Fig. 50.1
Axial 2-deoxy-2(18F)-fluoro-d-glucose PET scan in a child with intractable temporal lobe epilepsy and normal MRI. EEG changes were occasionally bilateral or equivocal. PET scan showed hypometabolism in the left medial temporal (arrow) and increased the confidence for epilepsy surgery. The child underwent surgery, which showed mild sclerotic changes in the medial temporal lobe on histopathology, and is seizure-free after the surgery
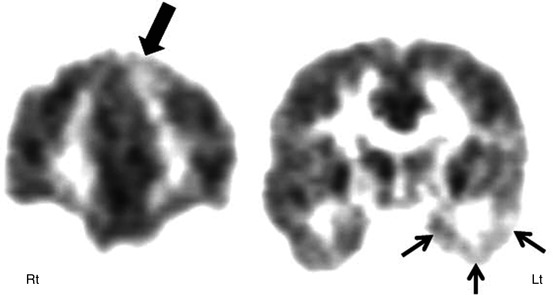
Fig. 50.2
2-Deoxy-2(18F)-fluoro-d-glucose PET in a child with dual pathology. Coronal PET showing an area of decreased glucose metabolism in the left medial frontal cortex (thick arrow), in addition to hypometabolic left temporal lobe (small thin arrows) in a child with intractable temporal lobe epilepsy and bilateral epileptiform discharges on EEG. The child underwent epilepsy surgery with simultaneous resection of the left medial frontal cortex and hippocampus and is seizure-free
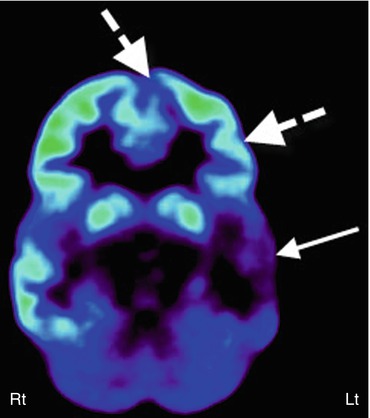
Fig. 50.3
2-Deoxy-2(18F)-fluoro-d-glucose PET scan in a case of suspected secondary epileptic focus. A child with long-standing temporal lobe epilepsy (primary seizure focus) developed a change in seizure semiology with independent epileptiform discharges from frontal regions. PET scan showed severe hypometabolism of the left temporal lobe (arrow) along with reduced glucose metabolism in the left inferior frontal lobe (broken arrows), suggesting a possible secondary epileptic focus because of persistent kindling through the uncinate fasciculus
Another PET tracer, FMZ, has been investigated by quite a few centers. FMZ binds to the GABAA receptors, the main binding sites of the inhibitory neurotransmitter γ-aminobutyric acid (GABA). Since neurons in the normal hippocampus and amygdala have strong GABAA receptor expression, FMZ PET has been found to be particularly useful in detecting medial temporal lobe epileptic foci, which often show decreased FMZ binding, and may sometimes delineate abnormalities, not seen or subtle on FDG PET (Fig. 50.4). FMZ PET can also show decreased GABAA receptor binding in the epileptogenic neocortex, and the extent of abnormality is usually less than that seen on FDG PET scan (Henry et al. 1993; Savic et al. 1993; Szelies et al. 1996; Ryvlin et al. 1998). FMZ PET is almost 100 % sensitive in cases of hippocampal sclerosis and can reveal contralateral abnormality in almost one third of patients with apparently unilateral hippocampal sclerosis on MRI (Henry et al. 1993; Koepp et al. 1996). FMZ PET can sometimes show increased FMZ binding in cases of cortical developmental malformations (Koepp et al. 1997) or microdysgenesis in the white matter (Hammers et al. 2002). Still, clinical use of FMZ PET in temporal lobe epilepsy is not established. Increasing availability of novel, 18F-labeled (thus, longer half-life) flumazenil tracers may facilitate further exploration of this technique as a clinical tool.
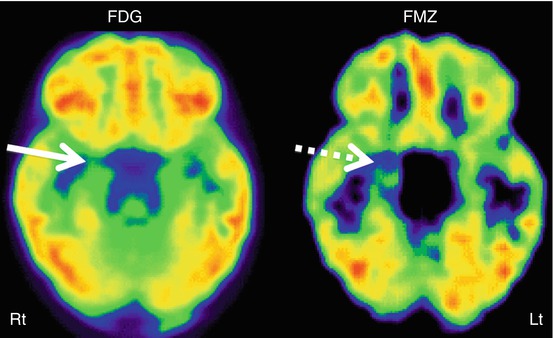
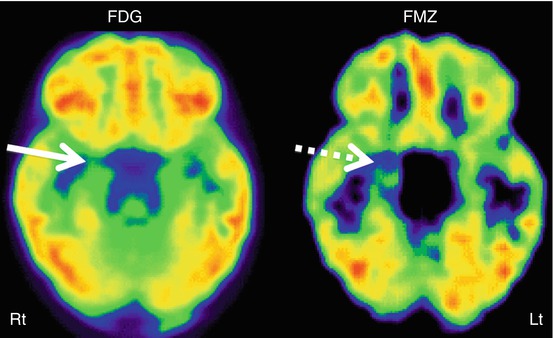
Fig. 50.4
11C-Flumazenil PET (right image) showing better delineation of right hippocampal abnormality (broken arrow) in a child with medial temporal lobe epilepsy, compared to 2-deoxy-2(18F)-fluoro-d-glucose PET (left image) which showed only subtle changes (solid arrow) in this region
Several other PET tracers have been tested in temporal lobe epilepsy for receptor imaging with variable results. AMT PET has been reported to be somewhat useful in identifying the epileptic foci in patients with temporal lobe epilepsy and normal hippocampal volume, but not in those with hippocampal sclerosis (Natsume et al. 2003); however, the added clinical value in individual cases remains uncertain. Studies of opiate receptor PET imaging have reported both increased (Frost et al. 1988; Madar et al. 1997) and normal (Mayberg et al. 1991; Theodore et al. 1992) receptor density in patients with temporal lobe epilepsy. Similarly, the level of monoamine oxidase B has been found to be both high (Kumlien et al. 1992) and low (Kumlien et al. 2001) in temporal lobe epilepsy. While significantly reduced dopamine receptor binding has been reported in the epileptogenic temporal lobe (Werhahn et al. 2006), no binding alterations were found with 11C-verapamil PET, a P-glycoprotein substrate (Langer et al. 2007). Patients with temporal lobe epilepsy have also been shown to have reduced NMDA receptor binding (Kumlien et al. 1999), but increased 11C-doxepine uptake (Iinuma et al. 1993) in the ipsilateral temporal cortex. Recently, evidence of inflammatory changes was found in the hippocampus, parahippocampal gyrus, amygdala, and fusiform gyrus, ipsilateral to the epileptic focus in patients with temporal lobe epilepsy who underwent PET scanning with the translocator protein (TSPO) tracer 11C-PBR28 (Hirvonen et al. 2012).
