The development of radiofrequency ablation for the treatment of atrial fibrillation has led to an increased interest in the accurate determination of pulmonary vein anatomy and left atrial fibrosis assessment to help plan the procedure and to monitor for postablation pulmonary vein stenosis. Contrast-enhanced magnetic resonance angiography (CE-MRA) readily demonstrates the pulmonary veins and is the method of choice for these imaging studies. In this chapter, we review the methods used to image the pulmonary veins and normal and anomalous pulmonary venous anatomy. We also describe how late gadolinium enhancement (LGE) imaging is used to identify atrial fibrosis and scar. The utility of cardiovascular magnetic resonance (CMR) before and after atrial fibrillation ablation is described.
Imaging Methods: Pulmonary Veins
The pulmonary veins can be identified by using standard anatomic and functional CMR imaging sequences. Although these methods are sufficient for identifying the anatomic relationship of the pulmonary veins to the heart and the other major vascular structures, CE-MRA is usually used for a volumetric three-dimensional (3D) understanding of the intricate pulmonary venous anatomy. A 3D spoiled gradient echo sequence is acquired during the first pass of gadolinium (Gd) contrast. Clinical protocols vary but have mainly common elements. The technique uses short repetition times (TR; 2–5 ms), a high flip angle (25–60 degrees), and fractional echoes, all of which provide T1 weighting and minimal flow artifacts. The spatial resolution varies from 1–2 × 1–2 mm in-plane with 2 to 4 mm slices, before interpolation. A single 3D volume requires a 10 to 20 second breath-hold to suppress ventilatory motion, but scan time can be shortened using smaller fields of view, shorter TRs, partial Fourier, lower spatial resolution, parallel imaging, or compressed sensing. Electrocardiogram (ECG) triggering is not employed, although it is recognized that the position and shape of the pulmonary veins changes throughout the cardiac cycle. Images obtained with this method reflect the pulmonary veins at their maximal size. Axial or coronal slabs are usually acquired, using either sequential or centric k -space filling. For the pulmonary vasculature, the arterial-venous transit time is very short (4–7 seconds) and therefore artery-vein separation is highly challenging and generally not targeted. Contrast is injected with a gadolinium dose of 0.1 to 0.2 mmol/kg at a rate of 1 to 2 mL/s, followed by a saline flush. A precontrast mask can be acquired, although mask subtraction is not essential for pulmonary venography because the background lung signal is very low. Often a second time frame is acquired immediately after the first-pass image to ensure acquisition during peak contrast. Timing of the acquisition to the first pass of contrast through the pulmonary veins is critical, and is achieved using either a bolus timing scan or with fluoroscopic triggering. For either method, imaging is timed to begin with the appearance of contrast in the left atrium (LA). Time-resolved imaging using view-sharing methods is also valuable, providing multiple 3D volumes during the passage of contrast.
More intravascular contrast agents (gadobenate dimeglumine, gadofosveset trisodium, or ferumoxytol) are available to extend the duration of shortened blood T1, and improve quality, but they may reduce the contrast between blood and scar/fibrosis, if LGE CMR is acquired.
Image Display
Once the 3D MRA dataset is obtained, the images can be transferred to a workstation for further manipulation and analysis ( Fig. 42.1 ). The simplest and often most informative is to dynamically view two-dimensional (2D) slices within the 3D dataset in the axial, coronal, and sagittal planes. The axial images usually provide a good overview of the pulmonary veins and their relationship to the LA, but the coronal and sagittal images are frequently required to determine specific anatomic findings, such as a left common or anomalous pulmonary vein.
