the liver and lungs, followed by bone. Metastatic disease is present in 20% to 30% of patients with esophageal cancer at initial presentation. PET has shown to be more accurate and sensitive for the assessment of distant disease compared with CT and EUS. The meta-analysis conducted by van Westreenen and co-workers demonstrated a pooled sensitivity and specificity of 67% and 97%, respectively, for detection of distant metastases by PET. PET/CT has been shown to prevent surgery by upstaging disease via identifying distant metastases not seen by CT in approximately 14% (5 of 36) of patients in a study by Flanagan and colleagues and in 28% (7 of 25) of patients in another study by Rankin and associates. Heeren and co-workers found that PET upstaged 15 patients (15 of 74; 20%) correctly as M1 disease, missed by CT and EUS, and correctly downstaged 4 patients (5%) from M1 to M0 disease, with an overall incremental value of 25%.
Restaging and Treatment Response
The accuracy of CT for the detection of recurrent disease or evaluation of treatment response is limited because of the difficulty of differentiating post-treatment changes of inflammation and fibrosis from viable tumor. Morphologic changes do not always reflect tumor response because tumor size may remain stable, despite the viable tumor cells. Guo and colleagues evaluated PET/CT for disease recurrence and divided anatomic sites of recurrence to local, regional, and distant. The study demonstrated sensitivity values of 96.9%, 85.9%, and 90.5% and specificity values of 50%, 92.2%, and 89.9%, respectively. PET/CT was of low specificity for the evaluation of local recurrence. In the perianastomotic region, EUS is much more sensitive and specific for the detection of recurrence ; however, in the post-treatment patient, the use of endoscopy may be limited because of scarring, inflammation, postradiation esophagitis, or luminal stenosis in the anastomotic region. Therefore, just as with initial staging, a multimodality approach should be applied for restaging esophageal cancer following treatment.
In a meta-analysis by Westerterp and associates comparing the accuracy of CT, EUS, and PET in the assessment of response to neoadjuvant treatment, the maximum joint sensitivity and specificity values for CT, PET, and EUS were 54%, 85%, and 86%, respectively. PET and EUS had equivalent accuracy, but the invasive nature and limitations of EUS in the post-treated esophagus were noted. EUS was not feasible in 6% and suboptimal in 14% of patients.
Another study conducted by Cerfolio and co-workers investigated the accuracy of PET/CT, EUS with FNA, and CT for restaging following neoadjuvant chemotherapy and found that PET/CT accurately predicted complete response in 89% of patients compared with 67% with EUS-FNA and 71% with CT. The accuracy for detecting nodal disease was also superior with PET/CT, 93%, compared with 78% each with EUS-FNA and CT.
Response rates to neoadjuvant treatment are variable with esophageal cancer. Favorable response rates have ranged from 16% to 31% of patients in several published series. Patients who do not respond to neoadjuvant treatment may have a worse prognosis because of adverse effects of treatment or delay in surgery. Therefore it is crucial to have a test to select the pool of responders accurately and individualize treatment accordingly. PET has been widely used for this purpose.
Many studies have shown a strong correlation of FDG uptake with pathologic stage and survival in esophageal cancer. This has led to various studies investigating the use of the standardized uptake value (SUV) in determining the metabolic response to neoadjuvant treatment.
Ott and colleagues divided patients based on a predetermined rate of SUV reduction before and after neoadjuvant chemotherapy and classified them into metabolic responders and nonresponders in a prospective study of 65 patients. They found that metabolic response significantly correlated with pathologic response and median survival.
The Municon trial was a prospective study by Lordick and associates, which included 119 patients undergoing neoadjuvant chemotherapy, using PET to assess metabolic response rates in guiding treatment of distal esophageal adenocarcinoma. On the basis of a cut-off of 35% reduction in the maximum SUV (SUV max), patients were divided into metabolic responders and nonresponders following neoadjuvant chemotherapy. A major histologic response was seen in 58% of metabolic responders compared with 0% in nonresponders. Event-free and overall survival rates were also significantly better in the responder group at 29.7 versus 14.1 months in nonresponders. This study confirmed the benefit of PET in the metabolic evaluation of treatment response in patients receiving neoadjuvant chemotherapy and the feasibility of PET-guided treatment algorithms.