Although limited SPECT data exist in the pediatric epilepsy population, it has been reported to be consistent with other noninvasive presurgical examinations in up to 95 % of patients (O’Brien et al. 1998; Chiron et al. 1999a; Vera et al. 1999; Lawson et al. 2000; Kaminska et al. 2003; Lee et al. 2005; Kurian et al. 2007). Interictal SPECT has very low sensitivity (less than 50 %) in the detection of epileptogenic regions in temporal lobe epilepsy, with false-positive or false-negative findings in 20–75 % cases. In contrast, ictal SPECT can correctly localize the epileptogenic foci in 70–90 % of cases with unilateral temporal lobe epilepsy (Fig. 50.5) (Rowe et al. 1991; Harvey et al. 1993; Lee et al. 2005; Benifla et al. 2006). Various registration techniques, such as SPM or subtraction ictal SPECT co-registered to MRI (SISCOM), can further increase the sensitivity and specificity of ictal SPECT but requires two separate SPECT studies including interictal and ictal scans. In children, SISCOM has been reported to be helpful in identifying the epileptogenic region in up to 95 % of cases (Chiron et al. 1999b). In comparison to intracranial EEG findings, ictal ECD SPECT was found to correctly localize the seizure onset zone in 80 % of children with intractable epilepsy (Kaminska et al. 2003). In addition, in the majority of children with favorable outcome following resective epilepsy surgery, the surgical margin coincided with the SPECT focus. Postictal SPECT is also more sensitive (70–90 %) than interictal SPECT, and also its accuracy can improve further with the use of SISCOM (Rowe et al. 1989; O’Brien et al. 1999). SISCOM can be particularly useful in cases of dysembryoplastic neuroepithelial tumor (DNET), which is often located in the temporal lobe and most prevalent in the pediatric population. SISCOM can demonstrate some additional dysplastic cortical areas around the DNET, and removal of these areas is essential for better surgical outcome. The use of SISCOM can increase the focus detection rate up to 93 %, compared to 74 % without it (Chiron et al. 1999b; Vera et al. 1999; Valenti et al. 2002).
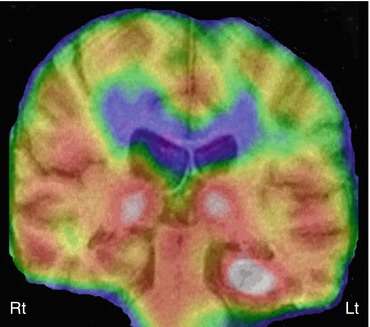
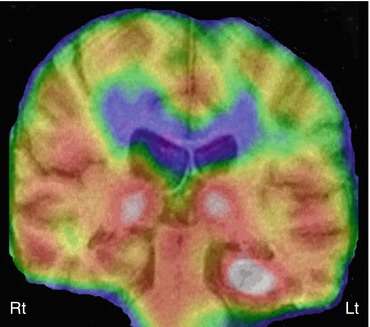
Fig. 50.5
Ictal 99mTc-ethyl cysteinate dimer SPECT (coronal view, co-registered on to the corresponding MRI slice) in a child with temporal lobe epilepsy showing increased perfusion in the left hippocampus, suggesting an epileptic focus. Also noted is increased perfusion in bilateral thalami, which can be seen during the ictal phase
Extratemporal Lobe Epilepsy
FDG PET plays a relatively more important role in extratemporal lobe epilepsy in children, compared to adults, due to its higher incidence in children with a large majority having noncontributory MRI (Wyllie et al. 1998). Normal MRI is perhaps due to the higher incidence of subtle cortical dysplasia in children compared to the adults, as these lesions are often difficult to detect on MRI. Here FDG PET can play a valuable role, as the dysplastic cortex is usually hypometabolic (Fig. 50.6). Even when MRI is positive, it does not provide the full extent of the dysplasia, which is more obvious on FDG PET scan. FDG PET can also sometimes reveal additional epileptic foci, which can have a huge impact on surgical planning. Although a variable sensitivity of 45–92 % has been reported for FDG PET in extratemporal lobe epilepsy, usually it is less sensitive than in temporal lobe epilepsy (Henry et al. 1991; Swartz et al. 1995; da Silva et al. 1997; Kim et al. 2002; Honbolygo et al. 2006; Patil et al. 2007). The wide range of sensitivity is likely due to the variable patient populations studied and the lack of standardized analytic techniques across studies.
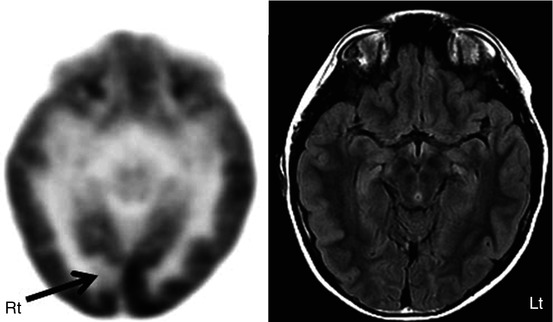
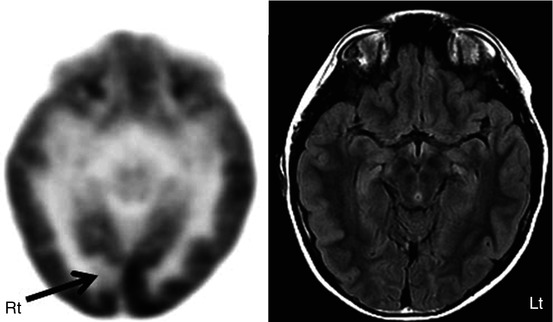
Fig. 50.6
2-Deoxy-2(18F)-fluoro-d-glucose PET (left image) in a child with intractable epilepsy, normal FLAIR MRI (right image), and bilateral synchrony on EEG showed a very focal area of hypometabolism (arrow), which is strongly suspected to be cortical dysplasia
FMZ PET has a sensitivity of 60–100 % for detecting the epileptogenic cortex in extratemporal epilepsy (Savic et al. 1995; Ryvlin et al. 1998; Muzik et al. 2000b), although most studies included a highly biased surgical population. FMZ PET abnormalities tend to be smaller than the area of FDG hypometabolism and sometimes may show better concordance with intracranial EEG findings (Arnold et al. 2000; Juhasz et al. 2000; Szelies et al. 2002) (Fig. 50.7). FMZ PET can reveal abnormality even in patients with normal MRI (Muzik et al. 2000a; Koepp and Woermann 2005), and the complete surgical resection of FMZ abnormality has been found to be associated with good seizure outcome (Muzik et al. 2000b; Juhasz et al. 2001). The use of SPM can further increase the usefulness of FMZ PET, providing objective detection of cortical GABAA receptor abnormalities in those with or without normal MRI (Richardson et al. 1998, 2001).
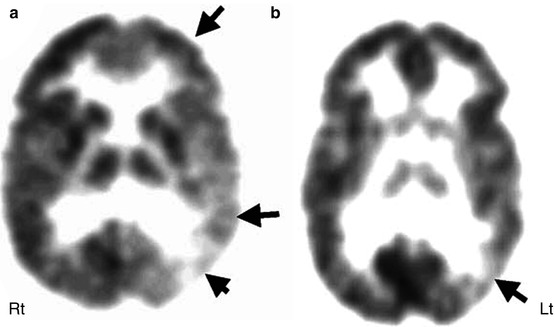
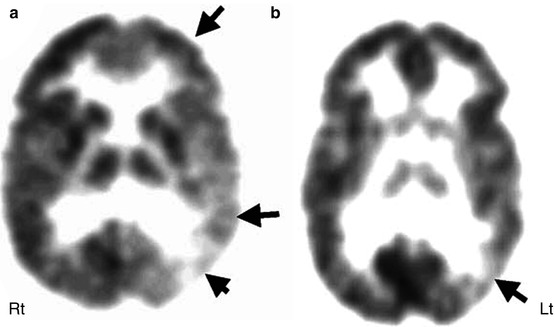
Fig. 50.7
11C-flumazenil PET (b) showing much smaller area of reduced flumazenil binding (arrow) compared to the extensive area of reduced glucose uptake in 2-deoxy-2(18F)-fluoro-d-glucose PET scan (a) in the left temporal and frontal lobe, which was more consistent with predominantly posterior quadrant epileptiform activity on EEG
Another PET tracer, AMT, a labeled tryptophan tracer, appears to have some role in selected cases, particularly in patients with cortical dysplasia and normal MRI and mild FDG changes (Juhasz et al. 2003; Wakamoto et al. 2008; Kumar et al. 2011). In these cases, AMT PET sometimes shows increased tracer uptake, particularly in patients with cortical dysplasia type IIB, who usually have very good surgical outcome (Chugani et al. 2011) (Fig. 50.8). This is an important development as it demonstrates, for the first time, that an in vivo imaging technique appears to be able to predict pathologic subtype and thus may provide presurgical prognostic information. Although the specificity of AMT PET is very high for detecting the seizure onset lobe in both lesional (97 %) and non-lesional neocortical epilepsies (up to 100 %), its sensitivity is much lower (47 % in lesional vs. 29 % in non-lesional cases) (Wakamoto et al. 2008).
