RADIOBIOLOGY/ PATIENT DOSIMETRY
A. Energy transfer
–Ionizing radiation transfers energy to electrons in the absorbing medium.
–Interacting x-rays produce energetic recoil Compton electrons and photon electrons.
–Figure 7.1 illustrates the fate of an energetic photoelectron, which results in a large number of additional ionization events.
–Ionizing radiations have sufficient energy to break apart chemical bonds.
–Direct action occurs when Compton/photoelectrons directly ionize a target molecule.
–Indirect action occurs when Compton/photoelectrons interact with water to produce a (free) hydroxyl radical.
–Free radicals are chemically reactive molecules with unpaired electrons produced by ionizing radiation.
–Hydroxyl radicals exist long enough to diffuse to, and damage, target molecules.
–About two thirds of the biologic damage by x-rays is caused by indirect action and the remaining one third by direct action.
–The physics and chemistry are very rapid, and occur in less than a millisecond.
–Energy deposited in a cell can damage biologically important molecules (e.g., DNA).
–The DNA molecule carries the code needed for cell metabolism and is duplicated when cells divide.
–Radiation may damage a DNA molecule, possibly causing cell death, somatic damage, or a mutation that results in hereditary effects.
B. Cells
–Radiobiology is the study of the effects of ionizing radiation in cells and animal models.
–Cell cycles for mammalian cells include mitosis (M), G1, DNA synthesis (S), and G2.
–Cells are generally most sensitive in M and G1 and most resistant during S.
–Energy from x-rays is deposited unevenly and produces double-strand DNA breaks.
–Chromosome breaks and aberrations are examples of biologic damage caused by radiation.
–Double-strand breaks are important factors for cell death, carcinogenesis, and mutations.
–By contrast, single-strand breaks are much more likely to be repaired.
–Health consequences of cell death occur on a time scale measured in hours and weeks.
–A small amount of energy deposited in a cell may cause cell function to be modified.
–Damaged somatic cells can result in the induction of cancer (fatal and nonfatal).
–Induction of cancer by radiation takes years and decades to develop.
–Damaged sperm and eggs (i.e., germ cells) can result in hereditary effects (mutations).
–Hereditary effects are sometimes called genetic effects.
–Changes in the genetic code of a germ cell can affect future generations.
C. Cell sensitivity
–The amount of biologic damage produced depends on the total amount of energy deposited in a cell or tissue (i.e., absorbed dose).
FIGURE 7.1 Fate of an energetic Compton electron showing some of the hundreds of ionization events this electron will produce.
–A plot of the surviving fraction of cells versus radiation dose is called a cell survival curve.
–Cell survival curves for x-ray exposures are not straight lines, but are curved.
–Cells may die attempting to divide (mitotic death).
–Programmed cell death may also occur (apoptotic death).
–For a constant total dose, reduced dose rates generally reduce cell killing.
–Sublethal damage repair can occur when the radiation delivery is protracted.
–The radiobiologic LD50 is the lethal dose that will kill 50% of irradiated cells.
–LD50 values are generally several Gy, much higher than most doses encountered in diagnostic radiology.
–Mammalian cells are more radiosensitive than bacteria, because they have a larger amount of DNA.
–Rapidly proliferating cells (e.g., bone marrow stem cells) are most sensitive.
–Highly differentiated and/or nonproliferating cells (e.g., nerve cells) are least sensitive.
–Oxygen influences the biologic effect of x-rays.
–The oxygen enhancement ratio (OER) is (doses of hypoxic irradiation)/(dose of aerated irradiation) that produces the same amount of biologic damage.
–The OER for x-rays is between 2 and 3.
–This means that oxygenated cells are therefore two to three times more sensitive when irradiated by x-rays than anoxic cells.
D. Linear energy transfer (LET) and relative biologic effectiveness (RBE)
–Linear energy transfer (LET) is the energy transferred per unit length of track.
–LETs for x-rays are ~1 keV/μm.
