Pathologies involving the pulmonary arterial system include pulmonary embolus (PE), pulmonary arterial hypertension (PAH), congenital anomalies, and pulmonary artery tumors. These diseases present a unique clinical diagnostic challenge often requiring multiple diagnostic examinations spanning the entire radiologic armamentarium. Initially, cardiovascular magnetic resonance (CMR) played only a minor role in evaluation of the pulmonary arterial system. However, with the advent of improved, high-performance gradients and bolus gadolinium, contrast-enhanced cardiovascular magnetic resonance (CE-CMR) is now well suited for this challenge. It not only provides precise, three-dimensional (3D) anatomic information, but also provides functional data critical to a complete evaluation of the pulmonary arterial system. Beyond the basic flow parameters, four-dimensional (4D)-CMR is able to assess information about more advanced parameters (e.g., wall shear stress); however this technique requires standardization before routine clinical application. Further developments are expected in the field of molecular imaging by using target-specific contrast agents in humans, allowing the interaction and detection of pathological mechanisms. CMR is noninvasive and requires neither iodinated contrast nor ionizing radiation, and consistently demonstrates anatomic accuracy comparable with that of computed tomography (CT) while providing reproducible functional data previously only available by catheter based pulmonary angiography.
Pulmonary Embolism
Venous thromboembolism (VTE) incorporates deep venous thrombosis (DVT) and PE. VTE is one of the major contributors to global disease burden: the incidence ranges from 100 to 200 per 100,000 persons per year with an increased incidence from 200 to 700 per 100,000 in those ≥70 years. The risk for the disease doubles in each decade after the age of 40 years. PE represents the most serious and potentially life-threatening condition related to VTE. It often occurs as a consequence of DVT when a blood clot breaks off, migrates, and eventually obstructs the pulmonary arteries. An estimation model based on data from six countries of the European Union showed that 317,000 deaths could be attributable to VTE. Approximately one-third of patients suffered from sudden death caused by PE, 59% died as a result of existing but undiagnosed PE, and only 7% of patients who died were appropriately identified with PE. Because the presenting signs and symptoms are nonspecific and overlap in a wide variety of common ailments, diagnosing PE is challenging and relies on a multidimensional prognostic model. Risk stratification of patients into high-risk, intermediate-risk, and low-risk categories is based on the additive value of clinical scores, imaging tests, and laboratory markers. Therefore the current workup typically includes two or more of the following tests: blood assays, computed tomography angiography (CTA) or ventilation/perfusion (V̇/Q̇) scintigraphy, compression venous ultrasonography (CUS) of the deep venous system, and occasionally the reference standard, catheter-based pulmonary angiography. From the resulting array of diagnostic information, the clinician must then assess the patient’s likelihood of having PE and initiate treatment. The most extensively used clinical score in acute PE is the pulmonary embolism severity index (PESI) or its simplified version (sPESI), which is helpful in the prognostic assessment of 30-day mortality. For hemodynamically stable patients, pretest probability relies on the revised Geneva score and/or Wells rule, which both aim to stratify patients into three-level (low, intermediate, and high risk) or two-level (PE unlikely and PE likely) schemes based on information that is easy to obtain. Elevated plasma levels of D-dimer as well as markers of myocardial injury (cardiac troponin I or T) and/or ventricular dysfunction (brain natriuretic peptide-BNP and N-terminal-pro BNP) support the diagnosis of PE. However, the relatively low positive predictive values of laboratory biomarkers do not allow reliable exclusion of PE. The suspicion of PE is therefore a common indication for imaging.
CTA is the most used noninvasive imaging method for detection of PE. A negative CTA in patients with low/intermediate risk allows for safely ruling out PE, although in patients with negative CTA, but high probability of PE, further investigations are required. The high spatial resolution of current CT scanners and the administration of contrast media allows for the identification of PE at least to the segmental level. However, potential pitfalls can occur, especially at distal levels of the pulmonary arteries. A retrospective review of PE on CTA showed discordance between subspecialists in chest radiology and general radiologists in 26% of the cases. The disagreement was most frequent if the PE was adjudicated as solitary (46% of solitary PEs were later adjudicated as negative) or segmental/subsegmental (27% of segmental and 59% of subsegmental PE were later considered as negative). Additionally, CTA exposes the patient to ionizing radiation and requires iodinated intravenous contrast, which is potentially nephrotoxic and can provoke allergic reactions. Radiation exposure is of particular concern in young adults and those who are referred for multiple CTA scans because of a history of prior PE.
