Functional magnetic resonance (fMR) imaging and diffusion MR tractography have emerged as valuable tools in the evaluation of verbal language in brain tumor patients, and have changed the way neurosurgeons look at patients with a mass in the dominant hemisphere. The techniques have obtained recognition as valuable presurgical clinical tools in the determination of hemispheric dominance and in the selection of candidates who may benefit from awake craniotomy. In the near future fMRI and diffusion MR Tractography may contribute to elucidate mechanisms of brain plasticity and may provide predictors of favorable postoperative clinical outcome. The functional anatomy of the language network and the role of fMR imaging and diffusion MR tractography in the evaluation of patients with a brain tumor are the focus of this article.
Cortical mapping with direct electrical stimulation mapping (ESM) during surgical craniotomy in awake and cooperative patients was introduced at the beginning of the twentieth century. In the early 1990s the refinement of intraoperative neurophysiologic monitoring, as well as the development of imaging-guided neuronavigational devices and of new functional and anatomic magnetic resonance (MR)-based mapping techniques set the stage for a renaissance of intraoperative ESM. Improvements in lesion localization, and in presurgical and intraoperative assessment of functional brain activity have increased the surgical accessibility of focal brain lesions seated in eloquent areas.
Modern neuroimaging methods provide morphologic, metabolic, and functional measurements that have the potential of guiding and refining surgical treatment. In particular, functional MR (fMR) imaging and diffusion MR tractography have the potential to map nodes and connections of several functional networks. If validated, modern imaging methods may determine hemispheric dominance, establish the relationship of a lesion to adjacent critical gray and white matter structures, and predict postoperative outcome. Neurosurgeons appreciate the value of having functional imaging maps available at their desk when planning a surgical procedure.
Mapping brain functions
Historical Perspective
The aim of understanding the functional organization of the human brain has motivated philosophers and scientists for centuries. The observation that a focal brain lesion may cause a behavioral or motor deficit was alluded to in an Egyptian papyrus that has been dated to roughly 2500 bc. It is possible that Imhotep, a military surgeon at the time of the pyramids, was the author of this detailed clinical report on 27 cases with head injury. It was at the time of the Alexandria Medical School in the third century bc that Erofilo from Calcedonia (Turkey), an early anatomist, suggested that the brain harbors motor, sensory, and cognitive functions.
It was only in the sixteenth century that Costanzo Varolio (1543–1575) suggested that higher cognitive functions might take place in the brain parenchyma. In the seventeenth century Thomas Willis (1621–1675), the English anatomist and surgeon, localized memory to the cortical gray matter, imagination in the white matter, perception and movement in the striatum, and involuntary actions to the cerebellum and brainstem. It was Giovanni Galvani (1737–1798), an Italian from Bologna, who demonstrated that nervous system activity is an electrophysiological, as opposed to hydrodynamic, phenomenon.
In the nineteenth century, the progress of Neurology was tremendous, due to multiple technical innovations and the application of the anatomoclinical correlation methods introduced by Giovan Battista Morgagni (1682–1771). It was Paul Broca (1824–1880) who declared “Nous parlon avec l’hemisphere gauche” in 1865. Sir Victor Horsley (1857–1916) was the first neurosurgeon to perform direct ESM of the cortex during resection of a deep lesion. Horsley identified an area of motor representation around the central sulcus.
Notwithstanding these innovations, the holistic theory developed by Flourens remained the dominant theory in the academic world throughout the first half of the 1900s. Studies of anatomy and neurophysiology provided more evidence for localization of brain functions during the first 2 decades of the twentieth century. In 1929 Hans Berger, an Austrian neuropsychiatrist, made the first report of the electroencephalogram (EEG) recorded from the scalp. In 1935 Otfrid Foerster (1873–1941) and H. Altenburger acquired the first electrocorticography, and identified the supplementary motor area (SMA). Wilder Penfield (1891–1976), the Neurosurgeon-in-Chief at the Montreal Neurologic Institute, was Foerster’s student. Penfield devised direct intraoperative ESM as we know it today. He performed ESM in 1132 epileptic patients, and found that not only motor and somatosensory areas but also language and memory sensations of “deja-vu” can be mapped on the brain surface.
George Ojemann, of the University of Washington in Seattle, provided a more recent historical contribution. He introduced the concept that language localization is highly variable among individuals. Ojemann suggested that the classic nineteenth century model of language localization was inaccurate for establishing the risk of aphasia in patients with a focal lesion in the dominant perisylvian cortex. Rather, the risk of developing neurologic deficits could be better assessed in each individual patient using cortical stimulation mapping techniques. Ojemann also performed the first controlled study of language-related activity in the human temporal lobe, using microelectrode recordings.
In the last 20 years modern noninvasive functional imaging techniques, including fMR imaging and positron emission tomography (PET), have been developed and quickly they have become the most popular methods to map the organization of the working human brain. These techniques measure local changes in brain blood flow and blood oxygenation associated with mental activity. The idea of inferring neuronal activity in the brain from a measurement of changes in local blood flow dates back to the observations made by Angelo Mosso, a nineteenth century Italian physiologist from Turin (Italy), in patients with skull defects following neurosurgical procedures. Mosso noted that pulsations of the human cortex change during mental activity and “blood very likely may rush to each region of the cortex according as it is most active.” He supposed that a very small adjustment in local blood flow follows the needs of the cerebral activity. The idea of using blood flow to map brain activity definitely preceded the ability to do such measurements.
