An underlying hypothesis for rapid cartilage loss in patients with osteoarthritis (OA) is that perturbation from normal joint mechanics produces locally high biomechanical strains that exceed the material properties of the tissue, leading to rapid destruction. Several imaging findings are associated with focally high biomechanical forces and thus are potential candidates for predictive biomarkers of rapid OA progression. This article focuses on 3 aspects of knee biomechanics that have potential magnetic resonance imaging correlates, and which may serve as prognostic biomarkers: knee malalignment, meniscal dysfunction, and injury of the osteochondral unit.
The American College of Rheumatology defines osteoarthritis (OA) as a heterogeneous group of conditions that leads to joint signs and symptoms that are associated with defective integrity of articular cartilage, and associated changes in the underlying bone and at the joint margins. Although OA is a multifactorial disease process, early cartilage damage and ultimate loss of articular cartilage is a central feature and a significant contributor to clinical symptoms. The rate of progression of tissue damage and clinical symptoms can vary substantially between patients. A clinical challenge in managing patients with OA is differentiating individuals at risk for rapidly progressive disease. A potential role of imaging is identification of specific biomarkers that are prognostic of rapid OA progression. In clinical care such indicators could guide lifestyle changes or treatment recommendations in select patients at greatest risk for rapid onset of OA. In research, identifying subjects likely to have rapid OA progression would provide more efficient clinical trials by shortening the observation period or allowing for a smaller sample size.
It is difficult to identify specific features predictive of OA progression using radiographic methods. A systematic review of 1004 studies conducted prior to 2003 identified 37 studies meeting the inclusion criteria for quality and suggests that knee pain, radiologic severity at baseline, sex, quadriceps strength, knee injury, and regular sport activities are not related to OA progression. For other factors, the evidence was limited or conflicting. The more recent use of magnetic resonance (MR) imaging in clinical studies of OA has the potential to provide additional imaging biomarkers that may be better predictors of OA progression. In a cohort of 43 subjects, Biswal and colleagues found that anterior cruciate and meniscal tears along with focal chondral lesions in the central weight-bearing zones were predictive of more rapid OA progression. A recent report from the Multi-center Osteoarthritis Trial (MOST trial) identified high body mass index (BMI), meniscal damage, meniscal extrusion, and any high-grade MR imaging feature defined as a Whole-Organ Magnetic Resonance Imaging Score (WORMS) score of 2 or more as baseline risk factors for fast cartilage loss over a 30-month period. However; because these features were present in both slow and rapid progressors, they did not predict the rate of OA progression. Further study is needed to determine whether combination of imaging biomarkers or greater refinement of potential biomarkers will improve differentiation of those individuals who are likely to have rapidly progressive OA.
An underlying hypothesis for rapid cartilage loss in patients with OA is that perturbation from normal joint mechanics produces locally high biomechanical strains that exceed the material properties of the tissue, leading to rapid destruction. Several imaging findings are associated with focally high biomechanical forces and thus are potential candidates for predictive biomarkers of rapid OA progression. In this article, the authors focus on 3 aspects of knee biomechanics that have potential MR imaging correlates, and which may serve as prognostic biomarkers: knee malalignment, meniscal dysfunction, and injury of the osteochondral unit.
Knee malalignment
The causes of varus and valgus malalignment are multifactorial, and can lead to an imbalance in loading of knee articular cartilage. Local factors within the joint, such as loss of joint congruence through bone and cartilage injury, anterior cruciate ligament disruption, and meniscal degeneration and extrusion, play a role in determining alignment. Other causes of acquired varus and valgus malalignment include osseous remodeling, osteophytes, and ligament and capsular damage resulting from chronic repetitive microtrauma and tissue remodeling. Prior surgical procedures including osteotomy, meniscectomy, and meniscal debridement may also affect knee alignment. Childhood malalignment has been proposed to have a high association with OA. In a natural history study by Schouten and colleagues, patients with childhood varus or valgus malalignment had a fivefold increase in risk of OA. Deviation from neutral alignment at the hip, knee, or ankle will also affect load distribution at the knee.