For years, the World Health Organization (WHO) criteria and Response Evaluation Criteria in Solid Tumors (RECIST) have been used to follow treatment response in solid tumors, which used anatomic metrics based on tumor size reduction. However, it is known that metabolic response usually precedes and is more accurate than morphologic response. With the introduction of molecularly targeted treatment options and the use of PET to assess the metabolic treatment response, these conventional evaluation methods have become of limited value. Recently, Wahl and co-workers introduced the PET Response Criteria in Solid Tumors (PERCIST), which is a new quantitative assessment of the metabolic response of tumors. Yanagawa and colleagues compared the use of RECIST to PERCIST and found that PERCIST was the strongest independent predictor of outcomes in patients receiving neoadjuvant chemotherapy for esophageal cancer.
PET/CT has also been used for radiation treatment planning. Calculating gross tumor volume in radiation therapy for the accurate delineation of tumor margins to achieve the best conformity index, the ratio between target tumor volume and irradiated volume, is necessary to treat the tumor successfully while keeping treatment-related complications to a minimum. The use of PET leads to improved accuracy of tumor volume calculation, with up to 20% changes in the calculated tumor volume compared with CT. In a study by Leong and associates, the use of CT only compared with PET caused a geographic miss of gross tumor volume, predominantly in the longitudinal direction, in 31% of patients. However, it is a developing method, and interobserver variability exists. More studies are needed before the use of PET/CT for radiotherapy planning gains widespread clinical use.
Conclusion
The main value of PET for the staging of esophageal cancer is its ability to detect distant lymph node and organ metastases otherwise not detected with other diagnostic modalities, thus altering the treatment in patients with more advanced disease status. Staging attempts should not be limited to PET because of its inherent limitations in T and N staging of disease. Ideal staging would use PET/CT for the detection of distant metastasis with precise anatomic localization and morphologic characterization provided by CT, supplemented by EUS for the improved detection of locoregional lymph nodes and assessment of depth of tumor invasion.
Another major strength of PET is its ability to evaluate treatment response more accurately by determining temporal changes in metabolic activity, thus providing the opportunity to identify those who do not respond to treatment and make alterations in their management. Standardization of scan timing, specific imaging protocols, and the establishment of PET treatment guidelines have yet to be determined. Further studies are needed to establish such standards.
Stomach
Gastric cancer is one of the most common primary gastrointestinal (GI) tract malignancies worldwide. It is often asymptomatic or causes nonspecific symptoms in its early stages. By the time symptoms occur, the cancer is often advanced and commonly metastatic, which accounts for its overall poor prognosis. As a result, gastric carcinoma, especially prevalent in Asian countries, is the second leading cause of cancer mortality, accounting for 600,000 deaths annually worldwide. Cure can only be achieved by gastric and adequate regional lymph node resection. However, the prognosis remains poor and recurrence rates are high, despite surgical resection and neoadjuvant therapy. As with other cancers, accurate preoperative staging is crucial in determining the most appropriate treatment option and whether curative surgery should be attempted. The main use of PET in gastric cancer is primarily for the detection of distant metastases. It has limited use in the primary diagnosis and detection of lymph node status.
When interpreting PET images for gastric carcinoma or for incidental findings in studies performed for other causes, it is helpful to keep several pitfalls in mind to avoid misdiagnoses. Several benign inflammatory or infectious causes, such as gastritis secondary to medications or Helicobacter pylori infection, may frequently cause nonspecific, diffuse FDG uptake of the stomach. This may challenge interpretation because this pattern of FDG uptake can also be seen in infiltrative primary or metastatic adenocarcinoma and lymphoma. Physiologic uptake in a nondistended stomach with collapsed gastric folds may also accentuate background uptake; the use of water ingestion or low-density oral contrast to distend the stomach adequately before PET scanning is recommended by many to increase the accuracy of PET. In these cases, clinical correlation for the patient’s symptoms of gastritis, recent endoscopy, or underlying primary malignancy may be helpful. In patients with unexplained diffuse or focal, moderate- to high-intensity uptake, correlation with endoscopy should be recommended.
Gastric cancer is staged by the standard TNM classification according to the depth of tumor invasion through the gastric wall (T), status and number of lymph nodes (N), and presence of metastasis (M). Gastric cancer usually occurs at the gastroesophageal junction; the most common histologic type is adenocarcinoma (>90%) followed by less common types such as mucosa-associated lymphoid tissue (MALT) lymphoma, carcinoid tumor, and sarcomas. Currently, no routine screening programs exist in the United States for the prevention or detection of gastric cancer. Prompted by suspected clinical or imaging findings, the diagnosis is usually made with endoscopy, along with tissue sampling.