There are at least 3 MR-based techniques that can measure brain function: T2∗-weighted susceptibility contrast, perfusion with arterial spin labeling, and in-flow MR angiography. All 3 fMR imaging techniques take advantage of the coupling of cerebral blood flow with oxygen consumption and neural activity. The most robust method with whole brain coverage and higher spatial resolution is susceptibility contrast fMR imaging, based on the discovery made by Ogawa and colleagues in 1990. Deoxyhemoglobin is paramagnetic, and changes in its concentration will affect the MR signal of blood. An increase in deoxyhemoglobin will determine an attenuation of the MR signal that is known as a blood oxygenation level dependent (BOLD) effect. Increments in oxygen content occur at the site of activation. fMR imaging with BOLD contrast provides indirect measurements of neuronal activity by monitoring local hemodynamic changes during performance of a task.
Functional Magnetic Resonance Imaging of Verbal Language
It is important to emphasize that patients with a focal brain lesion should be able to perform the task well to obtain a reliable presurgical mapping of the language network. Even though these patients are usually cooperative and motivated, they may be more anxious than healthy subjects, especially if the fMR imaging study is performed a few days before brain surgery. Because the aforementioned factors may interfere with the subject’s performance and the fMR imaging results, a careful preparation and evaluation of these patients is important.
The extension of the cortex activated during the fMR imaging experiment depends on the condition that is experimentally chosen to isolate the function of interest. The essence of language is the capacity to retain, retrieve, and combine arbitrary symbols of a native language into an infinite number of potential expressions. The sensory (input) and motor (output) processes as well as the attention-related functions that are associated with verbal language should be considered distinct from it. The choice of the resting (control) condition is also important, because the brain processes occurring will be inevitably subtracted from the tested condition. The resting condition will have to include all brain processes that are associated with the function of interest but are distinct from it.
Silent language tasks are usually preferred because motion artifacts are reduced. Stimulus input may be visual or acoustic. The former is preferable for evaluating specific language-processing components activating the temporal lobes, because language-related BOLD responses will not be confused with responses in primary auditory areas.
A variety of language paradigms have been developed: verbal fluency with word generation, verb generation, sentence comprehension, and passive listening. All these tasks are easy to implement, perform, and analyze. It has been shown that the use of multiple language paradigms increases the sensitivity and specificity of presurgical fMR imaging. Thus it would be preferable to use more than one language paradigm.
Verb generation (VGEN) is the most commonly used and probably the most reliable test in the clinical arena. Instructions are simple: given a word think of the associated verb. Most patients understand it well and are compliant once inside the magnet. Even patients with mild to moderate language deficits can perform it without great difficulty. The VGEN can be administered with a visual or auditory input. At the author’s institution the visual input is preferred. Performance of this task includes both language components: comprehension and production. The 3 main linguistic processes are involved: semantics, phonology, and syntax. These linguistic processes evoke a robust BOLD response both in the laterofrontal and temporoparietal language cortex. It has been shown that the VGEN is reliable in assessing language dominance.
Two additional tasks that nicely complement the VGEN are the verbal fluency and the sentence comprehension (SC) tasks. Word generation (WGEN) can be used as a verbal phonological fluency task: given a letter the subjects must generate a series of consecutive words starting with the letter shown. The SC task is designed to elicit a stronger response in the posterior language areas: given a one-line sentence to read, the subject has to recognize whichever one of two scenes best illustrates the sentence.
A controversial topic is whether a clear-cut functional parcellation of Broca area can be demonstrated with fMR imaging using different types of verbal fluency tasks: phonological, semantic, and syntactic. Intraoperative ESM studies have shown high specificity of the stimulated cortical areas. The fMR imaging literature suggests that semantic and phonological fluency tasks selectively activate left Brodmann areas (BA) 44 (pars opercularis) and 45 (pars triangularis) in the Broca area, respectively. However, this view has been challenged by a recent fMR imaging study that has shown all different types of verbal fluency tasks to evoke a strong BOLD response in BA 44 and 45. A single paradigm with low linguistic specificity but evoking BOLD response in all language cortical areas may not be so exciting for a cognitive neuroscientist; however, it would indeed be valuable in presurgical fMR imaging. To date, the VGEN language paradigm has been considered the most robust and the most widely used.
Interpretation of fMR imaging studies requires a high degree of experience. The statistical maps must be checked for quality and artifacts. The location of clusters of BOLD response in cortical areas that are putative nodes of the language network must be accurately identified. The spatial extension of the BOLD response in each location must be evaluated at different statistical thresholds to choose the optimal value. Spatial extent of the BOLD response can be highly variable among subjects, because it depends on the quality and quantity of signal acquired rather than on intrinsic boundaries of brain function.
There are several limitations inherent to the fMR imaging technique. There are important differences in the time course of electrophysiological and hemodynamic responses. This uncertainty of neurovascular coupling is a big challenge and a potential source of error in interpreting clinical fMR imaging studies. The BOLD response often localizes in the adjacent sulcus, and it can be a few millimeters away from the electrophysiologically functioning cortex. Colocalization of an important part of the BOLD signal with the draining vasculature is well known and studied. Not least, it should be kept in mind that activation of an area detected by fMR imaging and PET predominantly reflects the input to the area and the corresponding changes in information processing rather than output (ie, neuronal firing) from that area.
