A. Film dosimetry
–Film can be used to measure radiation doses received by radiation workers.
–Film sensitivity, however, depends on x-ray photon energy.
–Silver in film, which has a 25 keV K-shell binding energy, absorbs 30-keV photons very well but would absorb far fewer 300-keV photons.
–For the same air kerma, the blackening of film at 30 keV is much greater than for 300-keV photons.
–Film badges consist of a small case with a piece of film placed between different filters.
–Filters are little squares of Cu, Sn, Al, and plastic.
–The pattern of blackening behind the filters provides evidence of the energies of the photons responsible for the operator’s exposure.
–More uniform blackening behind the filters implies a higher photon energy.
–The film is processed and the optical density measured to estimate air kerma.
–The minimum air kerma film badges can detect is ~0.2 mGy.
–One advantage of film badges is that they provide a permanent record of operator dose.
–Film badges have limited accuracy because of their strong energy dependence.
–The accuracy of the reading can also be affected by heat and chemicals.
–For all these reasons, film badges have been largely replaced by alternative devices (e.g., thermoluminescent dosimeters [TLD]).
B. Thermoluminescent dosimeters
–Solid-state materials can store energy absorbed during x-ray exposure in electron traps.
–The stored energy is in the form of electrons trapped in high-energy imperfections in the crystal.
–In thermoluminescent dosimeters (TLDs), these energetic electrons are released by the application of heat.
–The released electrons result in the emission of visible light.
–Heating TLDs after exposure results in a light output that is proportional to the radiation air kerma incident on the material.
–Lithium fluoride (LiF) is the TLD used in diagnostic radiology because it simulates the absorption of x-rays by soft tissues.
–LiF has an atomic number (Z = 8.3), close to that of soft tissue (Z = 7.7), which makes it tissue equivalent.
–The response of TLD does not depend on photon energy, and similar signals would be obtained at both 30 keV and 300 keV (same air kerma).
–The energy response of LiF is thus far superior to that of film.
–TLDs materials are available that can measure doses as low as 0.01 mGy or as high as 10,000 mGy (10 Gy).
–TLDs are frequently used to measure patient doses during radiographic examinations and may be used for personnel dosimetry.
–The detection limit of a TLD used to monitor workers in radiology is ~0.2 mGy.
FIGURE 8.1 Schematic of the essential parts of ionization chambers that measure the electrons produced by x-rays interacting with air atoms.
C. Ionization chambers
–Ionization chambers detect ionizing radiation by measuring the charge (electrons) liberated when x-ray photons ionize the gas inside.
–Ionization chambers need a positive voltage at the collecting electrode (anode), which attracts the liberated electrons.
–The applied voltage should be high enough to collect all the liberated electrons.
–Figure 8.1 shows a schematic of the components of an ionization chamber.
–Charge liberated in the chamber is collected and used to determine the air kerma.
–Ionization chambers are accurate dosimetry devices.
–X-ray tube output is measured using ionization chambers.
–X-ray tube output is the air kerma (mGy/mAs) at a specified distance and voltage.
–A typical x-ray tube output is ~0.1 mGy/mAs at a distance of 1 m (80 kV).
–Ionization chambers are not very sensitive, and would be useless for detecting small amounts of radioactive contamination in a nuclear medicine (NM) department.
D. Geiger counters
–A Geiger counter is an ionization chamber with a very high voltage across the chamber.
–An incident photon interacting in this chamber produces a small number of free electrons.
–These electrons are accelerated by the large positive voltage and gain energy.
–These energetic electrons will cause more electrons to be ejected from gas atoms in the chamber, which are further accelerated and produce even more electrons.
–As a result, there is an electron avalanche corresponding to a large amplification of the initial charge liberated by the incident electron.
–The large amplified output results in the “click” heard when using a Geiger counter.
–Quenching gases are added to Geiger counters to improve stability.
–Geiger counters are sensitive and are used to detect low levels of radioactive contamination.
–Geiger counters are far too sensitive to measure diagnostic x-ray beams.
–The number of photons in x-ray beams is ~107 photons/mm2 (i.e., huge).