V̇/Q̇ scintigraphy is a combined noninvasive procedure that uses nuclear radiotracers for diagnosing PE. The reported radiation exposure of the examination is significantly lower compared with CTA (1.1 mSv vs. 4 mSv) and might be a preferred alternative over CTA in younger patients, especially women, in whom radiation exposure of CTA is associated with higher risk of breast cancer. However, V̇/Q̇ scans are frequently nondiagnostic, which raised concerns because of the need for further diagnostic tests. Diagnostic accuracy can be improved by incorporation of a single photon emission tomography (SPECT) to V̇/Q̇ scintigraphy.
Catheter-based pulmonary angiography remains the reference standard technique for the exclusion of PE. The use of this method, however, is infrequent due to the good diagnostic accuracy provided by noninvasive CTA. Furthermore, the invasive nature of the test carries risk for procedure-related complications, especially in patients with hemodynamic instability and/or respiratory failure.
CUS is useful for detection of DVT, which is thought to be a predisposing condition for development of PE. However, a negative test does not exclude the presence of PE: in one study, CUS found DVT in only 30% of patients with proven PE. A proximal DVT detected by CUS in patients with isolated subsegmental PE is an adequate finding to start anticoagulation therapy. However, an ongoing trial raised concerns about treating small blood clots attributed to the elevated risk of bleeding and investigates withholding anticoagulants in patients with subsegmental PE without cancer.
The prognostic value of CMR for diagnosing PE has been investigated in the prospective, multicenter Prospective Investigation of Pulmonary Embolism Diagnosis (PIOPED) III trial. However, the results were disappointing: CMR images were technically unsatisfactory in 25% of patients. Another study found that 30% of CMR scans were inconclusive. Furthermore, the latter investigation reported high sensitivity for proximal PE (97.7%–100% assessed by two readers) but significantly lower sensitivity for segmental (68%–91.7%) and subsegmental PE (21.4%–33.3%). Another issue is that access to CMR is relatively limited, particularly outside of office hours. Current guidelines therefore do not include CMR in the primary imaging work-up of PE. Nevertheless, because of its radiation-free nature CMR remains a useful imaging modality for detection of PE, especially in young women and children.
There are two fundamental and complementary approaches to CMR in the diagnosis of PE. The earliest approach builds on the model of V̇/Q̇ scintigraphy and uses CE-CMR to evaluate pulmonary perfusion, to identify segmental, wedge-shaped perfusion defects in the pulmonary parenchyma. In this regard, several investigations demonstrated good agreement between CMR lung perfusion and V̇/Q̇ scintigraphy. Another method of diagnosing acute PE on CMR is the visualization of vascular alterations, which include intravascular filling defects, abrupt cutoff of intravascular signal and the absence of contrast material distal to the obstructed artery. Furthermore, CMR allows for accurate evaluation of flow changes in the bronchial arteries in patients with chronic PE.
Molecular CMR with the use of specific contrast agents might also allow for direct visualization of thrombus formation. Fluorine (F) CMR and α2-antiplasmin (α2 AP ) labeled contrast agents have been shown to be able to detect thrombi with a diameter <0.8 mm in murine inferior vena cava. Further development of this method in humans might help in the identification of evolving thrombi following invasive procedures with increased risk for thrombosis.
