Pulmonary vascular assessment commonly relies on computed tomography angiography (CTA), but continued advances in magnetic resonance angiography have allowed pulmonary magnetic resonance angiography (pMRA) to become a reasonable alternative to CTA without exposing patients to ionizing radiation. pMRA allows the evaluation of pulmonary vascular anatomy, hemodynamic physiology, lung parenchymal perfusion, and (optionally) right and left ventricular function with a single examination. This article discusses pMRA techniques and artifacts; performance in commonly encountered pulmonary vascular diseases, specifically pulmonary embolism and pulmonary hypertension; and recent advances in both contrast-enhanced and noncontrast pMRA.
Key points
- •
Pulmonary magnetic resonance angiography (pMRA) is an effective nonionizing examination for the exclusion of pulmonary embolism.
- •
pMRA with cardiac magnetic resonance imaging provides quantitative information predictive of outcome in patients with pulmonary hypertension.
- •
Knowledge of common pMRA artifacts is crucial.
- •
Future uses of noncontrast magnetic resonance angiography and four-dimensional flow magnetic resonance imaging for pMRA remain to be explored.
Introduction
Historically, magnetic resonance angiography (MRA) has been used for the evaluation of the systemic circulation, including the thoracic and abdominal aorta and branches, the renal arteries, upper and lower extremity peripheral vasculature, and head and neck vasculature. This technique most commonly uses gadolinium-based contrast agents to opacify the vasculature of interest, and, because magnetic resonance (MR) imaging is not limited by concerns related to ionizing radiation, multiple images can be acquired over the course of contrast transit to provide time-resolved MRA (TR-MRA) or dynamic, contrast-enhanced MRA (CE-MRA). Noncontrast MRA techniques are also available, and, with improved MR imaging hardware and acceleration approaches, extremely high spatial and temporal resolution MRA images can be acquired.
With these improvements, there has been increasing use of MRA for the evaluation of pulmonary vascular diseases, including pulmonary embolism (PE) and pulmonary hypertension (PH). These commonly encountered diseases can result in significant morbidity and mortality if not correctly diagnosed, and medical imaging plays an important role in evaluating the underlying causes and complications of these and other pulmonary vascular diseases. Most commonly, pulmonary vascular assessment relies on computed tomography angiography (CTA) and can be supplemented by nuclear medicine ventilation-perfusion (VQ) scintigraphy. In PH, invasive measurement of right heart pressure and estimated pulmonary vascular resistance is also commonly performed. One of the key advantages of pulmonary MRA (pMRA) relative to these other techniques is the opportunity to evaluate pulmonary vascular anatomy, hemodynamic physiology, lung parenchymal perfusion, and (optionally) right and left ventricular function with a single examination without exposure to ionizing radiation.
This article provides an overview of pMRA acquisition techniques and its performance and potential in commonly encountered pulmonary vascular diseases. It also provides an outlook for additional pMRA applications and advanced techniques that are actively being investigated.
Pulmonary magnetic resonance angiography technique
MRA evaluation of the pulmonary vasculature is best accomplished using CE-MRA, with imaging timing optimized for visualization of the pulmonary arteries (PAs). In general, gadolinium-based contrast agent is injected through a peripheral intravenous catheter, and timing for PA imaging can be performed using test timing bolus or fluoroscopic triggering. For high-quality imaging, a 0.1-mmol/kg dose of high-relaxivity contrast agent is recommended. Note that several gadolinium-based contrast agents have been approved for MRA, but not specifically for pMRA. The contrast can be diluted in normal saline for a total injected volume of 30 mL with injection rate of 1.5 mL/s, which helps improve artifacts related to contrast bolus timing (discussed later). Proper imaging timing is crucial for high-quality pMRA, and our protocol consists of manual fluoroscopic triggering with real-time two-dimensional (2D) images acquired in an axial or sagittal oblique orientation and scanning initiated when contrast is seen in the right ventricle.