–Geiger counters cannot differentiate between different types of radiation.
–Any beta particle or individual photon results in the same signal (electron avalanche).
–Geiger counters are not accurate radiation dosimeters.
–A Geiger counter provides “counts per minute”, not mGy/minute.
E. Pocket dosimeters
–Pocket dosimeters are ionization chambers that look like large pens.
–A typical analog dosimeter uses a positively charged quartz fiber suspended in an air-filled chamber.
–X-rays incident on the chamber will produce ions that neutralize the charge and cause the fiber to move.
–The x-ray photon energy must exceed 20 keV to penetrate the wall of the dosimeter.
–The typical range of a pocket ionization chamber is 0 to 2 mGy.
–Pocket ionization chambers are also available that work up to 50 mGy.
–Pocket ionization chambers are easily recharged and reused.
–Pocket ionization chambers are frail and may be damaged if dropped on the floor.
–Digital pocket dosimeters can be obtained that use radiation-sensitive diodes coupled to solid-state electrons to measure and display the dose.
–The principal benefit of pocket dosimeters is that they provide immediate readings.
–Pocket dosimeters are used to assess the dose to a parent who holds a child or infant during a radiologic examination.
–Doses to parents holding children will be low (scatter radiation is 0.1% of the entrance air kerma at 1 m).
–Pocket ionization chambers can confirm low doses, and reassure parents who hold children.
A. Organizations
–The International Commission on Radiological Protection (ICRP) was founded in 1928 and issues periodic recommendations on radiation protection.
–ICRP Publication 103 (2007) is the latest publication from the ICRP that provides recommendations for radiation workers, patients, and members of the public.
–ICRP Publication 103 replaces Publication 60 (1990).
–The International Commission on Radiological Units and Measurements (ICRU) advises on issues such as measurement units in radiology.
–The ICRU was responsible for replacing exposure (R) with air kerma (Gy).
–In the United States, the foremost radiation protection body is the National Council on Radiation Protection and Measurements (NCRP).
–The NCRP advises federal and state regulators on radiation protection.
–In the United States, the federal government is primarily responsible for regulating radioactive materials.
–The Nuclear Regulatory Commission (NRC) is responsible for the rules and regulations regarding nuclear materials.
–Specific rules and regulations are compiled in Parts 19 and 20 in Chapter 10 of the Code of Federal Regulation (CFR).
–Some states are known as agreement states and arrange with the NRC to self-regulate medically related licensing and inspection requirements for nuclear materials.
–Other states (i.e., non–agreement states) are regulated directly by the NRC.
–U.S. states are responsible for regulating x-ray emitting devices.
–States coordinate their x-ray protection activities through the Conference of Radiation Control Program Directors (CRCPD), which meets annually.
B. Occupational (whole body)
–Occupational dose limits exclude doses from medical procedures and natural background radiation.
–The ICRP recommends an effective dose limit of 20 mSv per year, when averaged over 5 years.
–The NCRP recommends a lifetime cumulative effective dose limit of ten times the individual’s age (mSv).
–Both ICRP and NCRP limit occupational exposure in any year to 50 mSv.
–ICRP and NCRP philosophy is to balance operational flexibility with an adequate level of safety over a longer time frame.
–In the United States, the regulatory (i.e., legal) effective dose limit for radiation workers is 50 mSv/year.
–Regulatory dose limits are similar at the federal and state levels.
–Federal laws generally apply to exposures from radioactive materials (NM), whereas state regulations cover exposures from x-ray devices including CT.
–The most highly exposed workers are unlikely to receive regular annual effective doses >~5 mSv (2008).
–People who are occupationally exposed to radiation should be monitored using personnel dosimeter such as film badges or TLDs (see Section I).
–Emergency occupational exposures can exceed these dose limits if lifesaving actions are involved.
–Older workers with low lifetime accumulated effective doses should volunteer for emergencies where high exposures are expected (up to 500 mSv).
–If an emergency exposure exceeds 500 mSv, possible acute and long-term risks need to be addressed.