Ferumoxytol is an intravenous iron preparation intended for treatment of the anemia associated with chronic kidney disease. It is a carbohydrate-coated, superparamagnetic iron oxide nanoparticle. Ferumoxytol has been used off-label for CMR as a contrast agent for vascular imaging. It does not contain gadolinium and therefore might be beneficial in patients with renal impairment. The prolonged steady state of ferumoxytol allows T1-weighted CMR to be performed under steady-state conditions (rather than only first-pass imaging with conventional gadolinium contrast agents). Thus ferumoxytol has been proposed to be useful in patients who have difficulty with breath-holding.
Pulmonary Artery Hypertension
PAH is an enigmatic progressive condition characterized by abnormally elevated blood pressure in the pulmonary artery circulation. Normally, the pulmonary arterial system is a low-resistance vascular bed with a mean arterial pressure of 14 ± 3 mm Hg and with an upper limit of 21 mm Hg. PAH is defined as a mean pulmonary arterial pressure of ≥25 mm Hg measured by right heart catheterization. Poor reporting of PAH hampers the estimation of its incidence on a global level. In the United Kingdom, a prevalence of 97 cases per million has been reported; in the United States the age-standardized death rate ranges between 4.5 and 12.3 per 100,000 persons. The clinical classification of the disease has recently been updated attributed to newly recognized clinical entities (e.g., new gene mutations) and other conditions that might require the establishment of new subcategories. The new classification includes PAH (previously primary PAH), PAH as a result of left heart disease, PAH caused by lung disease and/or hypoxia, chronic thromboembolic PAH and other pulmonary artery obstructions, and PAH with unclear or multifactorial mechanism. The specific cause of the disease initiates several pathophysiologic mechanisms (vasoconstriction, cell proliferation, remodeling of the pulmonary arterial wall), which increase pulmonary vascular resistance and eventually lead to the development of right ventricular (RV) dysfunction. As pulmonary arterial pressure rises, patients experience an insidious onset of nonspecific symptoms, such as progressive dyspnea and exercise intolerance, which are often mistaken for signs of coronary artery disease, thereby delaying diagnosis and treatment. Although comprehensive registries demonstrated improved prognosis, early diagnosis is of utmost importance because patients experience poor quality of life.
Establishing PAH currently requires numerous tests interpreted in concert. CMR has been suggested as a “one-stop shop” imaging technique for the evaluation of PAH and its impact on the right ventricle. The assessment of the pulmonary vascular and circulatory changes encompasses the following CMR techniques: 2D and 3D CE-CMR, CMR lung perfusion, and velocity-encoded phase-contrast (PC) imaging. Radiological signs on CE-CMR indicative of PAH include the dilation of the main pulmonary artery, abnormal proximal-to-distal tapering (“pruning”) of pulmonary vasculature, and reduction and tortuosity of the distal vessels ( Fig. 45.1 ). CE-CMR also assists in establishing the etiology of the hypertension. Findings associated with chronic thromboembolic PAH such as recanalization and organization of thrombus reflects the underlying pathology of the disease. CE-CMR demonstrates thrombotic wall thickening and irregularity, as well as intraluminal webs. Because CTA has essentially supplanted catheter angiography for the clinical diagnosis of PE, it has quickly become the study of choice for evaluation of PAH. However, CE-CMR shows similar accuracy when compared with CTA in identifying the presence of chronic thromboembolic disease in patients with PAH. Furthermore, CE-CMR is the desirable imaging modality in clinical scenarios such as chronic PE in pregnant women, young patients, or in case of multiple follow-up examinations. CMR lung perfusion imaging can also be used to diagnose and characterize PAH. Patients with PAH typically demonstrate perfusion defects that are characteristically segmental and/or focal (in chronic thromboembolic PAH) ( Fig. 45.2A ) and patchy and/or diffuse (in nonthromboembolic PAH). The diagnostic accuracy of CE-CMR–generated perfusion for the detection of chronic thromboembolic-related perfusion defects is comparable with CTA or conventional radionuclide scintigraphy ( Fig. 45.2B ). Furthermore, the combined use of CMR lung perfusion and CE-CMR is able to distinguish chronic thromboembolic PAH from nonthromboembolic PAH with an accuracy of 90%. A recent investigation demonstrated the potential of CMR lung perfusion for the quantification of perfusion changes in patients before and after pulmonary artery endarterectomy.