Diffusion Tensor Imaging
Diffusion tensor imaging (DTI) measures the effects of tissue microstructure on the random walks (Brownian motion) of water molecules in the brain. In tissues with an orderly oriented microstructure, such as the cerebral white matter, the measured diffusivity of water varies with the tissue’s orientation (anisotropic diffusion). Water diffuses fastest along the principal direction of the fibers and slowest along the cross-sectional plane. The DTI model provides the required information to construct a diffusion ellipsoid in each voxel of an imaging volume. DTI measures the diffusivities of water molecules along the 3 orthogonal axes of the ellipsoid (eigenvalues) and their average (mean diffusivity). Fractional anisotropy is a measure of eccentricity of the displacement of water molecules. In the healthy human brain, probably the most relevant factor affecting fractional anisotropy is the intravoxel orientation coherence of white matter fibers.
There are 3 main imaging output of DTI MR imaging: quantitative parametric maps displayed in gray scale (ie, fractional anisotropy maps), color maps showing the principal orientation of diffusion for each voxel, and 3-dimensional maps showing virtual dissection of tracts with streamline tracking methods. The aim of fiber tracking or MR tractography is to infer the 3-dimensional trajectories of white matter bundles by piecing together discrete estimates of the underlying continuous fiber orientation field measured noninvasively with DTI data.
Fiber tracking algorithms can be broadly classified into 2 types: deterministic and probabilistic. A DTI tractography atlas for virtual in vivo dissection of the principal human white matter tracts using a deterministic approach has been recently published. Few limitations of fiber tracking performed with the deterministic approach motivated the development of probabilistic tracking algorithms. It is important to understand well the inherent limitations of all methods of DTI-based virtual dissections and measurements. One important limitation is that in each voxel the eigenvector is the average of the orientation of all bundles included in the voxel. In volumes of white matter with many crossing bundles, as in the frontal and parietal paraventricular white matter, fractional anisotropy is low and the degree of uncertainty in the estimation of bundle orientation increases. The limitation of crossing fibers has been addressed with the development of more sophisticated imaging acquisition schemes using high angular resolution diffusion imaging (HARDI).
Functional anatomy of verbal language
Topology
The classic model of language was developed in the nineteenth century from retrospective studies that correlated neuropathology lesions with different types of aphasic syndromes. The model consists of a frontal expressive area (Broca), a posterior receptive area (Wernicke), and a connecting bundle (arcuate fasciculus, AF).
Topology is the study of places. The word derives from the Greek topos , meaning “place.” A functional cerebral network is made of specialized cortical areas (places or nodes) and their interconnecting white matter bundles (connections or pathways). Anterior frontal language cortical areas include the posterior part of the inferior frontal gyrus (IFG) that is known as the Broca area, the dorsolateral prefrontal cortex (DLPFC) in the middle frontal gyrus (MFG), and the ventrolateral prefrontal cortex (VLPFC), part of the premotor cortex. Posterior temporal language cortical areas include the superior temporal gyrus (pSTG), middle temporal gyrus (pMTG), and the cortex within the superior temporal sulcus (pSTS). Parietal language cortical areas include the supramarginal gyrus (SMG) and the angular gyrus (AG) in the inferior parietal lobule ( Fig. 1 ).
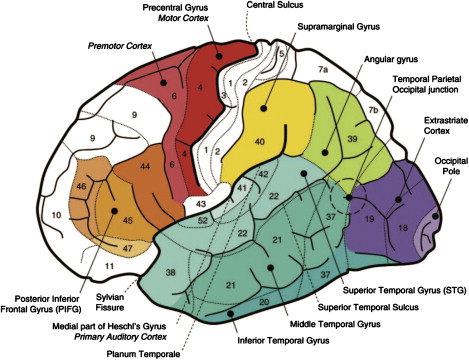
The robust fMR imaging language tasks that are used in clinical practice and are described herein identify well most of the areas included in the classic model. The tasks are robust also because they evoke simultaneously multiple linguistic processes.
The WGEN task gives a robust BOLD response in the Broca area in the dominant IFG, VLPFC, DLPFC, and STS, but it often gives a less consistent response in the inferior parietal lobule and in the posterior temporal cortex. The SC task gives a robust BOLD response in the dominant VLPFC, DLPFC, AG, and STS, but it gives a less consistent response in the Broca area. The VGEN task gives a robust and consistent BOLD response in all the aforementioned areas.
Modern neuroimaging studies of language processing with PET and fMR imaging suggested that the classic model might be oversimplistic and outdated, although still widely used. The new model represents multiple linguistic processes in the brain. According to the cognitive model verbal language is organized in parallel linguistic processes that include orthography, phonology, syntax, and lexical semantics. Functional imaging studies of individual linguistic processes have shown parcellation of activation in the classic language areas. Speech and language deficits are now classified according to the selective involvement of each linguistic process. Selection of the appropriate linguistic therapy in aphasic patients is also made according to the cognitive model.