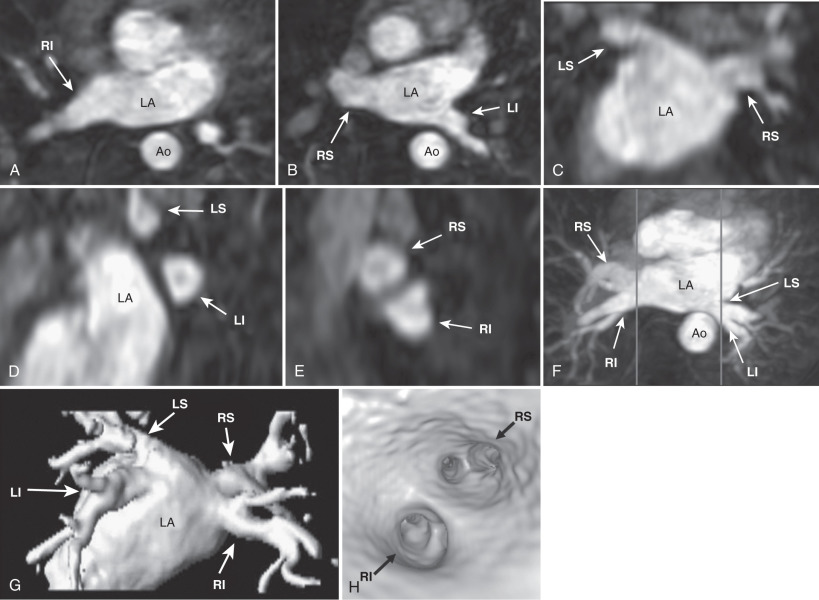
Although 2D slices are very useful for viewing the individual pulmonary veins, it is difficult to produce a single summary image of the anatomy. Maximal intensity projection (MIP) and 3D reconstructions displayed as shaded surface or volume-rendered images take full advantage of the 3D dataset and provide very good summary images. By convention, the LA and pulmonary veins are viewed in the posterior-anterior orientation. These 3D views are most useful when the displayed volume is limited to the LA and pulmonary veins. Because the aorta is directly posterior to the left-sided pulmonary vein, it frequently obscures them from view in the MIP images. Three-dimensional reconstructed images are frequently preferred because the aorta can be excluded from the displayed volume. Three-dimensional rendered images can also be rotated, to better appreciate the anatomy. Software is also available for generating an endovascular reconstruction, simulating the view of the pulmonary vein ostia from “inside” the LA. Direct anatomic measurements should not be obtained from these postprocessed images but from the 2D slices instead.
Pulmonary Vein Embryology
A clear understanding of pulmonary vein embryology is important for understanding both normal pulmonary vein anatomy, nonpathologic variations from the normal anatomy, and congenital anomalies. The pulmonary veins and associated apical LA are derived from the primitive common pulmonary vein. The primitive pulmonary venous system initially has no connection with the heart and drains into the cardinal veins and the umbilico vitelline system. At approximately the fourth week of gestation, the pulmonary venous drainage coalesces into a single vessel. At the same time, an outgrowth of the primitive LA extends toward the pulmonary venous system to meet this vessel to form the primitive common pulmonary vein and the venous connections to the cardinal veins and the umbilic vitelline system degenerate. The common pulmonary vein then expands to form the smooth-walled body of the LA, whereas the primitive LA forms the trabeculated left atrial appendage. The branches of the primitive common pulmonary vein form the adult pulmonary veins. The development of the LA and pulmonary veins is asymmetrical, with the two right-sided pulmonary veins developing first whereas the left-sided pulmonary venous drainage enters the LA through a single trunk that eventually bifurcates to form two veins.
Normal and Variant Pulmonary Venous Anatomy
Most commonly, there are four pulmonary veins that enter the LA: right superior, right inferior, left superior, and left inferior (see Fig. 42.1 ). Each of the veins is directed laterally, with the inferior veins directed posteriorly and the superior veins directed anteriorly. The left superior pulmonary vein frequently has a cranial angulation and may appear to arise from the superior portion of the LA.
Variant, nonpathologic pulmonary vein anatomy is very common, present in approximately 40% of patients. Although numerous variations have been described, the most common variations in the usual anatomy are a single left common pulmonary vein or an additional right middle pulmonary vein ( Fig. 42.2 ). These variations occur because of more or less incorporation of the primitive common pulmonary vein into the LA. Less incorporation leads to apparent fusion of pulmonary veins before entering the LA, whereas more incorporation results in additional pulmonary veins ( Fig. 42.3 ). Because the right-sided pulmonary veins form first and have more developmental time to be incorporated into the LA, it is more common to have additional veins on the right. Conversely, the left-sided pulmonary veins form later and are more likely to have a common trunk. These variations in pulmonary venous anatomy have not yet been identified as a cause of pathology.