An effective pMRA protocol has previously been described by Nagle and colleagues and is outlined in Table 1 . A typical CE-MRA protocol consists of a rapid three-dimensional (3D) spoiled gradient-echo sequence acquired in the coronal orientation. To limit respiratory motion artifact, which can significantly degrade image quality, breath holding is generally required but can be difficult for patients with cardiopulmonary diseases. Recent advances in image acceleration, namely 2D autocalibrated parallel imaging, now allows both shorter breath holds and higher spatial resolution. , Imaging can be performed at both 1.5 T and 3.0 T, with slightly higher spatial resolution available at 3.0 T without a significant reduction in signal-to-noise ratio. Of note, it is our practice to perform sequential MR images immediately after and approximately 30 to 60 seconds following the initial PA phase images. These series tend to be helpful for problem solving if contrast bolus timing or other artifacts degrade the initial pMRA and also allow for evaluation of the pulmonary veins and systemic vasculature. When properly implemented, pMRA examinations take less than 10 minutes on the scanner and can be deployed in the emergency setting ( Fig. 1 ).
Series | Use | Notes | |
---|---|---|---|
1 | Three-plane single-shot fast spin echo | Localizer | — |
2 | Precontrast coronal T1-weighted 3D SGRE | Assess for appropriate anatomic coverage | Must have adequate AP coverage Wrap artifact from shoulders should be minimized |
3 | Pulmonary arterial-phase T1-weighted 3D SGRE | PA visualization Lung parenchymal perfusion assessment | Fluoro triggered Bolus timing and truncation artifacts can reduce diagnostic accuracy |
4 | Immediate postcontrast T1-weighted 3D SGRE | PA visualization | Can be helpful if series 3 is degraded |
5 | Low-flip-angle postcontrast T1-weighted 3D SGRE | Pulmonary and systemic vasculature visualization | Performed 1–2 min after injection Low flip angle allows increased signal in vessels even though contrast is diluted |
6 | T1-weighted 2D axial fat saturated | Assess nonvascular structures | — |
Optional Series | |||
1 | Axial maximal intensity projections of series 3 | PA visualization | Can be helpful, particularly for novice readers |
2 | Time-resolved MRA | PA visualization Lung parenchymal perfusion assessment | Can allow for quantification of pulmonary hemodynamics |
3 | 4D flow MR imaging | Velocity and flow visualization and quantification | Allows hemodynamic assessment of pulmonary vasculature in single acquisition Requires specialized postprocessing and viewing software |
3 | Cardiac cine imaging | Cardiac qualitative and quantitative function | Useful in pulmonary hypertension evaluation |
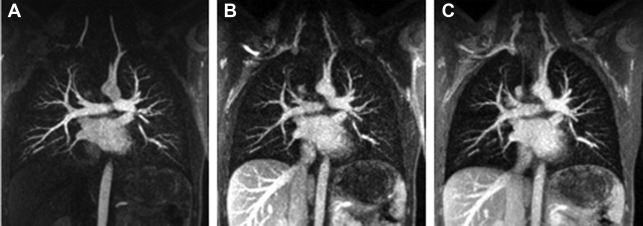
Time-resolved MRA can be included as a part of pMRA, which allows for imaging of the first pass of contrast through the pulmonary vasculature ( Fig. 2 ). This technique also uses 3D gradient-echo sequence and leverages acceleration approaches and optimized k-space sampling strategies to generate images with high spatial and temporal resolution, which can be viewed as a cine clip for qualitative assessment of pulmonary hemodynamics and also used to qualitatively or quantitatively assess lung parenchymal perfusion.