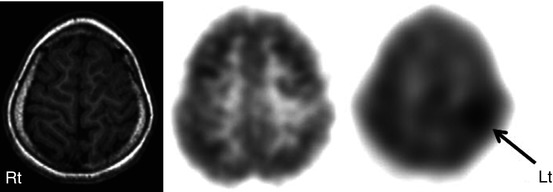
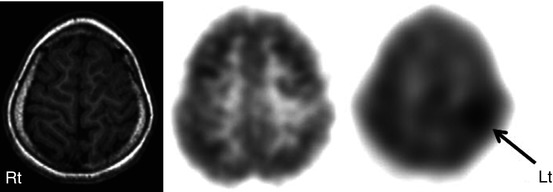
Fig. 50.8
T1-weighted MRI (left image), 2-deoxy-2(18F)-fluoro-d-glucose (middle image), and 11C-alpha-methyl tryptophan PET scan (right image) in a child with intractable epilepsy and a left frontal epileptic focus on the EEG. Whereas MRI was normal and 2-deoxy-2(18F)-fluoro-d-glucose PET showed only mild hypometabolism in the left frontal region, 11C-alpha-methyl tryptophan PET scan showed a focal area of increased tracer uptake interictally in this region (arrow). Postsurgical histopathology revealed focal cortical dysplasia
There have been a few studies which have evaluated various other brain receptors in extratemporal epilepsy. Lower opiate receptor availability was reported in the left parieto-temporo-occipital cortex in patients with reading epilepsy (Koepp et al. 1998). While reduced dopamine receptor binding in the right putamen was reported in autosomal dominant nocturnal frontal lobe epilepsy (Fedi et al. 2008), another group of investigators found increased tracer binding to nicotinic acetylcholine receptors in the midbrain, pons, and cerebellum and reduced binding in the dorsolateral prefrontal cortex in this condition (Picard et al. 2006).
Obtaining useful SPECT in pediatric extratemporal epilepsy is often difficult, as seizures are usually of short duration and propagate rapidly (such as infantile spasms or myoclonic epilepsy), thus making it difficult to acquire an ictal SPECT study. Postictal scans also appear less helpful because, unlike in temporal lobe epilepsy, seizure-induced perfusion changes do not always extend into the postictal phase. However, a success rate of 70 % has been reported for ictal HMPAO SPECT in localizing epileptic foci (Hwang et al. 2001), and under certain conditions, it can be very useful, particularly in children with non-localizing EEG (Marks et al. 1992). Again, the use of SISCOM can further increase its localizing value (O’Brien et al. 1998; Vera et al. 1999) (Fig. 50.9). In two studies, ictal SPECT and SISCOM were found to be more sensitive than FDG PET in the detection of epileptic foci (Sturm et al. 2000; Seo et al. 2011). However, in another study of occipital lobe epilepsy, ictal HMPAO SPECT was found to be less sensitive than FDG PET (Kim et al. 2001). The use of SISCOM appears to increase the diagnostic yield in extratemporal lobe epilepsy also and correlates better with postsurgical outcome (O’Brien et al. 2000; Knowlton et al. 2008). Nevertheless, a multimodal imaging approach may provide the most benefit in children with non-lesional epilepsy (Seo et al. 2011).
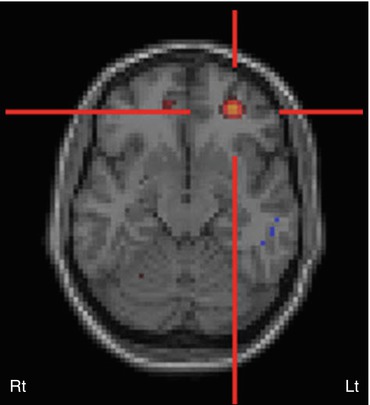
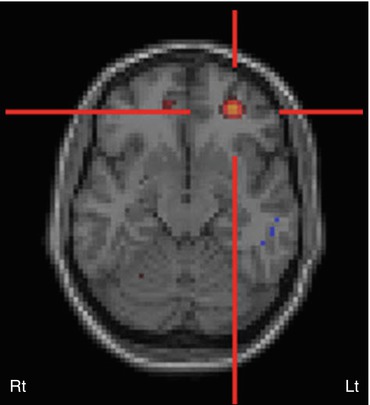
Fig. 50.9
Subtraction ictal SPECT co-registered to MRI (SISCOM) image in a patient with intractable epilepsy, normal MRI, and non-localizing surface EEG. SISCOM revealed a focal area of hyperperfusion in the left inferior frontal cortex (red cross)
50.2.2 Pediatric Epilepsy Syndromes
Infantile Spasms or West Syndrome
PET can play an important role in the evaluation of patients with infantile spasms, particularly those diagnosed with cryptogenic infantile spasms (Chugani et al. 1990, 1993). For example, uni- or multifocal cortical metabolic abnormalities were found in 95 % of children with an initial diagnosis of cryptogenic infantile spasms (Chugani and Conti 1996). Four different patterns of FDG hypometabolism were observed: unifocal in 20 %, multifocal in 65 %, diffuse in 5 %, and bitemporal hypometabolism in 10 % of children (Chugani and Conti 1996). Out of these, the patients with unifocal lesion can be considered for epilepsy surgery if they have uncontrolled spasms with the majority of EEG changes corresponding to the PET-defined lesion, as the surgical removal of focal brain lesion leads not only to cessation of the spasms but also to improved neurocognitive outcome (Fig. 50.10) (Branch and Dyken 1979; Mimaki et al. 1983; Palm et al. 1988; Ruggieri et al. 1989; Uthman et al. 1991; Chugani et al. 1993; Asarnow et al. 1997; Jonas et al. 2005; Yum et al. 2011). Occasionally, patients with a multifocal pattern can also be considered for palliative surgery, with improved quality of life but guarded developmental outcome, if the majority of seizures originate from one region (Chugani et al. 2010). The diffuse metabolic pattern is likely associated with underlying metabolic, genetic, or neurodegenerative pathology, and these patients are unlikely to be surgical candidates. Interestingly, children with bitemporal hypometabolism show a distinct clinical phenotype characterized by severe developmental delay, particularly in the language domain, and autism or pervasive developmental disorder (Chugani et al. 1996). These children are unlikely to become surgical candidates.