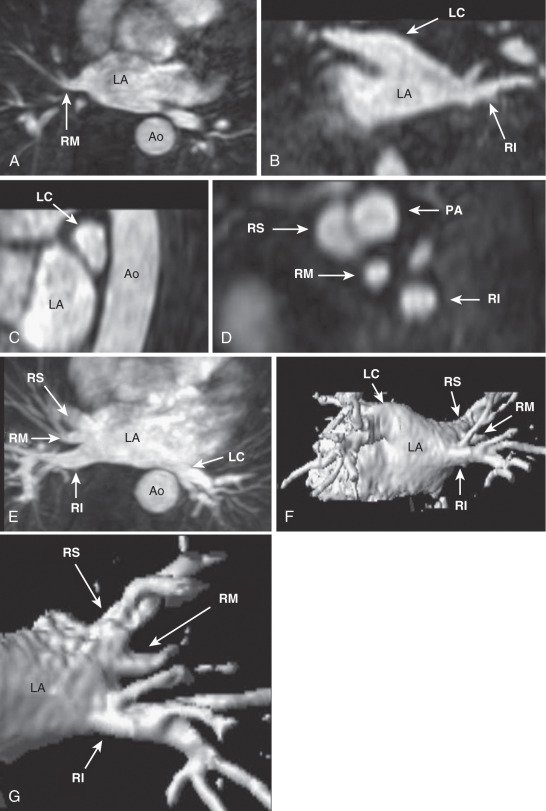
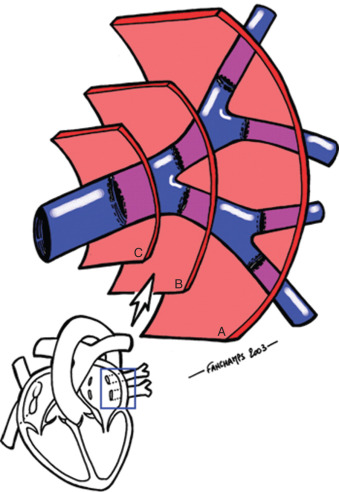
Congenital Pulmonary Venous Anomalies
Congenital pulmonary venous anomalies account for up to 3% of all congenital heart disease and approximately 2% of all deaths from congenital heart disease in the first year of life. The congenital anomalies that affect pulmonary veins are atresia, stenosis, and anomalous connections, which can be total or partial. These conditions occur when the normal connections of the primitive pulmonary venous system form abnormally or if embryologic connections to the cardinal vein or umbilic vitelline systems persist, and are frequently associated with other major congenital cardiac anomalies.
Anomalous pulmonary venous connections are the most common congenital anomaly. In total anomalous pulmonary venous connection, there is no connection of the pulmonary veins to the LA such that all of the pulmonary venous drainage enters the right atrium directly or via a systemic vein. This anomaly is necessarily associated with an atrial right-to-left shunt. Pulmonary venous hypertension is common because of twists in the artery or compression from adjacent vascular structures, whereas small atrial septal defects (ASDs) restrict systemic blood flow. These conditions results in cyanosis and heart failure. Although the mortality rate for symptomatic infants is 80% at 1 year, surgical repair is usually feasible and reduces the mortality rate to less than 25%. In partial anomalous pulmonary venous return, one or more pulmonary veins but not all enter the right atrium or a systemic vein. There is usually an associated ASD, frequently of the sinus venosus type with the right superior or right middle pulmonary veins draining into the superior vena cava. The physiology of this anomaly is similar to that of an ASD and depends on the magnitude of the left-to-right shunt and the presence of increased pulmonary vascular resistance. Patients are often asymptomatic if the shunt is relatively small and the pulmonary vascular resistance is normal. As a result, the diagnosis may not be made until adulthood. The scimitar syndrome, named after the characteristic chest radiograph finding, is a specific form of partial anomalous pulmonary venous connection in which all of the venous drainage from the right lung enters the inferior vena cava ( Fig. 42.4 ). This rare syndrome is also associated with anomalous arterial supply of the right lower lobe from the aorta, dextroposition of the heart, and hypoplasia of the right lung.
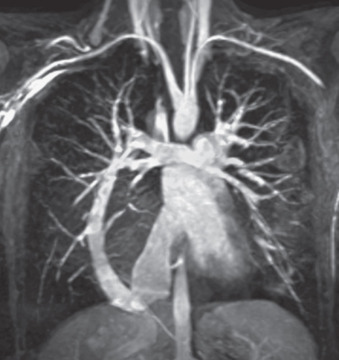
Congenital pulmonary vein atresia is defined as the absence of any connection of the pulmonary veins to either the LA or any other vascular structure. This is a very rare condition that is not compatible with life, although infants may survive for a short period of time because of small connections between the pulmonary veins and esophageal or brachial veins.