CT is the most commonly used modality for more locally advanced disease to stage and assess the extent of the primary tumor, to evaluate for spread to locoregional lymph nodes, and at the same time to determine metastatic status. CT provides a rapid overall assessment of local and distant disease based on morphologic changes and tissue enhancement patterns. However, the use of CT, as for other cancers, is limited in the detection of nonenlarged local lymph nodes that may harbor tumor cells and has limited specificity because of benign entities that may cause lymph node enlargement. CT may also be limited for the detection of peritoneal and hematogenous metastases. Unfortunately, approximately 23% of patients who are otherwise not suspected to have metastases with conventional imaging modalities are found to have metastatic disease during surgery, precluding curative intent. This has led several investigators to evaluate the value of PET/CT for detecting local and distant disease preoperatively.
In early gastric cancer, EUS can accurately T-stage mucosal and submucosal lesions, which are generally considered endoscopically resectable if limited to the gastric wall. According to a study by Xi and co-workers, the overall accuracy of EUS for determination of the T stage was 80.0% and for T1, T2, T3, and T4 was 100%, 71.4%, 87.5% and 72.7%, respectively. EUS is more accurate for assessing the depth of wall invasion in early cancer compared with other imaging modalities, including CT, but is limited in the assessment of locally advanced disease and the detection of distant metastases. In a recent meta-analysis by Dassen and colleagues, the sensitivity of PET for the detection of the primary tumor ranged from 26% to 63% for early-stage gastric cancer and from 93% to 98% for locally advanced gastric cancer. Early-stage tumors are not detected at an equal sensitivity compared with more advanced tumors, which could be related to the difficulty in detecting focal uptake in a background of diffuse physiologic uptake or to the limited resolution of PET/CT. Certain types of gastric cancers, such as MALT lymphoma (MALToma), mucinous carcinoma, and signet ring cell carcinoma, are also known to have low levels of FDG uptake, with limited sensitivity values. PET should not be used in the work-up of these patients. Kim and associates have shown that signet ring cell gastric carcinoma has the lowest sensitivity for the detection on PET, 15%, whereas other types of gastric cancer have moderate sensitivity levels ranging from 30% to 71%. According to a study by Stahl and co-workers, the sensitivity of PET also differs according to the histologic type of cancer, as described by the Lauren system, with a detection rate of 83% for the intestinal type versus 41% for the diffuse type. Therefore it can be concluded that PET is of limited value in the detection and T staging of gastric cancer.
In a study by Chen and colleagues assessing the usefulness of PET for the preoperative staging of gastric carcinoma, the amount of FDG uptake correlated with macroscopic type, tumor size, lymph node metastasis, histologic type, and TNM stage of the tumor. PET had an accuracy similar to that of CT for diagnosing local and distant lymph node metastases as well as peritoneal status; however, in assessing local lymph node status, PET had a higher specificity than CT (92% vs. 62%). Moreover, PET provided additional diagnostic value in 10 of 68 (15%) of patients by upstaging 4 (6%) and downstaging 6 (9%) patients. In a study by Namikawa and associates, the sensitivity, specificity, and accuracy of PET/CT in assessing regional lymph node metastasis were 64.5%, 85.7%, and 71.1%, respectively. In another prospective study by Kim and co-workers, which included 73 patients with gastric cancer, the sensitivity, specificity, positive predictive value, and negative predictive value of PET for detecting lymph node metastases were 40%, 95%, 91%, and 56% respectively. Currently, PET is considered to have low sensitivity and accuracy but high specificity for the detection of lymph node metastases. This is in part related to the low spatial resolution of PET and the difficulty in distinguishing uptake of the primary tumor or background physiologic uptake from the adjacent small perigastric lymph nodes, as discussed earlier for esophageal cancer. The benefit of PET over CT could be in its high specificity and positive predictive values for the assessment of locoregional lymph nodes as well as a theoretic increased accuracy for the detection of distant lymph node metastases (e.g., those in the retroperitoneum). To date, there have not been many studies comparing PET/CT to CT or PET alone for the evaluation of locoregional lymph nodes in gastric cancer. The added benefit of hybrid imaging with PET/CT has been well demonstrated in the preoperative evaluation of esophageal cancer and may have a role and added benefit for gastric cancer.