Hodology
Hodology is the study of pathways. The word derives from the Greek hodos , meaning “path.” In the nineteenth century the anatomy of white matter connections was studied with the fiber dissecting technique at autopsy. In the second part of the twentieth century brain connections were studied with microscopic staining sections, and in vivo in the monkey with tracing autoradiographic techniques. With emerging of diffusion MR tractography it has now become possible to virtually dissect the main white matter pathways in vivo in humans. A new interest on the anatomy and function of the white matter pathways has emerged. In particular for presurgical mapping and speech rehabilitation, MR tractography has the potential to answer 2 very relevant questions: (1) Which bundles are involved in verbal language? (2) Will severing of a specific bundle during surgery lead to permanent language deficits? MR tractography in combination with new advanced neuropathology studies has the potential to test multiple hypotheses about the role that each pathway may play in several language processes. With diffusion MR tractography the hodological approach can be tested in clinicoanatomic correlation studies.
Current theories on brain organization suggest that cognitive functions such as verbal language are organized in widespread, segregated, and overlapping networks. Hickok and Poeppel recently proposed a dual-stream model for auditory language processing. From the pSTG, which is engaged in early cortical stages of speech perception, the system diverges into 2 processing streams. The dorsal stream projects dorsally toward the inferior parietal lobule and the posterior frontal lobe (DLPFC), and is involved in auditory-motor integration by mapping acoustic speech sounds to articulator representations. The prototype task targeting this dorsal stream is repetition of speech. The ventral stream projects ventrolaterally to the middle and inferior temporal cortices, and the VLPFC serves as a sound-to-meaning interface by mapping sound-based representations of speech to widely distributed conceptual representations. The prototype task targeting this ventral stream is listening to meaningful speech.
In brief, the dorsal pathway mediates sublexical repetition of speech, whereas the ventral pathway mediates comprehension. The dorsal pathway is likely composed of the AF and components of the superior longitudinal fasciculus (SLF-I and SLF-II), whereas the composition of the ventral pathway is a matter of debate. Some investigators suggest that it is composed by the inferior fronto-occipital fasciculus (IFOF), uncinate fasciculus, and inferior longitudinal fasciculus (ILF), whereas others have suggested a pathway coursing along the extreme capsule between the insular cortex and the claustrum.
The relevance of the several bundles involved in language in determination of hemispheric dominance has also been raised. Hemispheric asymmetries of these pathways connecting the inferoventrolateral frontal, temporal, and inferior parietal language nodes have been recently described using diffusion MR tractography.
Functional anatomy of verbal language
Topology
The classic model of language was developed in the nineteenth century from retrospective studies that correlated neuropathology lesions with different types of aphasic syndromes. The model consists of a frontal expressive area (Broca), a posterior receptive area (Wernicke), and a connecting bundle (arcuate fasciculus, AF).
Topology is the study of places. The word derives from the Greek topos , meaning “place.” A functional cerebral network is made of specialized cortical areas (places or nodes) and their interconnecting white matter bundles (connections or pathways). Anterior frontal language cortical areas include the posterior part of the inferior frontal gyrus (IFG) that is known as the Broca area, the dorsolateral prefrontal cortex (DLPFC) in the middle frontal gyrus (MFG), and the ventrolateral prefrontal cortex (VLPFC), part of the premotor cortex. Posterior temporal language cortical areas include the superior temporal gyrus (pSTG), middle temporal gyrus (pMTG), and the cortex within the superior temporal sulcus (pSTS). Parietal language cortical areas include the supramarginal gyrus (SMG) and the angular gyrus (AG) in the inferior parietal lobule ( Fig. 1 ).
The robust fMR imaging language tasks that are used in clinical practice and are described herein identify well most of the areas included in the classic model. The tasks are robust also because they evoke simultaneously multiple linguistic processes.
The WGEN task gives a robust BOLD response in the Broca area in the dominant IFG, VLPFC, DLPFC, and STS, but it often gives a less consistent response in the inferior parietal lobule and in the posterior temporal cortex. The SC task gives a robust BOLD response in the dominant VLPFC, DLPFC, AG, and STS, but it gives a less consistent response in the Broca area. The VGEN task gives a robust and consistent BOLD response in all the aforementioned areas.
Modern neuroimaging studies of language processing with PET and fMR imaging suggested that the classic model might be oversimplistic and outdated, although still widely used. The new model represents multiple linguistic processes in the brain. According to the cognitive model verbal language is organized in parallel linguistic processes that include orthography, phonology, syntax, and lexical semantics. Functional imaging studies of individual linguistic processes have shown parcellation of activation in the classic language areas. Speech and language deficits are now classified according to the selective involvement of each linguistic process. Selection of the appropriate linguistic therapy in aphasic patients is also made according to the cognitive model.
Hodology
Hodology is the study of pathways. The word derives from the Greek hodos , meaning “path.” In the nineteenth century the anatomy of white matter connections was studied with the fiber dissecting technique at autopsy. In the second part of the twentieth century brain connections were studied with microscopic staining sections, and in vivo in the monkey with tracing autoradiographic techniques. With emerging of diffusion MR tractography it has now become possible to virtually dissect the main white matter pathways in vivo in humans. A new interest on the anatomy and function of the white matter pathways has emerged. In particular for presurgical mapping and speech rehabilitation, MR tractography has the potential to answer 2 very relevant questions: (1) Which bundles are involved in verbal language? (2) Will severing of a specific bundle during surgery lead to permanent language deficits? MR tractography in combination with new advanced neuropathology studies has the potential to test multiple hypotheses about the role that each pathway may play in several language processes. With diffusion MR tractography the hodological approach can be tested in clinicoanatomic correlation studies.