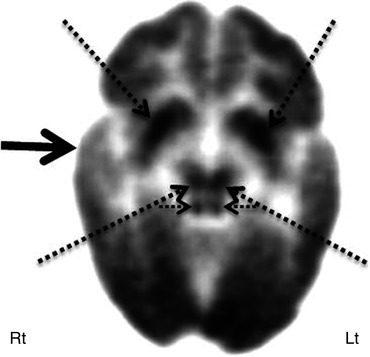
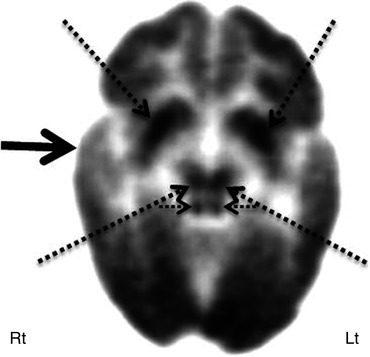
Fig. 50.10
2-Deoxy-2(18F)-fluoro-d-glucose PET showed focal hypometabolism in the right temporal cortex (solid arrow) in a child with infantile spasms and normal MRI. This child may be considered for focal cortical resection for possible seizure control and cognitive improvement. Also noted is hypermetabolism in the bilateral basal ganglia and brain stem nuclei (dotted arrows), likely implicating their role in spasm generation
FDG PET can also shed some light on the possible pathomechanism of epileptic spasms. For example, these patients may show a focal cortical hypometabolism along with prominent glucose metabolism in the lenticular nuclei and brain stem, suggesting complex cortical-subcortical interactions (Fig. 50.10) (Chugani et al. 1992). Basal ganglia and brain stem involvement likely suggests their role in the secondary generalization of focal cortical discharges, resulting in spasms. It appears that the spasms result primarily from focal or diffuse cortical abnormalities interacting with the brain stem and lenticular nuclei and that this type of generalization accounts for the bilateral motor involvement and relative symmetry of the majority of spasms even in the presence of a discrete focal lesion (Chugani et al. 1992). Ictal and interictal brain SPECT can also provide some interesting clues regarding the probable origin and propagation of electrical events responsible for spasms. Interictal SPECT studies have shown perfusion abnormalities, ranging from cortical hypoperfusion (Chiron et al. 1993; Miyazaki et al. 1994; Hwang et al. 1996; Sztriha et al. 1997; Haginoya et al. 1998, 2001) to cortical hyperperfusion (Chiron et al. 1993). Ictal SPECT has shown areas of cortical hyperperfusion with occasional hyperperfusion of the subcortical structures (Haginoya et al. 1998, 2001), consistent with FDG PET findings. Recent studies, using a combination of interictal and ictal SPECT, found ictal hyperperfusion of a cortical lesion and basal ganglia, supporting the notion that secondary generalization from a cortical focus may account for the spasms (Mori et al. 2007; Kakisaka et al. 2009). The finding of localized cortical perfusion defects in some children with West syndrome confirms that focal cortical lesions play an important role in its development (Haginoya et al. 2000). Interestingly, in infants, hyperperfusion has been found interictally in the area of cortical dysgenesis and appears to be specific for this young population (Hwang et al. 1996; Haginoya et al. 2000). These findings corroborate the notion that infantile spasms result from complex cortical-subcortical interaction with age and brain maturity playing an important role. Rapid involvement of the basal ganglia and brain stem likely results in the secondary generalization of a seemingly partial seizure, arising from a focal cortical lesion (Chugani et al. 1992). What leads to this interaction is yet to be elucidated.
Tuberous Sclerosis
Tuberous sclerosis complex (TSC) is an autosomal dominant neurocutaneous disorder characterized by multiple cortical tubers, caused by inactivating mutations in the TSC genes. Epilepsy is the main manifestation of this condition, with a prevalence of 80–90 % (Osborne et al. 1991), becoming refractory to medical treatment in 50–80 % cases, as early as 2 years of age (Evans et al. 2012). As intractable seizures have a detrimental effect on neurocognitive development, these patients often benefit from epilepsy surgery (Perot et al. 1966). However, many times it is difficult to localize the epileptogenic focus, due to multifocal EEG changes and multiplicity of cortical tubers, even though a single tuber may be epileptogenic. In these cases, PET can play a valuable role by noninvasively lateralizing and localizing the epileptic focus interictally and rendering these patients surgical candidates. FDG PET can show the tubers (Asano et al. 2000, 2005; Ohta et al. 2001; Karenfort et al. 2002; Chandra et al. 2006; Weiner et al. 2006; Moshel et al. 2010; Wu et al. 2010; Carlson et al. 2011); however, it cannot identify the epileptogenic one, as all tubers appear hypometabolic (Fig. 50.11). AMT PET has been reported to be very useful in this condition, as it shows increased tracer uptake in epileptogenic tubers only (Fig. 50.11) (Chugani et al. 1998a; Asano et al. 2000; Fedi et al. 2003), sometimes at one of its edges only with some involvement of the adjacent apparently normal cortex; such cortical regions usually turn out to be dysplastic on histopathological examination (Kagawa et al. 2005). The increased AMT uptake appears to be due to the activation of the kynurenine pathway, leading to the production of quinolinic acid, a proconvulsant, in the tuber and dysplastic cortex (Chugani et al. 1998a). AMT PET with quantitative evaluation of lesional uptake levels can identify epileptogenic tuber(s) in almost two thirds of children with tuberous sclerosis and intractable epilepsy and is almost 100 % specific (Chugani et al. 1998a; Asano et al. 2000; Fedi et al. 2003). Tubers with at least 10 % increase of AMT uptake appear to be invariably epileptogenic, and a cutoff threshold of 1.02 for AMT uptake ratio provides 83 % accuracy for detecting tubers that need to be resected to achieve a seizure-free outcome (Asano et al. 2000; Kagawa et al. 2005). The high specificity of increased AMT uptake is also suggested by the good correlation between resections of epileptogenic tubers, showing increased AMT uptake and seizure outcome (Kagawa et al. 2005). However, its sensitivity is suboptimal, likely depending upon the underlying pathology and method of data analysis. It appears that MRI-based quantitative assessment can increase the sensitivity of AMT PET to 79 % from 44 % with visual assessment (Juhász et al. 2002). This may be related to the fact that non-epileptic tubers show decreased AMT uptake and the epileptogenic ones with relatively increased AMT uptake, but still close to the surrounding normal cortex, and, therefore, these increases might appear subtle and be missed on visual analysis. SPECT can also play an important role in identifying epileptic tubers, by showing them hyperperfused during ictal phase (Koh et al. 1999; Romanelli et al. 2002; Weiner et al. 2006; Moshel et al. 2010; Aboian et al. 2011; Carlson et al. 2011). A good correlation has been reported between ictal SPECT and ictal scalp EEG (Koh et al. 1999; Mori et al. 2007). SISCOM further increases the usefulness of SPECT in identifying the epileptogenic zone and in guiding the location and extent of epilepsy surgery in children with tuberous sclerosis complex and multifocal abnormalities (Aboian et al. 2011). Complete resection of the SISCOM hyperperfusion abnormality was found to be associated with seizure freedom. SPECT, using 123I iomazenil, has been used to evaluate benzodiazepine receptor status in patients with tuberous sclerosis (Kuki et al. 2008, 2012; Mori et al. 2012). GABA receptor binding was found to be decreased in cortical tubers (Mori et al. 2012), suggesting that decreased neuronal inhibition might have a role in epileptogenesis in this condition.