Gastric cancer usually metastasizes to the liver, lungs, adrenal glands, and ovaries. The major advantage of PET is in the detection of these distant solid organ metastases, which may be metabolically active much sooner than they become morphologically apparent on CT. In one series, the overall sensitivity and specificity of PET to detect distant metastases was found to be 71% and 74%, respectively, with high values for liver and lung metastases but lower values for malignant ascites and malignant pleural effusion. Both CT and PET have limited sensitivity in the detection of peritoneal carcinomatosis, which is frequently diagnosed intraoperatively. Diffuse peritoneal FDG uptake or uptake in gross tumor deposits of omental caking can be seen; however, diffuse, small, nodular peritoneal deposits usually fall below the resolution of PET, although they can sometimes be detected by CT. Despite the added benefit of detecting distant unsuspected metastases in some cases with a high specificity, the added value of PET/CT in the diagnosis and work-up of patients with gastric cancer has yet to be determined.
PET/CT is useful in the detection of recurrence subsequent to surgical resection and in the assessment of treatment response to preoperative chemotherapy. PET can frequently differentiate treatment-related morphologic changes seen on CT from tumor recurrence. In a recent meta-analysis, Zou and Zhao analyzed results from 8 studies, which included a total of 500 patients, and found that the pooled sensitivity and specificity of PET/CT for the detection of local gastric cancer recurrence following surgery were 86% and 88%, respectively. Neoadjuvant therapy is frequently being used in the treatment of gastric cancer to improve survival with the subsequent surgical resection. Treatment response by CT is assessed with changes in tumor size following the RECIST criteria, which may sometimes be suboptimal. In some cases, the tumor may not reduce in size, despite no longer harboring tumor cells. PET can detect and separate treatment responders at an earlier phase than CT, thus reducing treatment-related morbidity and preventing delays in surgical treatment. In this regard, PET also provides prognostic information, because patients who do not respond to chemotherapy usually have more aggressive tumor biology.
There has been increasing interest recently in a new radiotracer, 18 F-fluorothymidine (FLT), in PET for the evaluation of gastric cancer, with the aim of acquiring more accurate diagnostic and staging information. Herrmann and associates have compared the feasibility of FLT-PET to FDG-PET and found FLT-PET to have a sensitivity of 100% in primary tumor detection compared with 69% with FDG-PET. These modalities are relatively new and warrant further investigation to gain acceptance in clinical practice.
To conclude, although PET may not have a role in the detection of the primary tumor and regional lymph nodes, it may provide valuable information with regard to increased specificity of findings, detection of distant metastases, and evaluation of treatment response. Fused PET/CT has been of additional value for various cancers, but its role in gastric cancer has yet to be fully determined. Initial results of studies investigating some new PET radiotracers appear promising.
Small Bowel
The most common small bowel cancer is adenocarcinoma which is mostly seen in the duodenum, followed by the jejunum, with the exception of patients with Crohn’s disease, in whom the most common location is the ileum. Metastases to the small bowel can also occur, usually seen with malignant melanoma, followed by breast and lung cancers. Less commonly seen tumors of the small bowel include neuroendocrine tumors (NETs), gastrointestinal stromal tumors (GISTs), and sarcomas ( Fig. 7-2 ). Some form of surgical treatment is offered with curative intent during early-stage cancer and for palliation, primarily to prevent obstruction in the more advanced stages. Neoadjuvant therapy is frequently used in more locally advanced disease, along with subsequent surgical resection, whereas systemic chemotherapy, which appears to provide a modest survival benefit, is offered to patients with more widespread disease. Given the variety of surgical and medical treatment options, accurate staging is crucial in deciding the optimal treatment to provide the most survival benefit to patients while minimizing unnecessary, treatment-related morbidity. Given the rarity of this type of cancer, the usefulness of PET/CT for the diagnosis and work-up of these patients has not been extensively investigated.