Current theories on brain organization suggest that cognitive functions such as verbal language are organized in widespread, segregated, and overlapping networks. Hickok and Poeppel recently proposed a dual-stream model for auditory language processing. From the pSTG, which is engaged in early cortical stages of speech perception, the system diverges into 2 processing streams. The dorsal stream projects dorsally toward the inferior parietal lobule and the posterior frontal lobe (DLPFC), and is involved in auditory-motor integration by mapping acoustic speech sounds to articulator representations. The prototype task targeting this dorsal stream is repetition of speech. The ventral stream projects ventrolaterally to the middle and inferior temporal cortices, and the VLPFC serves as a sound-to-meaning interface by mapping sound-based representations of speech to widely distributed conceptual representations. The prototype task targeting this ventral stream is listening to meaningful speech.
In brief, the dorsal pathway mediates sublexical repetition of speech, whereas the ventral pathway mediates comprehension. The dorsal pathway is likely composed of the AF and components of the superior longitudinal fasciculus (SLF-I and SLF-II), whereas the composition of the ventral pathway is a matter of debate. Some investigators suggest that it is composed by the inferior fronto-occipital fasciculus (IFOF), uncinate fasciculus, and inferior longitudinal fasciculus (ILF), whereas others have suggested a pathway coursing along the extreme capsule between the insular cortex and the claustrum.
The relevance of the several bundles involved in language in determination of hemispheric dominance has also been raised. Hemispheric asymmetries of these pathways connecting the inferoventrolateral frontal, temporal, and inferior parietal language nodes have been recently described using diffusion MR tractography.
Mapping eloquent cortex
Cortical Localization with Intraoperative Direct Electrical Stimulation Mapping
Intraoperative direct ESM is a safe, precise, and reliable electrophysiological technique that is currently used to identify eloquent cortical areas and subcortical white matter connections. Devised by Penfield and Roberts, ESM is based on the observation that applying a direct current to an area on the cortical surface may block a specific function, although no local sensation is reported by the awake patient. Intraoperative ESM historically has been used to identify eloquent cortex during resection of epileptic foci, and only more recently for resection of brain neoplasm.
ESM results provide a different perspective from lesion-deficit correlations in patients with chronic stroke, multiple sclerosis or neurodegenerative diseases. The brief duration of the stimulation makes it unlikely that any functional reorganization occurs during the time the current is being applied. Until George Ojemann published his landmark study about “Cortical language localization in the left dominant hemisphere: an ESM investigation in 117 patients” with epilepsy, knowledge of language localization was based on “the lesion method” and clinical-neuropathological correlations. Ojemann was the first to systematically evaluate data from intraoperative ESM of human cortex. Intraoperative ESM studies found that verbal language areas are highly localized in patients and form several mosaics of 1 or less square centimeters. The area of individual mosaics and the total area related to language are usually much smaller than the classic Broca and Wernicke areas.
Ojemann’s variability maps of the dominant hemisphere showed for the first time that language localization was highly variable. Although often highly localized in the individual patient, essential sites directly stimulated during performance of the naming task had exceedingly different anatomic location in the patient population. Language was also identified in areas in which it was not expected to be found, based on the lesion method and the classic model. Eloquent sites were found far beyond the traditional boundaries of the Broca area. With the only exception of the pars opercularis, no cortical site exceeded 50% of eloquent naming sites. In the temporal-parietal lobes ESM evoked errors in less than 36% of tested patients. Ojemann concluded that neither the location nor the absence of language function at a given cortical site could be reliably predicted by anatomic considerations. His work had a significant impact on the neurosurgeon’s ability to operate safely within language-dense cortex.
Variability of Language Localization with Functional Magnetic Resonance Imaging
With the aim to measure interindividual variability of language cortical sites in healthy subjects, Cerliani and colleagues have produced surface variability maps . The investigators generated variability maps for the dominant and nondominant hemispheres for each of 3 language tasks (VGEN, WGEN, and SC) in right- and left-handers. The fMR imaging variability maps are color maps that illustrate, for each vertex of the standardized folded cortical mesh, how many subjects show a significant experimental effect. The images are powerful in displaying the variability of the location and extent of the cognitive processes involved during the task. The maps also show that all 3 tasks reliably determine hemisphere dominance. In comparison with the ESM variability maps they offer 2 main advantages: they are semi-automatically generated and both cerebral hemispheres are displayed.
The variability maps may be clinically useful in evaluating the probability that a focal lesion may determine a language deficit in a specific cortical area. The maps also may suggest the language task of choice to study the relationship of an infiltrating mass with the adjacent functioning cortex. For instance, the WGEN and VGEN are better indicated to study a glioma infiltrating the left IFG and the VGEN to study the AG, whereas the VGEN and SC should be used to study lesions in the posterior temporal cortex ( Fig. 2 ).
The variability maps may inform the neurosurgeon about the probability to find a positive ESM focus in a particular location. In the presurgical evaluation of patients with a focal mass the fMR imaging variability maps may be useful to answer the following question: “Is the BOLD response in the patient any different from the BOLD response in the normal population?”