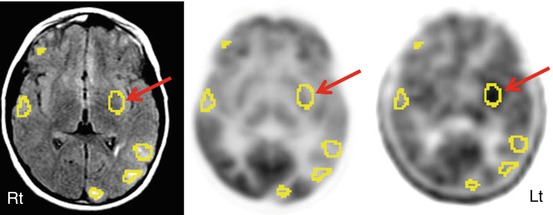
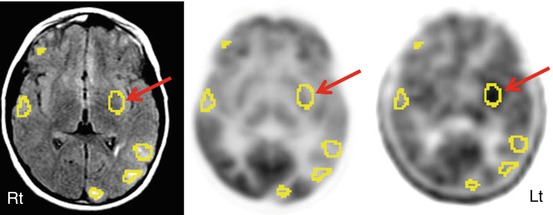
Fig. 50.11
FLAIR MRI (left image), 2-deoxy-2(18F)-fluoro-d-glucose (middle image), and 11C-alpha-methyl tryptophan PET (right image) scan in a patient with multiple tubers (encircled with “region of interests”), intractable seizures, and non-localizing scalp EEG. While 2-deoxy-2(18F)-fluoro-d-glucose PET showed hypometabolism in all the tubers and overlying cortices, interictal 11C-alpha-methyl tryptophan PET scan revealed increased alpha-methyl tryptophan uptake in an insular tuber (arrow)
Lennox-Gastaut Syndrome
FDG PET can help in the metabolic characterization of the brain in Lennox-Gastaut syndrome, a triad of multiple seizure types including tonic seizures, developmental delay, and 1–2.5 Hz generalized “slow” spike-and-wave EEG pattern, which may have implication for prognosis, as well as treatment planning (Gur et al. 1982; Chugani et al. 1987b; Iinuma et al. 1987; Theodore et al. 1987; Miyauchi et al. 1988; Ferrie et al. 1996; You et al. 2007; Zhai et al. 2010; Hur et al. 2011). These patients may show four metabolic patterns on FDG PET scan: unilateral focal, unilateral diffuse, bilateral diffuse hypometabolism, and normal patterns (Chugani et al. 1987b). Interictal SPECT usually shows multiple areas of hypoperfusion (Heiskala et al. 1993). Patients with unilateral focal pattern may be occasionally considered for cortical resection provided there is good concordance between PET and ictal EEG findings (You et al. 2007; Hur et al. 2011).
Sturge-Weber Syndrome
In children with Sturge-Weber syndrome (SWS), FDG PET reveals hypometabolism in the involved cortex and usually identifies additional areas of abnormal cortex extending beyond the abnormality seen on MRI (Chugani et al. 1989; Juhasz et al. 2007). However, affected infants may show a paradoxical pattern of increased glucose metabolism interictally in the cortex underlying the leptomeningeal angioma; as the disease progresses, the hypermetabolic area becomes hypometabolic (Fig. 50.12) (Chugani et al. 1989). The presence of early cortical hypermetabolism on PET may be an imaging marker of subsequent severe epilepsy, requiring surgery (Alkonyi et al. 2011). In some young patients, serial FDG PET scans show rapidly progressing and severe hypometabolism in the affected area, probably because of rapid demise of the brain tissue associated with the angioma: these patients will have improvement in seizure status and paradoxical preservation of cognitive function and therefore may not require surgical intervention (Behen et al. 2011). Early and rapid progression in unilateral cases of SWS leads to early and more efficient reorganization in the contralateral cortex. On the other hand, persistent mild hypometabolism of the lesion may indicate ongoing functional disturbance, and these patients may show persistent seizures and developmental arrest (Lee et al. 2001b; Behen et al. 2011). These are the patients who require surgical intervention for seizure control and possible cognitive improvement by promoting effective reorganization in the contralateral hemisphere while brain plasticity is still at a maximum during development. In SWS, detrimental metabolic changes mostly occur before 3–4 years of age (Juhasz et al. 2007), coinciding with a sharp increase in developmentally regulated cerebral metabolic demand (Chugani et al. 1987a). Progressive hypometabolism is associated with high seizure frequency in these children. However, metabolic abnormalities may remain limited or even partially recover later in some children with well-controlled seizures. Metabolic recovery accompanied by neurological improvement suggests a critical window for therapeutic intervention in children with unilateral SWS (Juhasz et al. 2007).
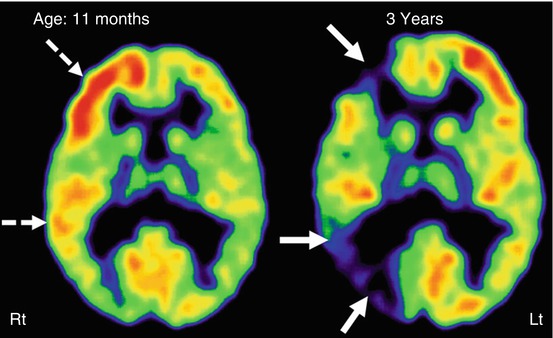
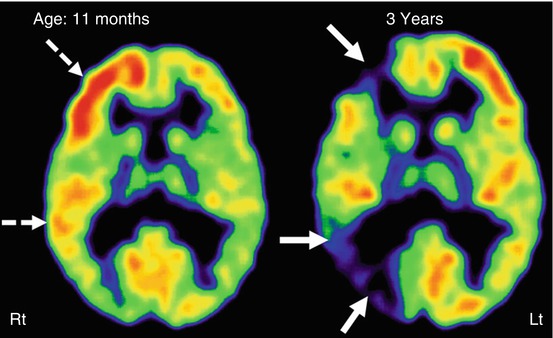
Fig. 50.12
Serial 2-deoxy-2(18F)-fluoro-d-glucose PET scan showing progression of glucose metabolic abnormalities in the affected right hemisphere (MRI showed extensive leptomeningeal enhancement on that side) in a child with Sturge-Weber syndrome. PET scan at 11 months of age showed a transient increase of metabolism, most prominent in the frontal lobe and mild in the temporal cortex (dashed arrows). Repeat PET at 3 years of age showed severe hypometabolism in the right frontal and temporal cortices (solid arrows)
Perfusion SPECT, using xenon-133, showed hyperperfusion in the lesion even before seizure onset (Pinton et al. 1997), analogous to the transient hypermetabolism seen on PET (Chugani et al. 1989; Juhasz et al. 2007). After 1 year of age, these areas typically show hypoperfusion, as revealed by another xenon-133 SPECT study (Chiron et al. 1989). In a single case report on a patient with failed functional hemispherectomy, ictal ECD SPECT showed hyperperfusion in the residual lesion with falsely lateralized EEG; further surgery resulted in seizure freedom (Bilgin et al. 2008). In recent years, SPECT studies have been largely replaced by perfusion MRI, which can be performed in conjunction with conventional clinical MRI and provide quantitative maps of blood volume and blood flow in children with Sturge-Weber syndrome (Alkonyi et al. 2012).
Hemimegalencephaly
Hemimegalencephaly is a severe congenital malformation of hemispheric development with a unilateral enlarged and defectively developed hemisphere and intractable seizures. PET and SPECT usually show variable appearance in the involved hemisphere: hypo- and/or hypermetabolism on the FDG PET and hypo- and/or hyperperfusion on HMPAO or ECD SPECT, depending upon the seizure status at the time of scanning. Early hemisphere disconnection in these children may lead to seizure control and improved cognitive development, provided the other hemisphere is normal. Therefore, the main role of PET or SPECT is the evaluation of the apparently normal hemisphere (Fig. 50.13). In children with hemimegalencephaly, FDG PET often shows additional less pronounced abnormalities in the opposite hemisphere, which probably accounts for the suboptimal cognitive outcome even with complete seizure control after surgical removal of the profoundly abnormal hemisphere (Rintahaka et al. 1993). Thus, FDG PET can be useful in such cases to assess the functional integrity of the contralateral hemisphere prior to hemispherectomy and help predict cognitive outcome.