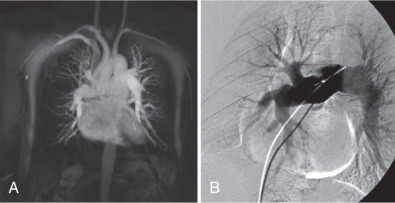
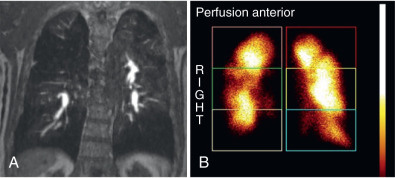
CMR assessment of the pulmonary arteries can not only accurately diagnose PAH and differentiate its cause, but also can provide functional assessments, which can help guide treatment and predict response. CMR can provide structural and quantitative functional analysis of the RV, including RV ejection fraction and mass, which are not readily obtained by echocardiography. A metaanalysis of eight studies investigated 21 CMR parameters and found RV ejection fraction to be the strongest predictor of mortality in PAH. CMR also allows assessment of the interventricular septum, which bows into the left ventricle when RV pressure exceeds left ventricular pressure. The amount of septal curvature is linearly related to right heart pressure as described by Laplace’s law. PAH has also been associated with a vortex of blood flow in the main pulmonary artery on velocity encoded PC CMR: the relative period of existence of the vortex showed high correlation with pulmonary artery pressure in patients with manifest PAH. The assessment of wall shear stress (WSS) profile is also feasible by both velocity encoded PC CMR and 4D-CMR. In patients with PAH, significantly reduced WSS was observed, which might affect the endothelial function and may lead to remodeling of the proximal pulmonary artery.
Dilatation of the bronchial arterial system has been noted in patients with PAH as a collateral pathway for providing blood to the lungs for gas exchange. The total area of bronchial arteries is significantly larger in patients with chronic thromboembolic PAH than in those with nonthromboembolic PAH. Larger total area of the bronchial arteries is not only indicative of the presence of chronic thromboembolic PAH, but also correlates with improved outcomes after surgery for thrombotic hypertension. Previously, the degree of bronchial arterial shunting could be measured only by an invasive contrast-medium dilution technique. However, by using velocity-encoded PC CMR of the pulmonary arteries, increased bronchial arterial shunting can be identified noninvasively in patients with chronic thromboembolic PAH. Finally, late gadolinium enhancement at the RV insertion points of interventricular septum has been shown to be associated with more advanced disease and poor prognosis.
CE-CMR and CMR perfusion techniques used for detecting acute PE are equally applicable to diagnose the anatomic changes of PAH, including thrombotic wall thickening, increased pulmonary arterial diameter, abnormal vessel tapering, and segmental or patchy perfusion defects. Additionally, CMR allows for the acquisition of structural and functional changes of the heart, and provides information for systemic-pulmonary arterial shunting. Further refinement in CMR including vascular imaging and the incorporation of 4D-CMR in the clinical setting can considerably contribute to the differential diagnosis and monitoring of PAH without ionizing radiation exposure or iodinated contrast material.
Congenital Vascular Disorders
A wide spectrum of congenital heart diseases (CHD) and congenital vascular anomalies affect the pulmonary arteries. Precise anatomic delineation of the abnormality is essential to plan and perform operative therapy. Echocardiography is the initial imaging modality for the diagnosis of pulmonary vascular anomalies. However, during the past years the use of other imaging modalities, such as CTA and CMR, has considerably increased to complement echocardiography. Numerous studies demonstrated the efficacy of CMR for detecting complex CHD affecting the pulmonary arterial and venous circulation and preoperative planning for a wide range of disorders, including the spectrum of tetralogy of Fallot, hypoplastic pulmonary arteries, partial anomalous pulmonary venous connection ( Fig. 45.3 ), pulmonary artery stenosis ( Fig. 45.4 ), pulmonary artery sling ( Fig. 45.5 ), and patent ductus arteriosus ( Fig. 45.6 ).