As illustrated in Fig. 1 , static assessment of knee alignment can be made using the mechanical axis determined from full-length standing views of the lower extremities. The mechanical axis of the lower extremity is represented on radiographs by a line drawn from the center of the femoral head to the center of the talus. Mechanical axis deviation is measured by a perpendicular line drawn from the center of the knee to the mechanical axis on the anteroposterior radiograph. In a neutrally aligned limb, the mechanical axis passes just medial to the midpoint of the knee between the tibial spines. In a varus knee, the mechanical axis deviates medially, increasing the load on the medial compartment. When the mechanical load-bearing axis passes lateral to the tibial spines, a valgus knee increases stress on the lateral compartment.
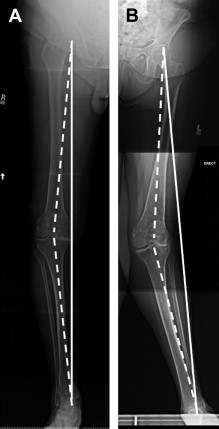
Knee alignment in the frontal plane is based on the relative angle between the mechanical axis of the femur and tibia. The mechanical axis of the femur passes from mid femoral head to center of the intracondylar notch. The mechanical axis of the tibia extends from the tibial spines to the mid talus. Measurement of knee alignment with the mechanical axis has been criticized for pelvic radiation exposure, higher cost, and the need for specialized equipment. A study by Kraus and colleagues demonstrated a high correlation between the data obtained from full-limb measures of the mechanical axis and short-film measurements using the anatomic axis of the distal femur and proximal tibia. In the tibia, the mechanical and anatomic axes are the same, but they differ in the femur where the anatomic axis is defined by the line that bisects the distal femoral diaphysis. In this study, the anatomic axis measurement was offset a mean 4.2° valgus from the mechanical axis measurement (3.5° in women and 6.4° in men).
The average knee alignment in those who do not have OA has been shown to be approximately 1° to 2° varus. In neutral alignment, the medial compartment bears 60% to 70% of the force across the knee during weight bearing. It has been proposed that the greater degree of loading may predispose to medial tibiofemoral compartment OA, which is 3 times more common than lateral compartment OA.
A recent systematic review found limited evidence for knee malalignment and incident knee OA; however, there was strong evidence that malalignment is an independent risk factor for progression of radiographic knee OA. With regard to incident OA, there are preliminary data to indicate that obese and overweight individuals with knee malalignment are at increased risk of developing knee OA compared with those with a normal BMI.
In the presence of existing OA, malalignment is associated with more rapid structural damage in the compartment with increased compressive force. Varus and valgus alignment increases focal joint loading and risk of OA progression in the medial and lateral compartments, respectively. The severity of malalignment at baseline showed a correlation with the magnitude of joint space narrowing and was linked to greater decline in physical function. Varus malalignment has been shown to lead to a fourfold increase in medial knee OA progression. Valgus malalignment has been shown to predispose to a twofold to fivefold increase in lateral OA progression. In an MR imaging study using quantitative assessment of cartilage in 251 knees, varus malalignment predicted medial tibial cartilage volume loss and tibial and femoral bone erosion, even after adjusting for other local factors such as meniscal damage, extrusion, and laxity.
While there was an effect of malalignment at almost all stages of knee OA examined, the impact of varus or valgus malalignment on the probability of disease progression increased with baseline severity of OA. In knees with mild OA (Kellgren and Lawrence [K/L] grade 2: definite osteophytes and definite narrowing of joint space), the probability of 18-month progression in medial compartment OA was increased fourfold by varus alignment at baseline, and lateral progression increased twofold by valgus alignment (approaching statistical significance). In knees with moderate OA (K/L grade 3: moderate multiple osteophytes, definite narrowing of joint space, some sclerosis, and possible deformity of bone contour), the impact of malalignment in either the varus or valgus direction on OA progression over the subsequent 18 months demonstrated a tenfold increase. The difference in impact between varus and valgus alignment on OA progression was less apparent in K/L grade 3 knees.