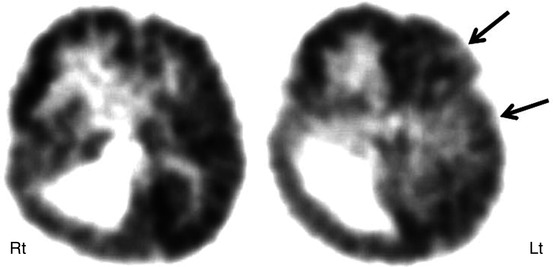
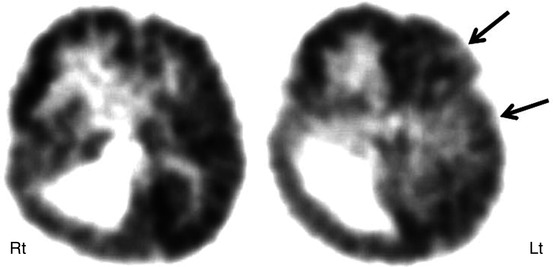
Fig. 50.13
2-Deoxy-2(18F)-fluoro-d-glucose PET scan in a child with right hemimegalencephaly showing an enlarged right hemisphere with increased glucose metabolism in the right frontal lobe because of epileptic activity during the radiotracer uptake period. Also noted are mildly hypometabolic areas in the frontal and temporal regions of the left hemisphere (arrows), indicating functional impairment of this hemisphere, which appeared to be normal on MRI. Although right hemispherectomy resulted in seizure freedom, the child remained developmentally delayed because of an abnormal left hemisphere
Rasmussen’s Encephalitis and Epilepsy of Suspected Inflammatory Origin
FDG PET can show both hyper- or hypometabolism in cases of Rasmussen’s encephalitis, depending upon the seizure status during the scan (Tien et al. 1992; Fiorella et al. 2001; Lee et al. 2001a). It can particularly help in the unequivocal identification of the affected cerebral hemisphere in patients whose MR imaging findings are subtle or distributed bilaterally (Fiorella et al. 2001). FDG PET can further help in guiding the site of biopsy when indicated, thus assisting in earlier diagnosis. Interictal hypometabolism expands over time and usually precedes the anatomical changes, thus further helping in clarifying the equivocal cases or assessing the functional impairment and its evolution (Lee et al. 2001a). Perfusion SPECT also shows hyper- or hypometabolism of the affected cortex, depending upon the seizure status during the tracer injection, and can guide the histopathological evaluation or surgical planning (Yacubian et al. 1997; Burneo et al. 2006).
Neuroinflammation is the underlying cause for intractable epilepsy in Rasmussen’s encephalitis, as well as some other epileptic conditions. Neuroinflammation is mediated by activated microglia, which secrete a number of proinflammatory molecules such as cytokines, chemokines and neurotoxins, free radicals, nitric oxide, proteinases, eicosanoids, and excitotoxins, which may play a role in epileptogenesis. Although the exact mechanisms are unclear, it appears that the inflammatory mediators act by increasing glutamatergic neurotransmission, decreasing GABA-mediated currents and inducing neovascularization, and damaging the blood–brain barrier. Detection of microglia is not possible with current radiological methods or biochemical techniques; however, they can be imaged with PET tracers binding to the activated microglia. 11C-PK11195 is the most commonly used radioligand which binds specifically to the translocator protein receptors, predominantly expressed by the activated microglia in cases of neuroinflammation, thus making the in vivo detection of neuroinflammation possible (Banati et al. 1999). PET scanning using 11C-PK-11195 can help in the early diagnosis of Rasmussen’s syndrome or other inflammatory conditions with intractable seizures, where CT and MRI are often normal for several months after the clinical manifestation of the disease (Fig. 50.14). Localization of the most affected brain regions may also provide a guide in deciding the site of brain biopsy in order to avoid sampling errors and can help in the surgical removal of that region (Kumar et al. 2008).
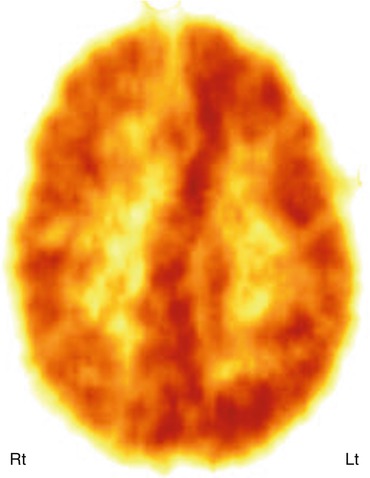
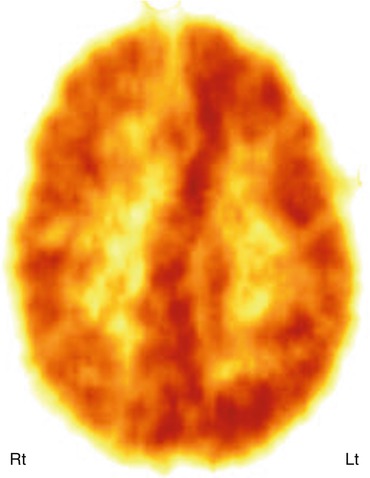
Fig. 50.14
11C-PK11195 PET scan showing increased radiotracer binding in the left hemisphere, indicating neuroinflammation mediated by activated microglia, in a child with Rasmussen’s encephalitis
50.3 Other Neurological Disorders
50.3.1 Perinatal Hypoxic Ischemic Brain Injury and Cerebral Palsy
PET scanning of cerebral glucose metabolism can be helpful to assess severity of brain damage in infants with hypoxic ischemic encephalopathy (HIE) and brain injury. Early FDG PET studies found areas of hypometabolism, extending beyond the affected regions shown by CT scan (Doyle et al. 1983). Cerebral perfusion PET showed relative hypoperfusion in the parasagittal regions in full-term neonates with perinatal asphyxia (Volpe et al. 1985). In preterm infants with intraventricular and intracerebral hemorrhage, large areas of hypoperfusion were reported in the affected hemisphere (Volpe et al. 1983). Subsequent studies suggested that persistently low cerebral glucose metabolism is associated with delayed development in children with HIE (Suhonen-Polvi et al. 1993). Total brain glucose metabolic rates (CMRglc) were found to be inversely correlated with the severity of HIE: neonates with the lowest CMRglc subsequently developed permanent neurological symptoms and cerebral palsy (Thorngren-Jerneck et al. 2001). A transient increase of glucose metabolic rates has been also observed in the basal ganglia in the neonatal period, followed by severe hypometabolism in the lenticular nuclei and thalami, in some newborns who suffered HIE and subsequently developed dystonic cerebral palsy (Fig. 50.15) (Batista et al. 2007).