In a brain tumor patient the atypical distribution of the BOLD response may be considered evidence of brain reorganization. Of note, plasticity mechanisms may act differently in glioma than in epileptic patients. More extensive reorganization may occur in low-grade glioma patients than in epileptic patients, because language function is more frequently preserved in the latter group of patients.
Validation of functional magnetic resonance imaging results with intraoperative electrical stimulation mapping
Several investigators have focused on presurgical fMR imaging feasibility, the choice of the most appropriate language task, and the spatial relationship between the lesion and the fMR imaging-activated tissue. In patients with a tumor around the central sulcus, investigators have reported a good correspondence between presurgical fMR imaging and intraoperative ESM results. Validation studies of presurgical language fMR imaging with ESM have involved small samples and the methods used for comparing eloquent foci have often been qualitative and subjective, with a few exceptions.
It must be emphasized that the physiologic measurements made with fMR imaging are different from those of intraoperative direct ESM. ESM is a disruption-based method that identifies nodes and connections essential to language processing. On the contrary, fMR imaging is an activation-based method that potentially identifies all nodes of the network with activity-related changes (essential and supplementary cortical areas).
The diagnostic accuracy of fMR imaging of language was measured by using intraoperative ESM as the reference standard in few studies. In only 3 studies was a site-by-site correlation with a relatively large number of ESM tags performed.
FitzGerald and colleagues evaluated 140 sites in 11 patients with 5 language tasks, and found a sensitivity of 81% and a specificity of 53%. Sensitivity was higher for results from all tasks combined than for results from a single task. VGEN was the language task with highest sensitivity. Roux and colleagues evaluated 426 tags in 14 patients. Using the VGEN and naming tasks combined and a high statistical threshold ( P <.005), they measured a sensitivity of 59% and a specificity of 97%, respectively. Decreasing the analysis threshold the sensitivity increased to 66% and the specificity decreased to 91%.
Bizzi and colleagues evaluated 141 tags in 17 patients with a focal mass in the perisylvian cortex ( Fig. 3 ). The location of all stimulated ESM tags was recorded on the fMR imaging dataset ( Fig. 3 D). This procedure is important in the determination of true and false results, and it may also reduce operator-dependent bias. An overall sensitivity of 80% and specificity of 78% for mapping language with the VGEN task was reported in this study. In addition, the investigators showed that sensitivity and specificity might vary according to lesion type and glioma grade. Sensitivity and specificity were 100% in cavernous angioma. Sensitivity was higher in glioma World Health Organization (WHO) grade II and III (93%) than in glioblastoma multiforme (GBM) (65%). On the contrary, specificity was higher in GBM (93%) than in glioma grade II (79%) and III (76%). There are 2 possible explanations for the higher rate of false-negative fMR imaging results found in GBM. Angiogenesis may lead to abnormal vasculature formation with loss of neurovascular coupling and absence of the BOLD response, despite the presence of neural activity. An alternative explanation is that the larger brain shift occurring in GBM, as the dura is exposed, may diminish the accuracy of the coregistration of preoperative imaging data with the brain tissue on the neuronavigation device.
The sensitivity reported by Roux and colleagues is lower than that reported by the FitzGerald and Bizzi groups. Differences in sensitivity and specificity results among the 3 studies may be explained in part by differences in patient population (tumor type, location, and craniotomy size), fMR imaging paradigms, and methods of analysis used. Bizzi and colleagues reported that sensitivity was higher in anterior than in posterior language areas. A higher percentage of ESM-positive sites for speech arrest in the anterior frontal cortex was reported by Ojemann and colleagues. This finding is relevant for presurgical evaluation, because verbal fluency and verbal memory are 2 functions that are critical to preserve, especially in patients who have built their professional career on those skills. The 2 linguistic processes are most likely segregated in the anterior frontal cortex.
Patient outcome for permanent deficits was 11% in the Bizzi series of 34 patients: 1 patient had severe morbidity due to surgical complications independent of functional localization techniques and 3 patients had moderate or mild permanent postoperative deficits. Other investigators have shown a low rate of postoperative deficits in patients who had eloquent tissue within the tumor when the mass was near the surface and could be evaluated with ESM. Roux and colleagues found fMR imaging intratumoral activated foci in 6 patients.
The ideal mapping technique should be both sensitive and specific. A method with a low rate of false-negative foci will have high sensitivity and will rarely miss functioning tissue. A method with a low rate of false positivity will have high specificity. The investigators of the studies reported here have shown that sensitivity of presurgical fMR imaging of language is consistently high, whereas its specificity may be lower than desired.
Notwithstanding these encouraging results, if fMR imaging is to be used as a reliable and accurate tool for planning and performing function-preserving radical tumor exeresis, its results will also have to be validated with postoperative clinical outcome.
Aims of presurgical planning with functional magnetic resonance imaging
The importance of functional localization has been only recently emphasized and, as discussed earlier, is only in part predictable by anatomic landmarks. fMR imaging and diffusion MR tractography are adding pieces of information once unthought-of of, and they may be useful for guiding surgical planning and intraoperative mapping.