C. Public
–Dose limits for members of the public are much lower than those for occupational exposure.
–The ICRP recommends a whole-body dose limit for members of the public of 1 mSv/year.
–ICRP Publication 103 (2007) maintained the public dose limit set in ICRP Publication 60 (1990).
–ICRP dose limits may be exceeded in any 1 year provided the 5-year average dose does not exceed 1 mSv/year.
–In the United States, the regulatory dose limit for members of the public is 1 mSv/year (2008).
–X-ray facilities must be designed to ensure that exposure to members of the public does not exceed 1 mSv/year.
–Dose limits to members of the public exclude natural background radiation.
–For regulatory purposes, medical doses are also excluded when determining doses for a member of the public.
–Exclusion of medical x-rays is justified because diagnostic information from radiologic examination will confer a benefit to the exposed individual.
–Public exposures from radiologic activities are normally negligible.
D. Pregnant workers
–The ICRP recommends a limit of 1 mSv from declaration of a pregnancy by a radiation worker to the subsequent birth of a child.
–The ICRP considers the fetus to be a member of the public for radiation protection purposes.
–Once a pregnancy is declared, the NCRP recommends a monthly limit of 0.5 mSv to the embryo or fetus of a radiation worker.
–The limitation on the rate at which the fetus is exposed helps ensure that any radiation risks to the fetus are kept to a minimum.
–In the United States, the regulatory dose limit for the fetus of a radiation worker is 0.5 mSv/month, which implies a total dose limit of 5 mSv.
–This fetal dose limit is higher than that of members of the public (1 mSv).
–This higher fetus legal dose limit permits women of reproductive capacity to seek employment as radiation workers (e.g., nuclear medicine technologists).
–Setting the fetal dose limit at 1 mSv would have deprived women of reproductive capacity employment as radiation workers.
–Pregnant radiation workers are monitored by a dosimeter worn on the abdomen to ensure fetal dose limits are not exceeded.
–Figure 8.2 shows a pregnant radiation worker wearing two dosimeters, one (A) worn outside the lead apron, and the other (B) that is worn under the lead apron.
–Dosimeter A is used to estimate the worker effective dose and dosimeter B is used to estimate the embryo/fetus dose.
–The dose to the fetus may be taken to be half the skin dose to account for attenuation by soft tissues between the fetus and skin surface.
E. Miscellaneous
–The dose limit to the eye lens of an occupational worker is 150 mSv per year.
–The special eye lens dose limit is to prevent the induction of eye cataracts over a working lifetime.
–The dose limit to the skin of a radiation worker is 500 mSv per year.
–Skin doses are to be averaged over the most highly exposed 1 cm2.
–The skin dose limit is designed to prevent the induction of deterministic effects.
–Radiation therapy shows that skin tolerates fractionated doses of 20 Sv (20 Gy); 20 Sv divided by 40 working years corresponds to 500 mSv/year.
–The dose limit for the hands and feet of radiation workers is also 500 mSv/year.
–Dose limits to members of the public are 15 mSv/year for the eye lens and 50 mSv/year for skin.
–Dose limits for member of the public have historically been 1/10th of those of radiation workers.
–Public dose limits are conservative to account for the possibility of multiple sources of exposure.
FIGURE 8.2 Monitoring the doses to a pregnant radiation worker.
A. Protection in radiology
–One important objective of radiation protection is to prevent significant deterministic effects.
–Deterministic effects can be prevented by keeping doses below the threshold dose of ~2 Gy.
–A second objective of protection practice is to minimize stochastic risk (cancer and hereditary effects).
–Minimization of stochastic risks needs to be reasonable and take into account any benefits gained by the radiation workers.
–Personnel monitoring devices are worn to ensure that workers receive doses below the appropriate dose limit and to monitor radiation safety practices.
–X-ray personnel receive significant exposures only when they are standing close to the patient undergoing the x-ray examination (i.e., in the same room).
–Sources of exposure to x-ray personnel include patient scatter and leakage radiation from the x-ray tube.
–As a general rule, the scatter dose level from patients at 1 m is ~0.1% of the entrance skin dose.