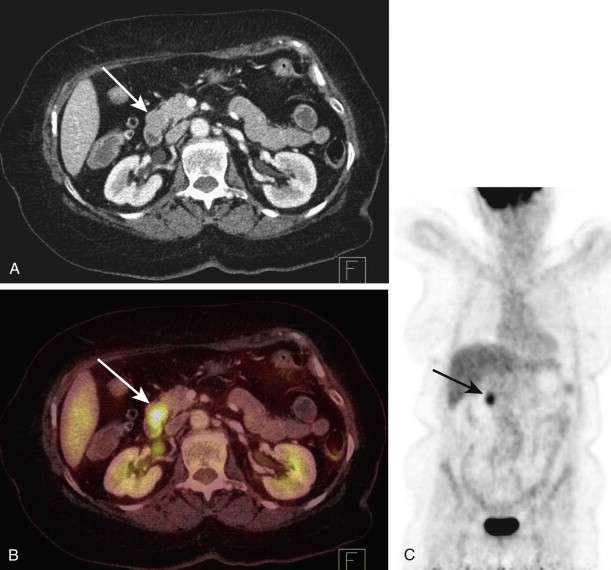
The most common appearance of small bowel carcinoma on PET/CT is focal or segmental wall thickening, with intense FDG uptake. However, potential pitfalls in interpretation exist and should be kept in mind to avoid misdiagnoses. The small bowel normally demonstrates diffuse, mildly heterogeneous FDG uptake, which may be more prominent in the pelvis, with multiple bowel loops overlying each other. FDG uptake in the small bowel uptake is nonspecific and can be seen with various infectious and inflammatory causes, including Crohn’s disease ( Fig. 7-3 ). Primary and metastatic small bowel malignancies, GISTs, and lymphomatous involvement of the small bowel can also show FDG uptake. Interpretation of the PET scans should be made in conjunction with CT findings to localize and characterize focal unexpected FDG uptake accurately, because a variety of bowel wall–thickening patterns have been well described in the CT literature.
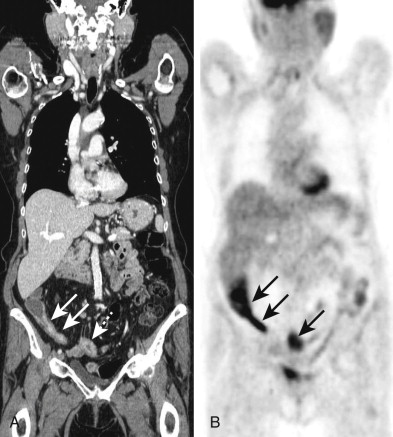
In patients suspected to have advanced disease, PET/CT would have the potential added benefit of staging the disease by identifying locoregional lymph node and distant metastases, evaluating treatment response, and restaging the disease subsequent to treatment. PET/CT has been used successfully in the imaging of small bowel tumors, as reported in various studies. PET/CT permits the detection and characterization of local and distant lymph node involvement and identification of unsuspected distant metastases, including the liver. Its added benefit has been proven in the work-up of patients with other GI tract malignancies, including esophageal, colorectal, and gastric cancers (see earlier). Currently, however, PET-CT’s accuracy and added benefit for detecting local and distant metastatic disease from small bowel adenocarcinoma have not yet been studied sufficiently to allow for their accurate determination.
GISTs are nonepithelial neoplasms typically arising in the bowel wall, mesentery, or omentum. Most GISTs occur in the stomach, followed by the small bowel, accounting for approximately 10% to 15% of small bowel cancers. All GISTs have malignant potential, with a worse prognosis in those that are of nongastric origin, large in size, and with a high mitotic index. PET has been used for detecting the malignant potential of GISTs by the use of FDG uptake and for assessing metastatic disease before surgery for accurate staging. However, its full potential for the work-up of patients with GISTs, as well as the indications and the selection of patients for which PET/CT would be useful, are yet to be determined.
NETs are typically not FDG-avid, so novel PET radiotracers such as 68 gallium-DOTATATE, 68 gallium-DOTA-NOC, and F- l -DOPA are being developed for applications in NET evaluation and have shown highly promising results. In a study by Haug and co-workers, 68 gallium-DOTATATE PET/CT identified NET in 29 of 36 patients and excluded the presence of a NET in 61 of 68 non-NET patients in a pool of 104 patients suspected to have NET. They reported a sensitivity of 81% and specificity of 90%. PET/CT had false-positive and false-negative results in 7 patients, with each indicating positive and negative predictive values of 81% and 90%, respectively, and an accuracy of 87%.
The use of PET/CT for the evaluation of small bowel tumors has yet to be determined, given the lack of sufficient data, which are restricted by the rarity of this type of cancer. Based on our experience, as well as extensive data obtained for other GISTs, PET/CT could be used as an adjunctive modality in the work-up of patients with small bowel tumors to detect local and advanced stages of the disease.