–X-and gamma-rays are said to be sparsely ionizing, and ionizing events from x-ray interactions are well separated.
–Alpha particle LETs are ~100 keV/μm.
–Alpha particles are said to be densely ionizing, and ionizing events from alpha particles are close together.
–Radiobiologists use the term relative biologic effectiveness (RBE) to compare the ability of different types of radiation to cause biologic damage.
–Relative biologic effectiveness (RBE) of some test radiation is the ratio D250/Dtest, in which D250 is the dose of 250-kV x-rays and Dtest is the dose from the test radiation.
–RBE pertains to a specified biologic end point (e.g., surviving fraction of 50%).
–For the same absorbed dose, high LET radiations produce more biologic damage than low LET radiation.
–RBE is close to unity for low LET (~1 keV/μm) radiation.
–RBE increases with LET to a maximum at ~100 keV/μm.
–High LET radiations can have RBE values up to eight times higher than x-rays (i.e., low LET radiation).
–Biologic effects of radiation depend on both total energy absorbed (i.e., dose), and radiation type (i.e., LET).
E. Equivalent dose
–Equivalent dose (H) quantifies biologic damage by different types of radiation.
–High LET radiation (alpha particles) causes more biologic damage than low LET radiation (x-rays and beta particles).
–The equivalent dose is the absorbed dose (D) multiplied by the radiation weighting factor (wR) of the radiation, or H = D × wR.
–For radiologic protection purposes, wR permits comparisons of effects of different types of radiation on a common scale.
–The radiation weighting factor wR depends on the radiation LET.
–For low LET radiation sources (e.g., electrons, x-rays, gamma rays), wR is 1.
–For high LET radiation sources (e.g., alpha particles), wR may be as high as 20.
–Equivalent dose is expressed in sievert (Sv).
–In diagnostic radiology and nuclear medicine, x-rays, gamma rays, and beta particles have a radiation weighting factor wR equal to 1.
–1 Gy of x-rays corresponds to an equivalent dose of 1 Sv.
–1 mGy of x-rays corresponds to an equivalent dose of 1 mSv.
–High LET radiations have high wR values.
–Alpha particles have wR equal to 20.
–Neutrons can also have a wR value as high as 20.
–An absorbed dose of 1 Gy from alpha particles corresponds to an equivalent dose of 20 Sv.
–Equivalent dose is primarily used for radiation protection purposes (see Chapter 8).
–Equivalent doses are approximate indicators of potential biologic harm.
–Biologic effects of radiation are best assessed taking into account type of radiation, as well as any temporal and spatial patterns of dose distribution.
A. Whole-body irradiation
–Lethal doses are normally associated with (approximately) uniform whole-body exposures.
–LD50 is the uniform whole-body dose that would kill half (50%) the population.
–The LD50 is 3 to 4 Gy for young adults without medical intervention.
–LD50 is likely to be lower for children, and older individuals.
–Symptoms at doses of ~LD50 include anorexia, nausea, and vomiting.
–A whole-body dose of ~100 Gy will kill in 1 to 2 days from permeability changes in the brain blood vessels (i.e., cerebrovascular syndrome).
–Fatal whole-body doses are associated with symptoms of diarrhea and low blood pressure.
–A whole-body dose of ~10 Gy kills in 5 to 10 days due to loss of epithelial lining of the gastrointestinal tract (i.e., GI syndrome).
–A whole-body dose of ~2 to 5 Gy sterilizes dividing precursor stem cells, which reduces circulating blood elements within 2 or 3 weeks (i.e., hematopoietic syndrome).
–Lethal doses of radiation are rare and occur during catastrophic accidents such as the Chernobyl Nuclear Power plant accident in Ukraine (1986).
–About 30 fire fighters are reported to have been killed during the Chernobyl accident because of their high doses of radiation.
B. Deterministic effects
–Radiation doses in diagnostic radiology are generally nonuniform.
–The magnitude of the localized (i.e., organ) dose is used to predict the effect of the radiation delivered to this organ.