–Table 8.1 illustrates the scatter radiation exposures for common radiologic examinations.
–Leakage radiation from the tube housing is very low.
–Regulatory limits specify that leakage radiation must be <1 mGy/hour at a distance of 1 m.
B. Lead aprons
–Lead is an effective protective barrier with a very high attenuation coefficient.
TABLE 8.1 Representative Scatter Radiation Air Kerma Levels in Radiology
–Attenuation of x-rays by lead is high because of its high density and high atomic number.
–The half-value layer of lead is ~0.1 mm at 60 kV, ~0.2 mm at 80 kV, and ~0.3 mm at 130 kV.
–The tenth-value layer of lead is ~0.35 mm at 60 kV, ~0.65 mm at 80 kV, and ~0.9 mm at 130 kV.
–Lead aprons used in diagnostic radiology are generally constructed from lead-impregnated vinyl.
–Tin impregnation may also be used as an alternative to lead, as it has a more appropriate K-absorption edge.
–Lead aprons used in diagnostic radiology should have 0.25 mm or 0.5 mm equivalents of lead.
–A lead apron with 0.5 mm lead equivalence weighs about 5kg (10 lb).
–A 0.5-mm lead-equivalent apron attenuates most of the x-ray beam in diagnostic radiology and should always be worn when working close to patients being irradiated.
–Lead aprons attenuate at least 90% of most x-ray beams.
–Individual organs not protected by lead aprons may receive much higher doses during fluoroscopy.
–Lead aprons should be stored on appropriate racks, as folding them can produce cracks.
–Lead aprons need to be tested annually by fluoroscopy to ensure that they do not contain any cracks.
C. Room shielding
–The design of barriers in radiology departments depends on the workload (W), which is how often the machine is in operation (mA-minute/week).
–The workload can be combined with the x-ray tube output (mGy/mAs) to determine the radiation intensity at the patient location.
–The source to the barrier distance is used to estimate exposures outside the x-ray facilities by means of the inverse square law.
–Room shielding also depends on the use factor and occupancy factor.
–The use factor (U) is the fraction of time that radiation points toward a specific barrier.
–For primary barriers, the use factor is 1 for the floor, 1/16 for the walls, and 0 for the ceiling.
–The occupancy factor (T) is fraction of time people work on the other side of the barrier.
–Occupancy factors are 1 for offices and laboratories, 1/5 for corridors and employee lounges, and 1/20 for restrooms and storage areas.
–Primary protective barriers absorb primary radiation.
–Secondary barriers protect workers from scattered and leakage radiation.
–In practice, the shielding used for most x-ray installations is 1.6 mm (1/16 inch) of lead in the walls.
–X-ray facilities need to ensure that there are no gaps between doors and that shielding extends at least 2 m from the floor.
–Figure 8.3 shows a schematic of an x-ray facility.
D. Operator doses
–Shielding of x-ray rooms and booths housing the x-ray controls offers a high degree of attenuation of the x-ray beams.
–As a result, most x-ray technologists receive relatively low effective doses.
–Doses to 90% of x-ray technologists will be below the detection limit of the radiation badges used.
–Significant doses to operators occur when operators are in the room when the x-ray beam is activated.
–The most likely sources of operator doses in diagnostic radiology are fluoroscopy examinations and interventional radiology.