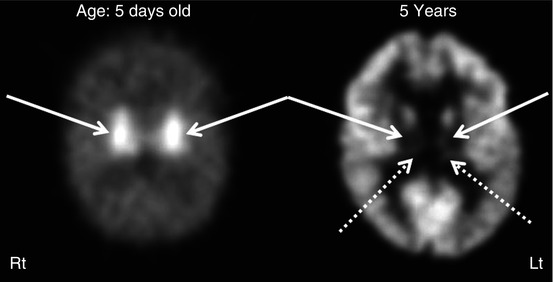
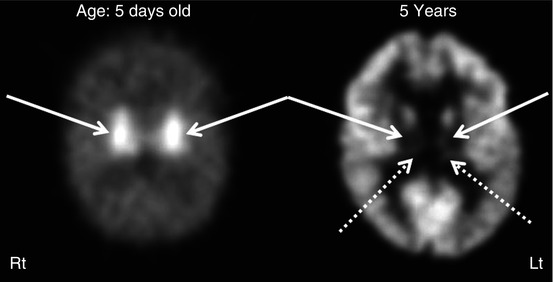
Fig. 50.15
2-Deoxy-2(18F)-fluoro-d-glucose PET scans of a child who suffered hypoxic ischemic encephalopathy at birth and later developed dystonic/choreoathetoid cerebral palsy. The left image from the newborn period showed intense hypermetabolism in the basal ganglia (solid arrows). The right image at 5 years of age showed severe hypometabolism in the lenticular nuclei (solid arrows) and thalami (dotted arrows). Note the relative preservation of metabolism in the cerebral cortex
Cerebral palsy is a heterogeneous neurological condition that sometimes may be related to perinatal brain injury. FDG PET studies of these children with cerebral palsy due to birth injury have shown that the distribution of metabolic impairment almost invariably extends beyond the apparent anatomical involvement seen on MRI. PEt also can help in identifying the location and severity of functional involvement of cortical and subcortical structures in the major subtypes of cerebral palsy in the chronic stages. Analysis of these different subtypes demonstrated focal areas of cortical hypometabolism in spastic diplegic patients, without any obvious cortical lesion on structural imaging; in contrast, a relatively normal pattern of cortical metabolism is seen in some children with choreoathetoid cerebral palsy, who often show hypometabolism in the thalamus and lenticular nuclei (Kerrigan et al. 1991). Relative sparing of the cerebral cortex is consistent with the clinical observation that many of these children have good cognitive function despite suffering from severe motor impairment. Use of FMZ PET has demonstrated increased GABA receptor binding in the bilateral motor and visual cortex and decreased binding in the brain stem, suggesting a role of abnormal GABA mechanisms in poor motor control (Lee et al. 2007). In addition, recent studies with a rabbit model of intrauterine brain injury suggested that PET imaging of activated microglia in newborns, using [11C]PK11195, can detect neuroinflammatory changes in the white matter that later develops severe structural damage causing symptoms of cerebral palsy (Kannan et al. 2007).
50.3.2 Autism
Functional neuroimaging in autism is currently a clinical research tool and has been utilized to identify neuroanatomic substrates involved in various symptoms associated with the disease. In early studies with PET scanning, increased glucose metabolic rates were reported in multiple brain regions in autistic adults, but no common regional abnormality could be identified (Rumsey et al. 1985). In a subsequent study involving 18 autistic children, neither the rates nor the regional distribution of brain glucose metabolism was different from those in control subjects (De Volder et al. 1987). Similarly, no significant abnormality in regional cerebral blood flow, oxygen consumption, and metabolism was reported in six adult autistic subjects (Herold et al. 1988). However, another study found some reversed (left>right) asymmetry of glucose metabolism in the anterior rectal gyrus (Siegel et al. 1992). A combined MRI and PET study in high-functioning autistic patients reported volume loss and associated hypometabolism in the anterior and posterior cingulate (Haznedar et al. 1997, 2000). Perfusion PET studies using radiolabeled water found hypoperfusion in the right dentate nucleus and left frontal cortex during verbal auditory and expressive language tasks (Muller et al. 1998) and hypoperfusion of the superior temporal cortex during acoustic stimulation and the cerebellum during nonverbal auditory perception task (Muller et al. 1999). Subsequently, lower glucose metabolism was reported in the medial frontal cortex and cingulate (Hazlett et al. 2004) and in the ventral caudate, putamen, and thalamus (Haznedar et al. 2006) during verbal memory task in autistic subjects.
Simultaneously with glucose PET studies, several SPECT studies attempted to find global or focal cerebral perfusion abnormalities in autistic subjects in the 1990s; most early studies were small and the results were mixed. One study found no regional perfusion abnormalities in 21 autistic children on xenon-133 SPECT (Zilbovicius et al. 1992). Another smaller HMPAO SPECT study found global hypoperfusion as well as regions with hypoperfusion, including the right lateral temporal lobe and frontal lobes in four autistic adults (George et al. 1992). In a subsequent longitudinal study, the same group reported frontal hypoperfusion in autistic children at ages 3–4 years, which disappeared by the ages of 6–7 years, suggesting a delayed frontal maturation in childhood autism. Yet another HMPAO SPECT study showed decreased blood flow in the temporoparietal region in six autistic patients between 9 and 21 years of age, without any apparent clinical correlation (Mountz et al. 1995). A relatively larger ECD SPECT study, including 23 children with infantile autism compared to 26 non-autistic controls (matched for age and IQ), reported decreased regional blood flow in the bilateral insula, superior temporal gyri, and left prefrontal cortices and found several clinico-imaging correlations (Ohnishi et al. 2000). First, altered perfusion in the medial prefrontal cortex and anterior cingulate gyrus was associated with impairments in communication and social interaction (related to deficits in the theory of mind), and second, altered perfusion in the right medial temporal lobe was related to the obsessive desire for sameness. Thus, these findings pointed to specific locations of brain dysfunction underlying abnormal behavioral patterns in autistic patients. More recent studies have utilized objective analytic approaches such as SPM to identify abnormal cerebral blood flow patterns in autism. One such study, using ECD SPECT, also reported both global reductions and an increased right-left perfusion asymmetry in 11 autistic children, as compared to age-matched healthy children; the asymmetries were particularly prominent in the temporoparietal areas associated with language and comprehension of music and sound (Burroni et al. 2008). Another study, using a similar approach in 23 children with autistic spectrum disorder (mostly boys), found bilateral frontal, temporal, and basal ganglia hypoperfusion in autistic children (Yang et al. 2011). Interestingly, there were greater asymmetries in children with Asperger syndrome than autism, suggesting different neurobiological mechanisms.
PET imaging with various radiotracers allows the study of global and regional cerebral abnormalities of specific neurotransmitter systems and their potential roles in the pathophysiology of autism. These studies have been designed based on previous work demonstrating alterations in neurotransmitters, hormones, and their metabolites in the blood, cerebrospinal fluid (CSF), and urine of autistic patients. In a PET study using [18F]-labeled fluorodopa (F-DOPA, a precursor of dopamine, accumulated in dopaminergic terminals) in 14 medication-free autistic children, decreased dopaminergic activity was found only in the anterior medial prefrontal cortex in the autistic group, suggesting that decreased dopaminergic function in the prefrontal cortex may contribute to the cognitive impairment seen in autism (Ernst et al. 1997). A subsequent study of 20 adults with autism also demonstrated increased dopamine transporter binding in the orbitofrontal cortex, and these abnormalities were inversely correlated with serotonin transporter binding, suggesting a potential interplay between the dopaminergic and serotonergic systems in affected brain regions (Nakamura et al. 2010).