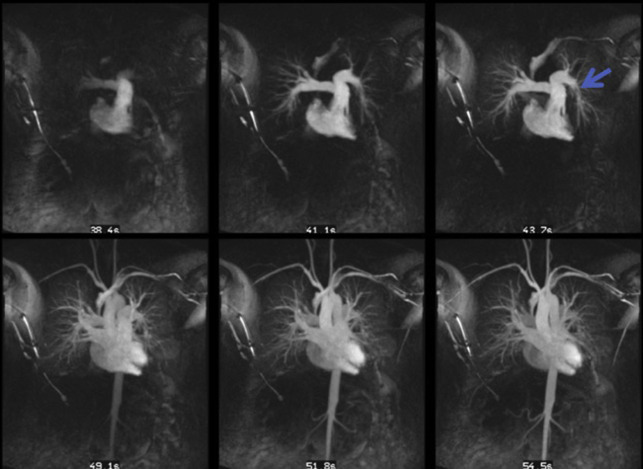
Artifacts associated with pulmonary magnetic resonance angiography
In addition to respiratory motion artifact, poor PA opacification caused by suboptimal contrast bolus timing and truncation (Gibbs ringing) artifact can reduce both image quality and diagnostic accuracy.
Pulmonary Artery Opacification
On contrast-enhanced pMRA, the high signal in the PA is caused by the T1-shortening effect of gadolinium-based contrast agent in the PAs. As described earlier, timing of imaging relative to arrival of the contrast bolus is critical to allow adequate opacification of the pulmonary tree. Challenges associated with timing are among the primary benefits of pMRA relative to CTA, because MRA allows multiple acquisitions without concerns related to radiation exposure, and delayed images may have improved PA opacification.
Two key parameters determine optimal contrast opacification: (1) image timing relative to bolus arrival, and (2) bolus length. For image timing relative to the contrast bolus, fluoroscopic triggering is used with imaging initiated when contrast is seen in the right ventricle or right ventricular outflow tract. Exact trigger timing may be site, scanner, and injector specific so feedback to the technologist regarding image quality related to triggering is important. Depending on k-space sampling strategies (standard cartesian vs elliptic centric), initiating the scan too early or too late can result in image blur or inappropriate edge enhancement ( Fig. 3 ). The narrower the bolus, the more difficult it becomes to align the contrast bolus timing with the selected k-space sampling scheme. Therefore, increasing the bolus duration by diluting the contrast with saline and injecting over a longer time period (∼20 seconds) allows more flexibility with contrast bolus timing with minimal impact on the overall signal in the PAs.
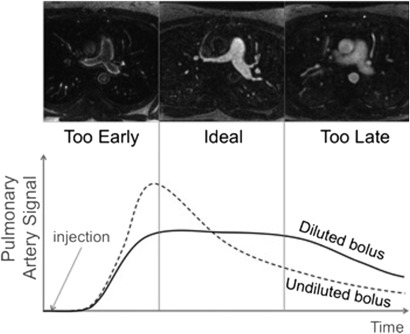
Truncation Artifact
Truncation (Gibbs) artifact is a well-known artifact in MR imaging that can lead to image interpretation challenges in pMRA, particularly when evaluating for PE. In MR imaging, high-spatial-frequency structures such as vessel edges are approximated by progressively higher frequency wave functions, and at sharp boundaries, such as a small vessel edge, infinite k-space sampling is required. Given the practical need to truncate higher spatial frequencies in k-space approximations of these edges, ripples of signal intensity can occur near sharp boundaries in reconstructed images. In larger anatomic structures such as the aorta or main PA, there is usually little problem generating adequate spatial frequencies to approximate the vessel walls without truncation artifact affecting signal in the vessel center. However, in small vessels, usually with intraluminal diameters on the order of 3 to 5 voxels, signal loss from destructive interference of the truncation artifact ripples can occur.
However, these artifacts can closely approximate true filling defects related to PE. Importantly, because the artifact is closely related to vessel size rather than contrast timing, it is likely to be present on all phases of images and therefore persistence of the filling defect on delayed series does not necessarily imply that the finding is a PE. A study by Bannas and colleagues showed that, by comparing the signal decrease in the central filling defect relative to surrounding blood, signal could be used to distinguish artifact from thrombus. A signal decrease of greater than 51% on pulmonary arterial-phase images or 47% on delayed-phase images resulted in a sensitivity of 100% and specificity of 90% for distinguishing PE from truncation artifact ( Fig. 4 ).