Congenital pulmonary vein stenosis can involve a focal segment of one or more pulmonary veins or more diffusely involve an entire pulmonary vein and is usually associated with other congenital cardiac malformations. Severe stenosis frequently results in cyanosis, heart failure, and death, although surgical repair is possible if only focal stenosis is present.
Imaging patients with congenital anomalies using CE-MRA is a valuable method for determining the pulmonary venous anatomy. It is generally able to identify all pulmonary venous anomalies, providing new information in 75% and identifying previously unsuspected anomalies in 30%.
Pulmonary Veins and the Pathophysiology of Atrial Fibrillation
Atrial fibrillation is the most common sustained cardiac arrhythmia, affecting more than 5 million people in the United States, and is a major cause of morbidity and mortality. It accounts for more than 400,000 hospitalizations each year and increasing the risk of death by 50% for men and 90% for women. Atrial fibrillation quintuples the risk of stroke and is the attributed cause for 15% of all strokes. The costs associated with the treatment of atrial fibrillation are estimated at US$6 billion. Although several antiarrhythmic drugs are available for the treatment of atrial fibrillation, maintenance of sinus rhythm is frequently suboptimal and all these drugs are associated with significant side effects or adverse events.
Increasingly, evidence has shown that the pulmonary veins play a critical role in the pathophysiology of atrial fibrillation. As noted, the pulmonary veins and LA are both derived from the primitive common pulmonary vein and therefore have many anatomic similarities. Both are smooth-walled structures that have electrically active myocardium. Approximately 90% of pulmonary veins contain atrial myocardium. Although the myocardium in the LA is uniform, myocardium in the pulmonary veins is frequently discontinuous and fibrotic. Patients with a history of atrial fibrillation uniformly have myocardium in the pulmonary veins and an increased rate of structural abnormalities. These structural abnormalities result in abnormal electrical activation, with slow and anisotropic conduction. Proarrhythmic re-entrant beats and sustained focal activity can be easily induced.
A landmark study demonstrated that the proarrhythmic electrical activity in pulmonary veins is directly responsible for the generation of atrial fibrillation in many patients. Among those with paroxysmal atrial fibrillation, 94% were found to have ectopic foci in the pulmonary veins that were responsible for the induction of atrial fibrillation. Radiofrequency ablation of these foci resulted in complete suppression of atrial fibrillation in a majority of patients.
Although these studies are suggestive that re-entry may be important in the initiation of atrial fibrillation, the mechanisms that sustain atrial fibrillation are still not known. Proposed mechanisms include triggers and an abnormal LA substrate. Triggers potentially include regions with ectopic electrical activity or atrial rotors. The presence of a dilated LA, by itself and with associated electrical, metabolic, and structural remodeling, is the abnormal substrate that may sustain atrial fibrillation.
Based on these findings, several related procedures were developed for the treatment of atrial fibrillation. Each of these procedures uses radiofrequency ablation to electrically isolate the pulmonary veins from the LA, with or without additional ablation in the body of the LA. Short-term success rates range from 65% to 85% in patients with paroxysmal atrial fibrillation, with a reduction in morbidity and improved quality of life.
Imaging Before and After Atrial Fibrillation Ablation
Imaging is usually performed before atrial fibrillation ablation to determine the pulmonary vein anatomy (size and number/orientation of the pulmonary veins). In addition, CE-MRA may be performed after the ablation if the patient has signs/symptoms suggestive of pulmonary vein stenosis (see later). The accurate determination of pulmonary vein anatomy is critical for the planning and execution of atrial fibrillation ablation. To achieve success, the operator must place a series of radiofrequency lesions that encircle the pulmonary veins and electrically isolate them from the LA. This necessarily requires that the pulmonary vein anatomy be determined before the procedure. In the initial development of the procedure the pulmonary veins were identified using invasive contrast venography. Although this can be done successfully, it greatly increases the procedure time and only provides projection images of the pulmonary veins. Most centers now use CE-MRA, noncontrast MRA, or computed tomography (CT) angiography to determine the pulmonary vein anatomy before the procedure. Either technique provides high-resolution 3D tomographic images of the pulmonary veins and other mediastinal structures. These images can also be imported into the 3D electrophysiologic mapping systems that are an integral part of the procedure to combine anatomic and functional information during the procedure to form an integrated image. Routine utilization of image integration has been associated with shorter procedure times and improved outcome after ablation for atrial fibrillation.