Patient Selection Criteria for Awake Craniotomy
The localization of language eloquent cortex is the most critical factor in planning a cortical trajectory and resection of a tumor. Selection of patients for awake craniotomy has been historically based on clinical presentation and proximity of the tumor to putative functional cortex on conventional MR imaging. It is important to understand functional anatomy of verbal language. Prompt identification of sulcal anatomy on 3-dimensional morphologic MR images is the key.
Unfortunately, the reliability of anatomic landmarks is low in healthy subjects and even lower in patients with a focal mass. Quiñones-Hinojosa and colleagues have addressed this issue and retrospectively found a strict correlation between anatomic variant types of sulcal anatomy and location of the speech arrest area with ESM. However, normal sulcal anatomy is often distorted by glioma infiltration, and eloquent cortex may be displaced by the mass or even reorganized at a distance.
High variability of language localization has been demonstrated by Cerliani and colleagues in healthy subjects with fMR imaging, and by Ojemann and colleagues in patients who underwent surgery for epilepsy with intraoperative ESM. Sanai and colleagues have shown that variability may be even higher in patients with a brain glioma infiltrating Broca, Wernicke, or even the dominant temporal pole. Localization of speech is even more problematic in patients who are fluent in different languages. Eloquent tissue may occasionally be found even within the infiltrating tumor.
fMR imaging has the potential to identify functional cortical tissue that, if damaged during surgery, may cause permanent neurologic sequelae. In the last decade the use of presurgical fMR imaging has increased tremendously. However, it is consistently applied only in highly specialized medical centers. It has been demonstrated that presurgical fMR imaging with DTI may reduce the risks and favor a more aggressive resection of the tumor. According to Ulmer and colleagues the risk of developing permanent postoperative deficits is low when the resection margin is at least 5 mm away from a functional site. These investigators reported 4% of unplanned surgically induced motor deficit in a series of 24 patients with the tumor near a motor eloquent area. In certain patients surgical time may be shortened, the extent of resection increased, and craniotomy size decreased.
Can Functional Magnetic Resonance Imaging Replace the Wada Test?
Functional hemispheric language lateralization has been correlated with handedness: approximately 90% of right-handers and 70% of left-handers show left-sided hemispheric language dominance, whereas 15% of left-handers show right-sided lateralization and the remaining 15% a bilateral representation. Of note, approximately 10% of the population is a true left-hander, and left-handedness is less common in women than in men. However, the influence of gender on hemisphere dominance remains a controversial issue. These data imply that roughly 92.5% of the population is left-hemisphere dominant and that only approximately 4% is right-hemisphere language dominant.
In the past, various methods have been employed to assess hemispheric dominance in epileptic patients. For the last 50 years the Wada test has been the standard of care. The Wada test is an invasive procedure with selective catheterization of both carotid arteries, and injection of amobarbital in the anterior and middle cerebral arteries of one hemisphere at a time. The drug causes temporary anesthetization of the brain tissue. Cerebral dominance for language and memory is determined on the patient’s performance during the period of functional disability. The Wada is a procedure associated with patient discomfort, high cost, and morbidity that greatly exceed those of noninvasive functional imaging methods.
Several investigators have shown that fMR imaging and the Wada test agreed on determination of language lateralization in most patients. Despite the overall agreement, these results must be taken with caution because of several important technical issues. Most investigators compute an fMR imaging laterality index (LI) using a region-of-interest (ROI) method. There are many experimental choices that can affect the final laterality result: the language task, the size and location of the selected ROI in the left and right hemispheres, and the statistical threshold.
Notwithstanding, fMR imaging has taken over the role of the Wada test in most medical centers because it is noninvasive, more accessible, and less expensive. In few highly specialized imaging centers with magnetoencephalography (MEG) capabilities, determination of the dominant hemisphere is relied on using the combination of fMR imaging and MEG. In a study on 172 patients with brain tumor abutting language areas, it was shown that measurement by fMR imaging and MEG combined increased the reliability of language localization.
Can Functional Magnetic Resonance Imaging Replace Intraoperative Electrical Stimulation Mapping?
At present, ESM is the reference standard for intraoperative decisions because it has been used extensively and it has been validated by clinical outcome. If eloquent sites identified by ESM are respected, the risk of developing permanent postoperative sequelae is low. Duffau and colleagues have compared 2 series of patients with supratentorial low-grade glioma operated by the same team: those operated with intraoperative ESM (n = 122) versus those operated without (n = 100). The study found that the use of intraoperative direct ESM lead to increasing percentage of surgery in eloquent areas (63% vs 35%) with decreasing severe permanent deficits (3% vs 17%). The quality of tumor resection was improved, with a favorable impact on survival. In a prospective longitudinally study designed to evaluate verbal language postoperative outcome in 149 patients with tumor in close proximity to or within language areas, Ilmberger and colleagues reported new language impairment at 7 days after awake craniotomy in 41 of 128 patients, without preoperative aphasic deficits. At 7 months after surgery, 14 patients continued to show mild language impairment. In this group of patients aphasic disturbance were mostly mild.
For fMR imaging to be mature to replace intraoperative direct ESM, it should be accurate in identifying brain tissue that can be safely removed. False-negative findings could possibly lead to postoperative language deficits. Type I errors occur infrequently in low-grade glioma and cavernous angiomas, as discussed in the validation subsection earlier. False-positive results are also undesirable, because they may discourage radical resection of the tumor. These additional nonessential areas identified by fMR imaging may be part of the network but if damaged will unlikely cause a permanent deficit.