–Radiologists and technologists who routinely work inside x-ray rooms
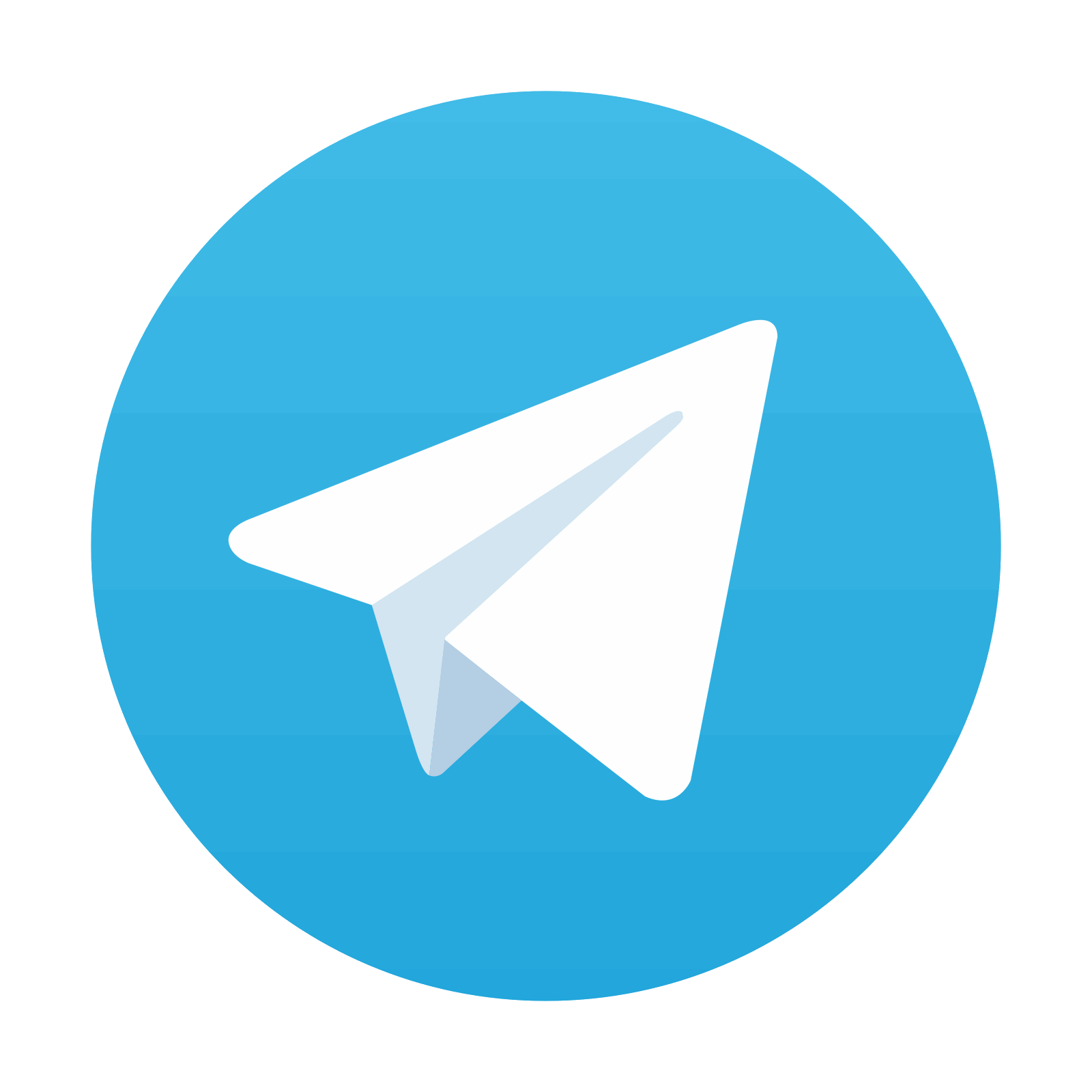
Stay updated, free articles. Join our Telegram channel

Full access? Get Clinical Tree
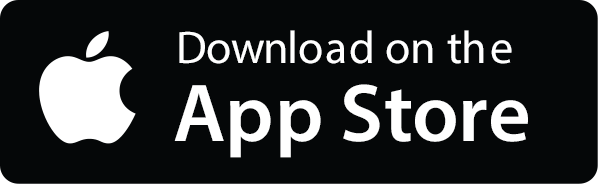
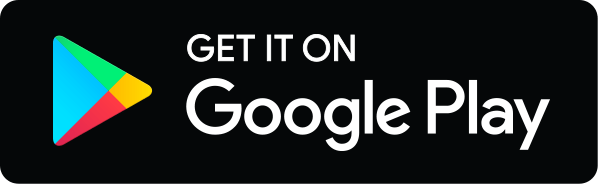