It is also important to identify the relationship of the pulmonary veins to other mediastinal structures to avoid complications during the procedure. The formation of an atrial-esophageal fistula is a rare but catastrophic complication caused by excessive heating of the posterior LA wall and the adjacent esophagus. The esophagus and its relationship to the LA can be readily identified on standard anatomic CMR sequences and on LGE ( Fig. 42.5 ). The esophagus almost always directly abuts the posterior LA and is usually closer to the left-sided pulmonary veins, but the location is highly variable. The esophagus is frequently within 5 mm of the pulmonary veins at a location that probably increases the risk for the formation of an atrial-esophageal fistula. Indeed, the esophagus often appears enhanced on postablation LGE. The risk of causing an atrial-esophageal fistula may be reduced by avoiding ablation in the region of the LA closest to the esophagus, but this may be difficult because the esophagus is mobile and may move during the course of the procedure.
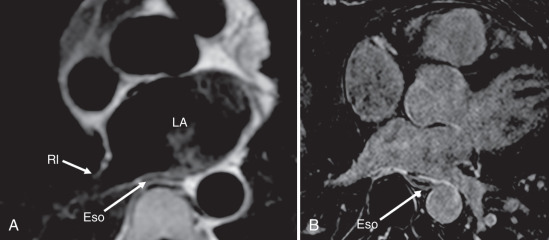
The pulmonary veins are sometimes imaged after the procedure if there is a suspicion for pulmonary vein stenosis. Pulmonary vein stenosis is an uncommon but severe complication of atrial fibrillation ablation ( Fig. 42.6 ). The application of radiofrequency energy to the pulmonary veins causes intimal proliferation and myocardial necrosis that can result in stenosis or occlusion. Severe stenosis occurs in up to 3% of patients after the procedure and results in pulmonary hypertension and decreased perfusion of the affected lung segments. Patients frequently present with cough or dyspnea, but a significant proportion are asymptomatic. Stenosis is most likely to occur in smaller pulmonary veins in which the ablation lesions were placed further into the pulmonary vein trunk and with greater extent of ablation. If stenosis does occur, pulmonary vein angioplasty is usually successful in restoring normal flow and alleviating symptoms. Techniques that have emphasized placing ablation lesions within the body of the LA under intracardiac echocardiographic guidance have dramatically reduced the rate of pulmonary vein stenosis such that screening for pulmonary vein stenosis after the procedure is no longer routinely performed.
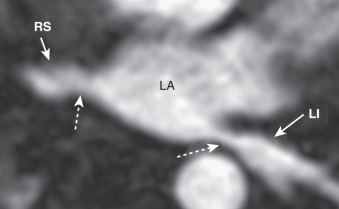
Quantification of Pulmonary Vein Size Before and After Ablation
The accurate measurement of pulmonary vein size is essential for serial assessment of pulmonary vein stenosis and to further investigate the role of pulmonary veins in the initiation and maintenance of atrial fibrillation. Most investigators have measured pulmonary vein diameters in a specified plane, usually at the ostia. These measurements tend to have poor reproducibility for several reasons ( Fig. 42.7 ). Identification of the true ostia is very difficult because the pulmonary veins and LA are embryologically related with no clear anatomic border between them. The pulmonary vein ostia are not round, such that measurements taken at the same location vary significantly with the plane of measurement. A further complication is that most measurements are derived from nongated images, although the pulmonary vein size varies significantly over the cardiac cycle. These difficulties were highlighted in a study comparing pulmonary vein diameter measurements performed using CT, intracardiac echocardiography, transesophageal echocardiography, and x-ray venography in the same patients. Each of these methods identified different numbers and positions of pulmonary veins, with a poor correlation between diameter measurements obtained with each imaging modality.