Assessment of malalignment from standing radiographs provides only a static impression of the mechanical forces being imparted on the joint in the frontal plane. Dynamic assessment of limb biomechanics is complex, and is achieved through gait analysis and measurement of knee adduction moment, which requires dynamic measurement of the ground reaction force and lower extremity alignment. The knee adduction moment arm, which adducts the knee during the stance phase of normal gait, has been described as the best predictor of knee OA progression. The mean maximum magnitude of the adduction moment during normal gait is approximately 3.3% body weight multiplied by height. Data from Miyazaki and colleagues found a significant relationship between adduction moment and the mechanical axis.
Techniques for estimating knee alignment have been proposed using coronal MR images from the hip through the ankle in an unloaded state. Although these techniques underestimate the degree of valgus malalignment, they have been shown to provide an accurate assessment of varus malalignment. Chronic high loading conditions can perturb the subchondral marrow signal and thus serve as an indirect marker of abnormal joint mechanics. As illustrated in Fig. 2 , subchondral marrow changes with decreased T1-weighted signal intensity and increased T2-weighted signal intensity are frequently associated with increased biomechanical load. Areas of abnormal MR imaging signal have been shown to also have increased radionuclide uptake on bone scan, suggesting they are associated with bone remodeling. Although frequently referred to as “bone marrow edema,” when evaluated histologically these sites of abnormal signal intensity are associated with a combination of bone marrow necrosis, fibrosis, and trabecular abnormalities. The amount of true bone marrow edema was similar in marrow with abnormal signal and normal perifocal or control zones. Although there is still variation in terminology, this finding is generally referred to as a bone marrow edema-like lesion (BML).
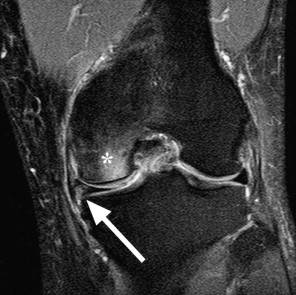
In patients with radiographic knee OA, those with subchondral BMLs are more likely to have knee pain than those without them. Large BMLs are associated with both progression of cartilage defects in the tibiofemoral joint and more rapid lateral tibial cartilage loss. The site of the BML is predictive of compartmental cartilage loss. Risk for medial progression was increased more than sixfold in patients with medial BMLs, and patients with lateral BMLs were at a similar risk for lateral progression. Much of the relationship of BMLs with radiographic progression of OA is explained by their association with malalignment. Varus limbs have an extraordinarily high prevalence of medial BMLs, whereas lateral BMLs occurred preferentially in valgus aligned limbs. OA progression in patients with BMLs may be the consequence of the marrow lesions themselves, knee malalignment, or some combination of the two. As discussed further in this article, BMLs are also associated with abnormal loading of subchondral bone resulting from meniscal injury and damage of the overlying articular cartilage.
Meniscal dysfunction
Since the pioneering work of Fairbanks in the 1940s, the meniscus has been recognized as a critical component for normal knee function and biomechanics. The meniscus plays a major role in load bearing and distribution, shock absorption, and knee proprioception, and it is also reported to play a role in knee stability and joint lubrication. Menisci are wedge shaped in cross section, with capillaries and nerves that penetrate into the peripheral 10% to 30% of the outer edge of meniscus. The lateral meniscus is more mobile in contradistinction to the medial meniscus, which is firmly attached to the joint capsule. Under normal conditions the lateral meniscus is estimated to carry 70% of the load and the medial meniscus 50% of the load on their respective sides of the knee. The load carried by the meniscus increases with knee flexion.