Abnormalities of the serotonergic system have been long implicated in the pathophysiology of autism. Tryptophan depletion, leading to decreased serotonin levels, resulted in an exacerbation of symptoms in autistic subjects (McDougle et al. 1996b). Conversely, serotonin reuptake inhibitors may result in the improvement of compulsive symptoms, repetitive movements, and social difficulties in autistic adults (Cook et al. 1992; Gordon et al. 1993; McDougle et al. 1996a). Chugani et al. (1997) used AMT PET scanning to study children with autism and their healthy non-autistic siblings. AMT PET demonstrated robust AMT uptake asymmetries in the frontal cortex, thalamus, and cerebellum in seven autistic boys (Fig. 50.16); however, this pattern was not seen in one autistic girl and in four of the five siblings. Specifically, decreased AMT accumulation was seen in the left frontal cortex and thalamus in five of seven autistic boys, while elevated AMT accumulation was present in the right cerebellum, in the dentate nucleus. The same children showed a normal pattern of brain glucose metabolism and normal MRI on visual evaluation, supporting that the observed novel focal cerebral abnormalities on AMT PET were specific for serotonergic abnormalities. In a subsequent larger study, Chugani et al. (1999) also reported global brain values for serotonin synthesis capacity of 54 children: 30 healthy seizure-free autistic children (24 males, 2–15 years), 8 healthy non-autistic siblings (6 males, 2–14 years), and 16 epileptic children without autism (9 males, 3 months–13 years). Non-autistic children showed a nonlinear change in global serotonin synthesis capacity: their values reached >200 % of adult values until the age of about 5 years and then gradually declined toward adult values. In autistic children, a different developmental pattern was found: serotonin synthesis capacity increased gradually between the ages of 2 and 15 years to values 1.5 times of the adult normal values. The data indicated a period of relatively high brain serotonin synthesis capacity during childhood and that the normal developmental process is disrupted in autistic children. These results prompted further larger-scale imaging studies of the serotonergic system of the developing brain in autism. When data of a larger group (n = 117) of autistic children were analyzed, additional focal patterns were recognized, including right cortical and left cortical decreases and a subgroup with no abnormal asymmetry (Chandana et al. 2005). Children with left cortical AMT uptake decreases showed a higher prevalence of severe language impairment, whereas right cortical decreases were associated with left and mixed handedness. These studies, altogether, suggested that both global and focal asymmetric development in the serotonergic system may be implicated in abnormal “wiring” of neural circuits related to hemispheric specialization. Therefore, restoration of serotonin levels pharmacologically in a critical age window may alter developmental trajectory and improve symptoms of autistic children.
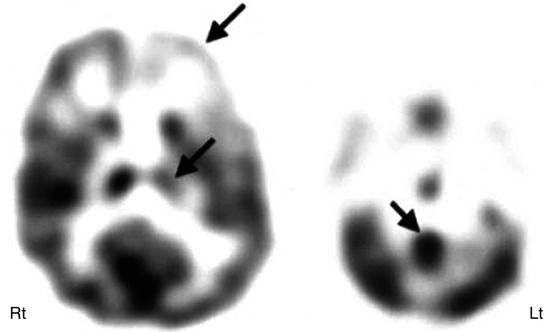
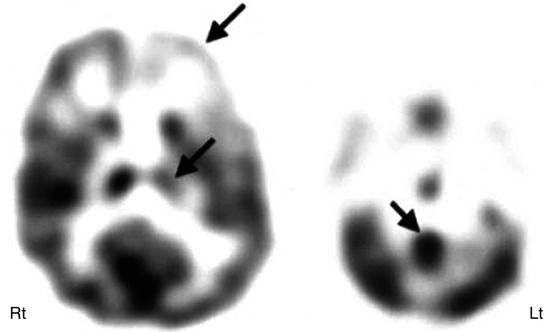
Fig. 50.16
11C-Alpha-methyl tryptophan PET showing asymmetric alpha-methyl tryptophan uptake in the frontal cortex, thalamus, and dentate nucleus of the cerebellum in a boy with autism spectrum disorder, with decreased uptake in the ipsilateral frontal cortex and thalamus with increased uptake in the contralateral dentate nucleus (arrows), suggesting the involvement of dentato-thalamo-cortical pathway in autism
50.3.3 Developmental Dyslexia
Dyslexia is a specific learning disability that manifests primarily with difficulty in written language, particularly with reading and spelling. Various PET studies have shown the predominant involvement of bilateral posterior temporal and parietal cortices. Initial cerebral blood flow studies, using Xe-133, found increased left more than right asymmetry and reduced anterior to posterior difference (Rumsey et al. 1987), as well as hypoperfusion in the left superior temporal region with hyperperfusion in the more posterior temporoparietal region (Flowers et al. 1991). Subsequently, PET studies using 15O-labeled water in dyslexic subjects showed a failure to activate the left temporoparietal cortex during a rhyme detection task and reduced activation/unusual deactivation in the mid- and posterior temporal cortex bilaterally and also in inferior parietal cortex, predominantly on the left side (Rumsey et al. 1992, 1997). Other PET studies showed less activation in the right superior temporal and right postcentral gyri and left cerebellum (McCrory et al. 2000) or in the right cerebellar cortex and left cingulate gyrus (Nicolson et al. 1999). Functional disconnection of the left angular gyrus from other parts of the reading network has also been reported in adults with persistent developmental dyslexia (Horwitz et al. 1998). A recent 15O PET study of the learning of phoneme categorization found a less strongly left-lateralized activation involving the superior temporal, inferior parietal, and inferior lateral frontal cortex during the speech mode (Dufor et al. 2007). Frontal and parietal subparts of these left-sided regions were found to be significantly less activated in the dyslexic subjects. However, larger activations in the right frontal cortex for both speech and acoustic modes compared to rest and an unexpected large deactivation in the medial occipital cortex for the acoustic mode were noted in the dyslexic subjects. Subsequently, the same group found activity enhancement in left premotor cortex, likely related to the persistence of motor coding for allophonic representations of speech (Dufor et al. 2009).
FDG PET studies have shown diminished asymmetry in glucose metabolism in prefrontal and lingual (inferior) regions of the occipital lobe during reading in dyslexic subjects, indicating that brain regions are activated differently in this disorder (Gross-Glenn et al. 1991). Another FDG PET study showed significantly higher absolute and relative brain metabolism along an anterior-posterior gradient, including the medial temporal lobe, in dyslexic subjects, during an auditory syllable discrimination task, suggesting more effortful processing in dyslexia (Hagman et al. 1992). Perfusion SPECT has also shown hypoperfusion in areas involved in the reading and writing processes, predominantly in the temporal lobe (Kaneko et al. 1998; Arduini et al. 2006; Sauer et al. 2006).
50.3.4 Landau-Kleffner Syndrome
Landau-Kleffner syndrome (LKS) is characterized by the sudden or gradual development of aphasia in children who had previously developed typically with normal language until disease onset. While many of the affected children have clinical seizures, some may have only electrographic seizures. FDG PET usually shows bilateral temporal lobe involvement, in the form of hyper- or hypometabolism depending upon the electrographic status of this region, particularly of the superior and medial part (Maquet et al. 1990; Rintahaka et al. 1995; da Silva et al. 1997; Honbolygo et al. 2006; Shiraishi et al. 2007). During the phase of aphasia, frequent epileptiform spiking is seen in these regions, associated with glucose hypermetabolism on FDG PET, which becomes quiescent during remission, manifested as hypometabolism on FDG PET scan. FDG PET can also show the possible involvement of temporal lobes in the generation of continuous spike waves during slow-wave sleep (Maquet et al. 1990, 1995; Rintahaka et al. 1995). FDG PET can be further useful to monitor dynamic changes of glucose metabolism in the temporal lobe during episodes of aphasia and remission, where it shows hyper- and hypometabolism, likely related to presence or absence of continuous spiking on scalp EEG, respectively, during these periods (Fig. 50.17) (Luat et al. 2006). Using 15O-labeled water PET, posterior superior temporal gyri were found to have decreased perfusion/activation, long after remission was achieved in patients with LKS, and residual impairment in verbal short-term memory, suggesting an important role played by the temporal lobe in this condition (Majerus et al. 2003). FMZ PET revealed a deficit in benzodiazepine receptor binding at the tip of the left temporal lobe in a child affected by LKS, suggesting that the tip of the left temporal lobe might play an important role in the pathogenesis of this disease (Shiraishi et al. 2007). SPECT studies have also shown variable perfusion abnormalities, predominantly in uni- or bilateral temporal lobes, and ranging from hypo- to hyperperfusion, likely related to the timing of the SPECT (Hu et al. 1989; O’Tuama et al. 1992; Guerreiro et al. 1996; Harbord et al. 1999; Sayit et al. 1999; Sinclair and Snyder 2005; Shiraishi et al. 2007).