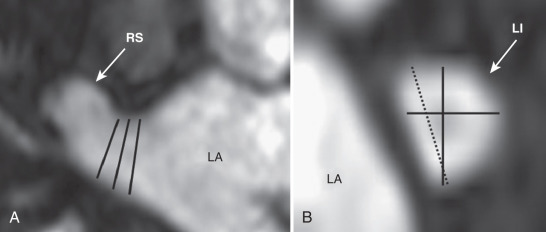
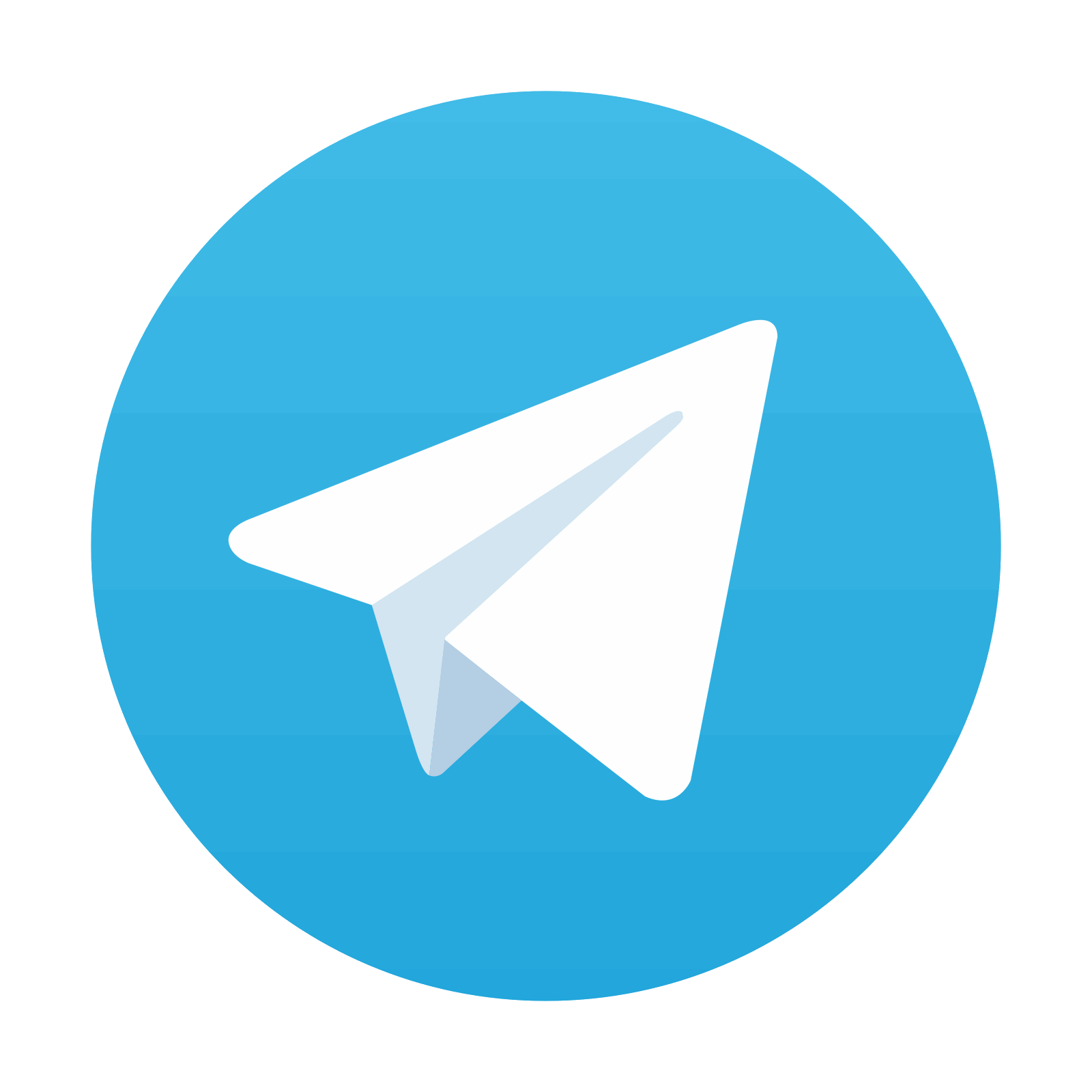
Stay updated, free articles. Join our Telegram channel

Full access? Get Clinical Tree
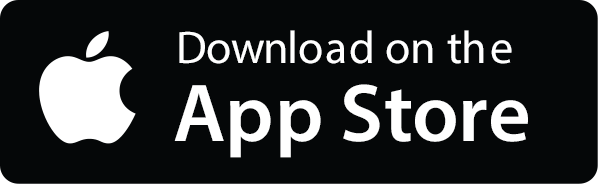
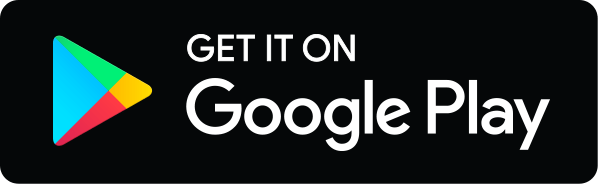