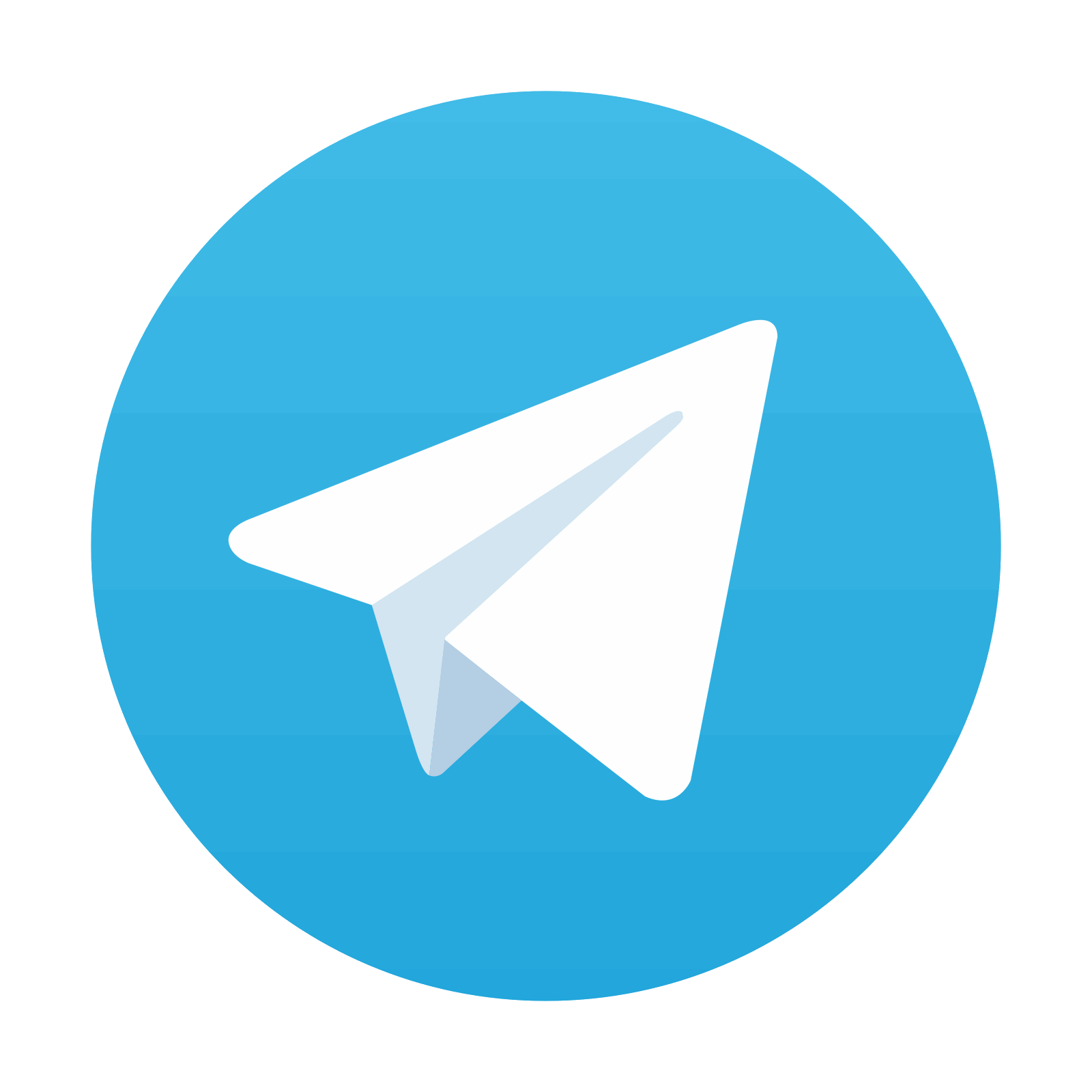
Stay updated, free articles. Join our Telegram channel

Full access? Get Clinical Tree
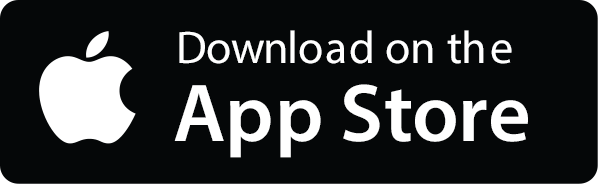
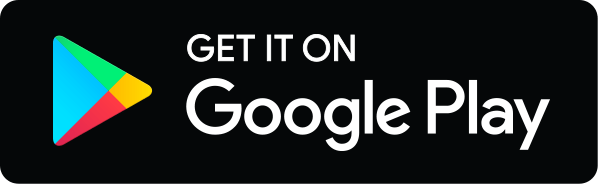