–A localized dose to a region has effects that are very different from the same dose delivered uniformly to the whole body.
–Five Gy to a toenail is of no clinical consequence, but 5 Gy to the whole body could be lethal.
–High organ doses may result in deterministic radiation effects.
–A deterministic effect has a threshold dose, below which the effect does not occur.
–When the threshold dose is exceeded, deterministic effects are possible.
–Deterministic effects are also called harmful tissue reactions.
–Radiation-induced skin damage is the most common example of a deterministic effect.
–Deterministic effects also include cataract induction and induction of sterility.
–Deterministic effects are mainly a result of cell killing.
–Severities of deterministic effects increase with dose.
–The practical threshold dose for use in diagnostic radiology is 2 Gy.
–Below 2 Gy, clinically significant deterministic effects are most unlikely.
–Above 2 Gy, deterministic effects are possible, and the patient should be monitored for such a possibility.
–At doses well above 2 Gy, deterministic effects are likely to occur.
C. Skin reactions
–The most common deterministic effect in diagnostic radiology is damaged skin.
–Highest skin doses occur where the x-ray beam enters the patient.
–For skin doses >~2 Gy, transient erythema may occur in a matter of hours.
–Skin doses of ~6 Gy produce erythema 1 to 2 weeks following the exposure.
–Skin doses that exceed 10 Gy can produce dry desquamation.
–Dry desquamation arises from the loss of clonogenic skin cells.
–Moist desquamation occurs at skin doses >15 Gy.
–Skin effects are reversible if the population of basal cells can recover.
–Epilation is another deterministic effect that can occur in diagnostic radiology.
–At doses <~3 Gy, there will be no epilation.
–For skin doses of 3 to 5 Gy, temporary epilation can occur.
–The onset of epilation occurs after 2 to 3 weeks.
–Hair that grows after a radiation-induced epilation may be of a different color (i.e., gray).
–At doses >~7 Gy, epilation can be permanent.
–Figure 7.2 depicts how radiation affects skin erythema and hair loss.
D. Cataractogenesis
–Cataracts are opacifications of the eye lens that is normally transparent.
–The eye lens has no method of removing dead or damaged cells.
FIGURE 7.2 Idealized probability of inducing two deterministic effects (skin erythema and hair loss) as a function of the absorbed dose to skin.
–The induction of cataracts is an important deterministic effect of ionizing radiation.
–Cataracts are a late effect of radiation.
–Cataracts that appear at the posterior pole of the lens of exposed individuals would suggest that the cataract has been caused by radiation.
–Cataract induction is a possibility for patients undergoing lengthy interventional procedures.
–There is evidence of early cataracts in astronauts.
–Cataract formation is dependent on the total dose and on the time over which this dose is delivered.
–An acute dose of ~2 Gy is required to produce a cataract.
–For chronic exposure, the threshold dose for radiation-induced cataracts is ~5 Gy.
–There is a latent period between irradiation and the subsequent appearance of cataracts.
–Latency periods for cataractogenesis are reported as ~8 years after eye lens doses of a few Gy.
–Neutrons are especially effective in causing cataracts.
–Neutron RBE values are between 10 and 50, depending on the dose level.
E. Sterility
–In the male, low doses of ~0.2 Gy can produce a diminished sperm count.
–Doses above 0.5 Gy can result in azoospermia (temporary sterility).
–Recovery time from temporary sterility depends on dose.
–Sterility requires a single dose of ~6 Gy in men.
–In males, fractionated exposure to the gonads produces more damage than acute exposure.
–Permanent sterility can result from ~3 Gy fractionated over a few weeks.
–In the female, radiation can induce permanent ovarian failure.
–The dose for female sterility is highly dependent on age.
–The dose required for permanent sterility in the female ovaries is reported to be as high as 12 Gy in prepuberty.
–Female permanent sterility results from a dose of ~2 Gy for premenopausal women.
A. Stochastic risks
–Carcinogenesis is the main concern following doses of ionizing radiations below the threshold for induction of deterministic effects (i.e., <2 Gy).