The structural orientation of collagen in the meniscus contributes to the biomechanical properties of the tissue. Meniscal collagen is approximately 98% type I, which is tightly woven in a predominantly circumferential pattern. Both menisci are attached to the tibia via anterior and posterior root ligaments, which anchor the circumferentially oriented hoop fibers. The hoop fibers are stabilized with radially oriented tie fibers. When the tibiofemoral joint is compressed, a portion of the compressive load is dissipated by converting the compressive force into tensile strain in the hoop fibers. The hoop strength of the collagen fiber orientation resists meniscal extrusion or subluxation. A damaged meniscus with disruption of the hoop fibers will be dislodged peripherally as axial load is applied, resulting in extrusion and joint space narrowing on radiograph. Tears of the posterior insertional ligament illustrated in Fig. 3 lead to loss of hoop strain, and from the standpoint of joint contact pressure are functionally similar to a complete meniscectomy. Radial tears that transect the hoop fibers substantially decrease the contact area of the tibiofemoral joint, increase the compressive load on the articular cartilage, and synergistically act to concentrate high loads on a small region of the articular surface. The absence of a functioning meniscus is reported to increase peak and average contact stresses in the medial compartment over a range of 40% to 700%. As illustrated by the case presented in Fig. 4 , locally high loading pressure can exceed the material properties of the tissue, resulting in rapid cartilage loss or osteochondral fracture.
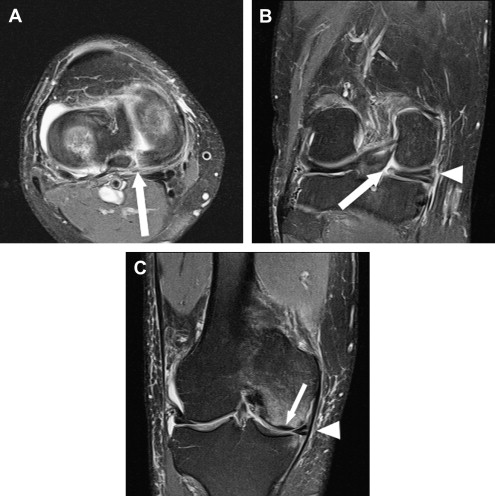
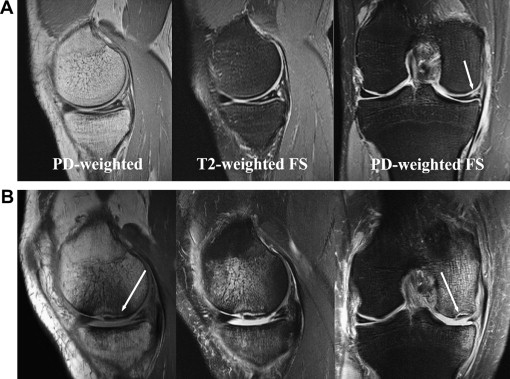
Normal menisci are rarely present in knees with OA. Degenerative meniscal tears or maceration of the meniscus is often present suggesting a strong relation between OA and meniscal pathology. In a study of middle-aged and elderly patients in Framingham, Massachusetts, 82% of knees with radiographic OA had meniscal damage and the prevalence increased with a higher K/L grade. In many cases, it is difficult to determine whether meniscal damage or OA was first to develop. The meniscus and the articular surface are interdependent, and failure of one will lead to more rapid damage of the other by increased load.
Meniscal damage plays a significant role in progression of knee OA. Biswal and colleagues demonstrated a higher rate of progression of cartilage loss in 26 of 43 patients with meniscal tears compared with a control group with intact menisci. In a study by Hunter and colleagues, the most significant risk for cartilage loss was related to the WORMS meniscal damage variable. However, the WORMS grading system does not specify the type of meniscal tear or the degree of meniscal extrusion. Another potential weakness of MR imaging for meniscal evaluation is that the lack of axial load on the knee may underestimate meniscal extrusion in the knee with OA. It remains uncertain as to what level of meniscal dysfunction related to loss of hoop strain must be lost before the risk of OA is increased.