The accuracy of fMR imaging in identifying the tissue that the neurosurgeon may take out must be also confirmed with outcome studies. Few similar studies have been performed thus far. Future larger fMR imaging studies will have to show that the long-term postoperative outcome is favorable. At the current state of the art, fMR imaging mapping is a useful guide in presurgical and intraoperative planning but does not replace the need for intraoperative testing in any patient with a functional language site less than 10 mm away from the tumor’s resection margin.
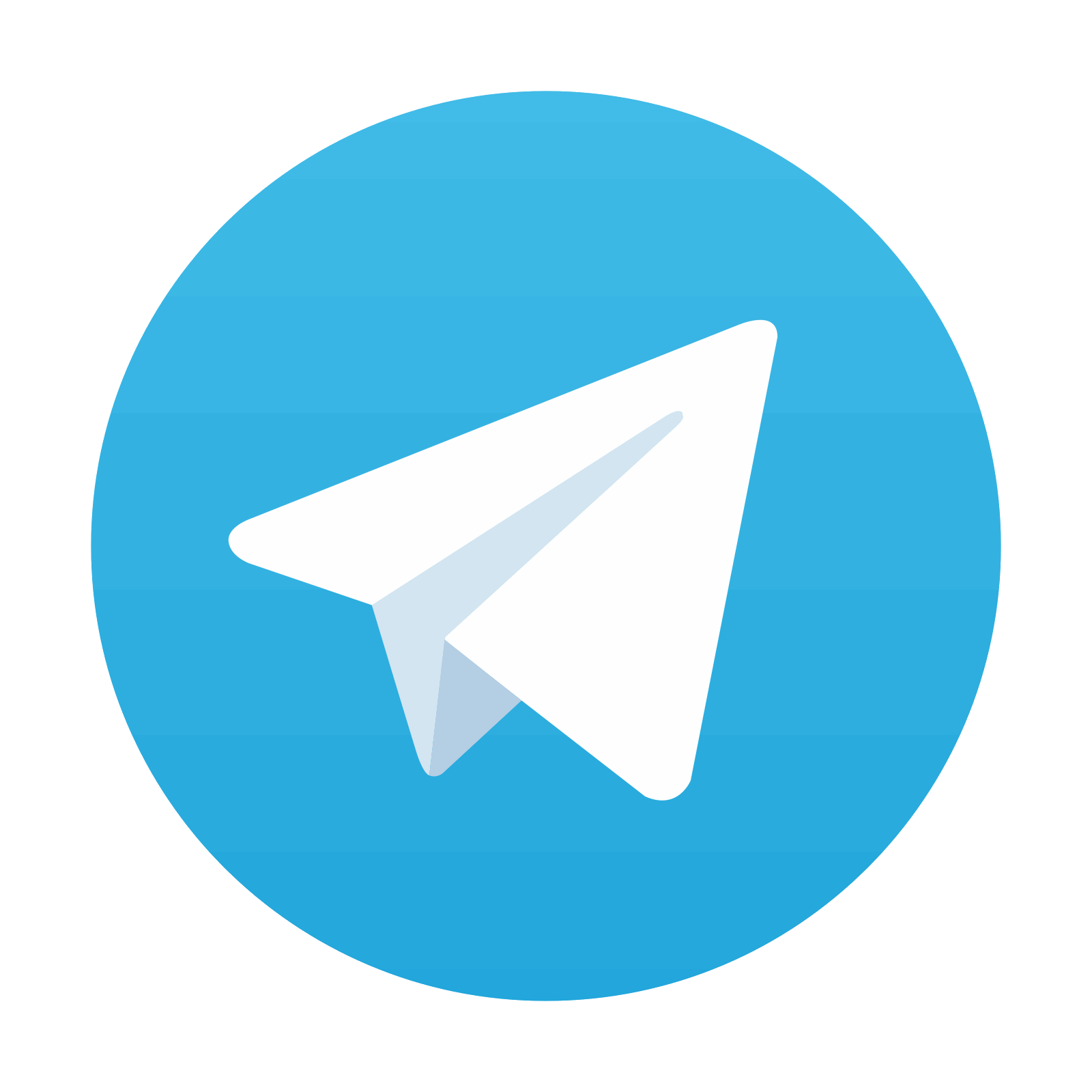
Stay updated, free articles. Join our Telegram channel

Full access? Get Clinical Tree
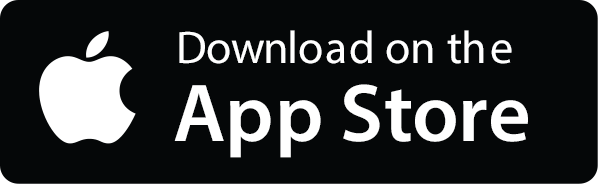
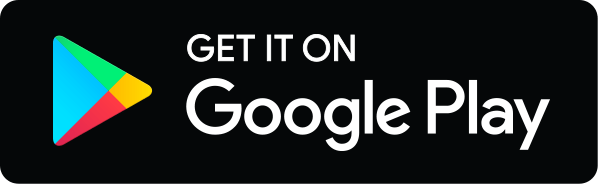