–Carcinogenesis is a stochastic effect of radiation, meaning random or probabilistic.
–The severity of radiation-induced stochastic effects is independent of the radiation dose.
–The radiation dose affects only the probability of the stochastic effect occurring.
–Radiation induces both benign and malignant tumors.
–As dose increases, the chance of a stochastic effect increases.
–For radiation protection purposes, stochastic effects have no threshold.
–In addition to carcinogenesis, the other radiation-induced stochastic risk is the induction of hereditary effects.
–Stochastic risks are dependent on sex and age at exposure.
–Radiation-induced thyroid cancer is more likely in children and women than in men.
–Carcinogenesis is the principal radiation concern in diagnostic radiology.
–Radiation-induced malignancies are similar to natural malignancies of the same type, and appear at similar ages.
–Bone marrow, colon, lung, female breast, stomach, and childhood thyroid are the organs that are most susceptible to radiation-induced malignancy.
–Bladder, liver, and esophagus are moderately radiosensitive.
–Minimizing patient radiation doses in diagnostic radiology is very important because this minimizes stochastic radiation risks.
B. Epidemiologic studies (medical)
–Epidemiologic studies of radiation-induced carcinogenesis require large cohort size(s) and adequate control group(s).
–Long follow-up periods (decades) are essential for observing solid tumors.
–Leukemia has been observed in patients irradiated for ankylosing spondylitis.
–Radiation induces acute and chronic myeloid leukemia but not chronic lymphocytic leukemia.
–Radiation-induced thyroid cancer has been observed in children irradiated to treat enlarged thymus or diseases of the nasopharynx and tonsils.
–Thyroid cancers have also been observed in children where radiation was used to treat acne, tonsillitis, tinea capitis (ringworm), and cancer.
–Breast cancer appears in patients treated with x-rays for postpartum mastitis.
–Patients fluoroscoped repeatedly during the management of tuberculosis have also shown an elevated incidence of breast cancer.
–Two independent studies in Nova Scotia and Massachusetts have shown similar results of increased female breast cancer.
–A significant excess of cancers has been observed following radiotherapy for Hodgkin lymphoma, prostate cancer, and carcinoma of the cervix.
–Excess cancers have been observed following radiation therapy for breast cancer and carcinoma of the testes.
–Secondary cancers have also been seen following radiation therapy for childhood malignancies.
–Bone cancers have been observed in patients who had injections of radium for tuberculosis or ankylosing spondylitis.
C. A-bomb survivors/radiation workers
–Detrimental effects of radiation have been studied in atomic bomb survivors and radiation workers.
–The largest group studied for radiation-induced cancer is the survivors of the atomic bomb survivors of Hiroshima and Nagasaki.
–Excess cancer deaths depend on dose, age at exposure, time since exposure, and gender.
–For solid tumors, the excess cancer incidence was found to be a linear function of dose.
–Leukemia data were best fitted by a linear quadratic function of dose.
–Lung cancer has been observed in uranium miners who were exposed to radon and radon daughter products.
–Excesses of lung cancer have been observed in miners in Colorado (U.S.), uranium mines in Czechoslovakia, fluorspar mines in Newfoundland (Canada), and Swedish mines (nonuranium).