In addition to removing displaced meniscal fragments that may cause symptoms of knee pain and locking, a goal of surgery in treatment of meniscal tears is to preserve meniscal function. Several methods of meniscal repair have been used over the years. The first reported operation was a meniscal suturing by a British surgeon in 1883. The same surgeon later recommended complete removal of the meniscus as preferable to repair. Total meniscectomy predominated for several years until it was shown to significantly increase the rate of OA progression. In 1948, Fairbank compared preoperative and postoperative radiographs in 107 patients undergoing meniscectomy and demonstrated development of ridge formation on the femoral condyle, joint space narrowing, and flattening of the femoral condyle in the postoperative patients. Fairbank hypothesized that frequent radiographic changes found after total meniscectomy were caused by loss of the load-protective function of the menisci. Further studies confirmed an increased incidence of OA after total meniscectomy. The most compelling argument against total meniscectomy was a 1998 study that reported a significant increase in risk for tibiofemoral OA over a 21-year period following total meniscectomy when compared with a matched control group. Total meniscectomy of the lateral compartment has been shown to have a worse outcome than medial meniscal resection, possibly a result of the greater portion of load carried by the lateral meniscus than by the medial meniscus.
Arthroscopic partial meniscectomy has several advantages over total meniscectomy, and is presently the treatment of choice. This procedure involves a limited resection of only the damaged portion of the meniscus. Short-term benefits include decreased length of stay in the hospital and shorter rehabilitation time. Long-term benefits include continued meniscal participation in load distribution, knee stability, and shock absorption if sufficient circumferentially oriented fibers are intact and hoop strength is sufficient to prevent extrusion. Meniscal repair may be indicated for acute lesions in young patients if the lesion is in the outer one-third “red zone” of the meniscus with a vascular supply to allow healing. Meniscal transplants have been described, but are preformed infrequently.
Meniscal dysfunction
Since the pioneering work of Fairbanks in the 1940s, the meniscus has been recognized as a critical component for normal knee function and biomechanics. The meniscus plays a major role in load bearing and distribution, shock absorption, and knee proprioception, and it is also reported to play a role in knee stability and joint lubrication. Menisci are wedge shaped in cross section, with capillaries and nerves that penetrate into the peripheral 10% to 30% of the outer edge of meniscus. The lateral meniscus is more mobile in contradistinction to the medial meniscus, which is firmly attached to the joint capsule. Under normal conditions the lateral meniscus is estimated to carry 70% of the load and the medial meniscus 50% of the load on their respective sides of the knee. The load carried by the meniscus increases with knee flexion.
The structural orientation of collagen in the meniscus contributes to the biomechanical properties of the tissue. Meniscal collagen is approximately 98% type I, which is tightly woven in a predominantly circumferential pattern. Both menisci are attached to the tibia via anterior and posterior root ligaments, which anchor the circumferentially oriented hoop fibers. The hoop fibers are stabilized with radially oriented tie fibers. When the tibiofemoral joint is compressed, a portion of the compressive load is dissipated by converting the compressive force into tensile strain in the hoop fibers. The hoop strength of the collagen fiber orientation resists meniscal extrusion or subluxation. A damaged meniscus with disruption of the hoop fibers will be dislodged peripherally as axial load is applied, resulting in extrusion and joint space narrowing on radiograph. Tears of the posterior insertional ligament illustrated in Fig. 3 lead to loss of hoop strain, and from the standpoint of joint contact pressure are functionally similar to a complete meniscectomy. Radial tears that transect the hoop fibers substantially decrease the contact area of the tibiofemoral joint, increase the compressive load on the articular cartilage, and synergistically act to concentrate high loads on a small region of the articular surface. The absence of a functioning meniscus is reported to increase peak and average contact stresses in the medial compartment over a range of 40% to 700%. As illustrated by the case presented in Fig. 4 , locally high loading pressure can exceed the material properties of the tissue, resulting in rapid cartilage loss or osteochondral fracture.