–
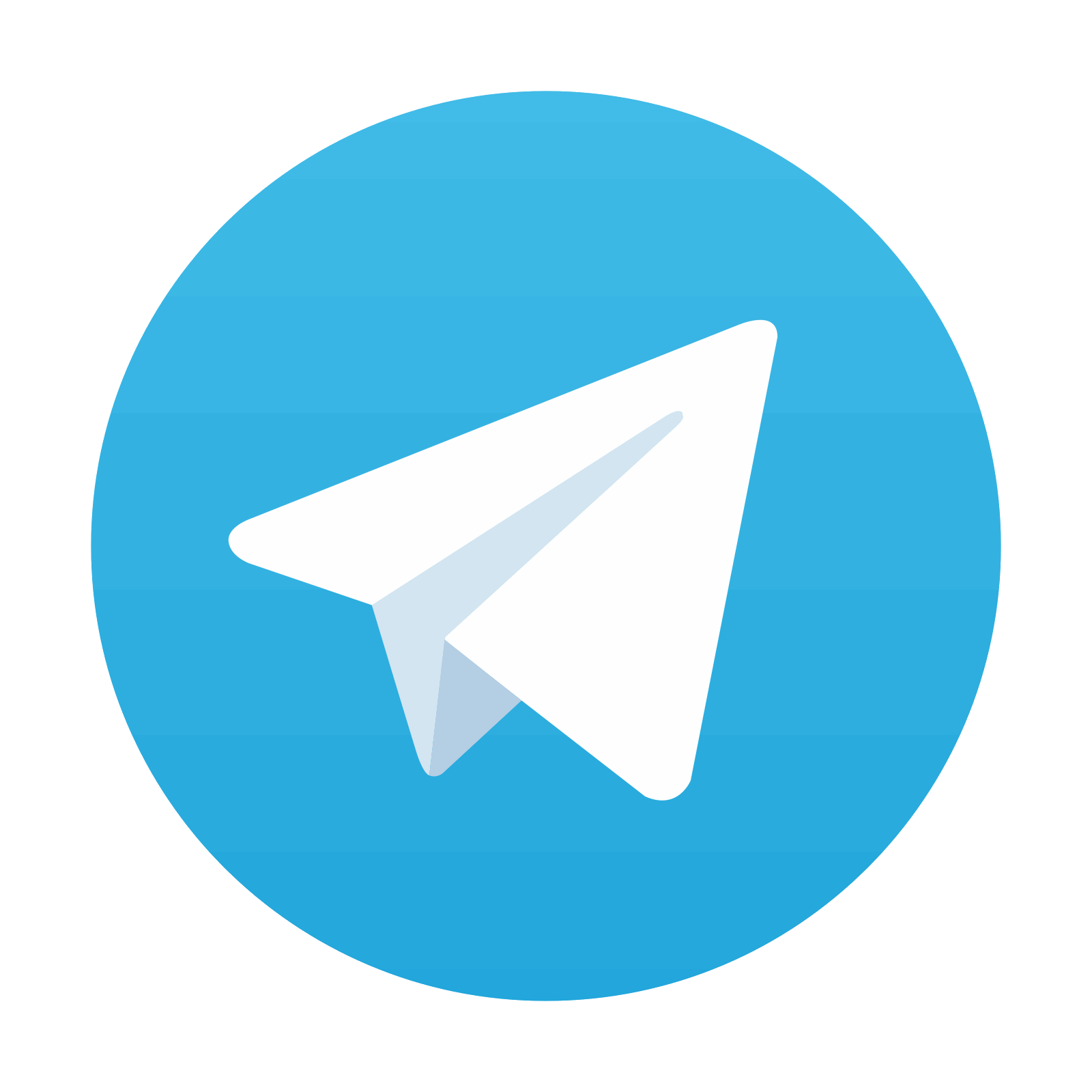
Stay updated, free articles. Join our Telegram channel

Full access? Get Clinical Tree
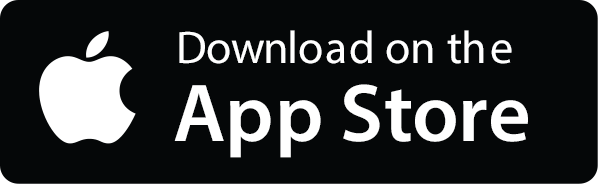
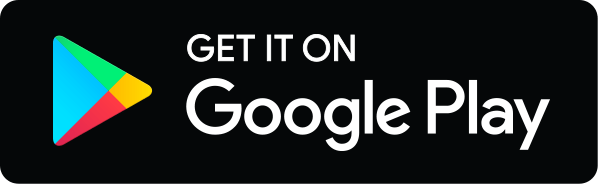