When planning for brain tumor resection, a balance between maximizing resection and minimizing injury to eloquent brain parenchyma is paramount. The advent of blood oxygenation level–dependent functional magnetic resonance (fMR) imaging has allowed researchers and clinicians to reliably measure physiologic fluctuations in brain oxygenation related to neuronal activity with good spatial resolution. fMR imaging can offer a unique insight into preoperative planning for brain tumors by identifying eloquent areas of the brain affected or spared by the neoplasm. This article discusses the fMR imaging techniques and their applications in neurosurgical planning.
Key points
- •
Functional neuroimaging has been shown to be a valuable tool in preoperative mapping of brain functions in patients with tumors and provides reliable in vivo assessment of the eloquent cortex to minimize the risk of postsurgical morbidity.
- •
Task-based functional magnetic resonance (tb-fMR) imaging is the most commonly used method for noninvasive assessment of eloquent cortex and can reliably display cortical activity, including sensorimotor, language, and visual functions.
- •
Emerging evidence suggests that resting state functional MR imaging is a promising tool in addition to tb-fMR imaging in clinical settings, and it has the potential to become the noninvasive standard tool for surgical planning and a biomarker of prognosis in patients with brain tumor.
- •
Although functional MR imaging is a powerful tool that can significantly influence the planning for brain tumor surgery, there are some limitations to this technique that should be addressed in order to optimally perform and interpret the results in clinical practice.
Introduction
When approached with the task of brain tumor resection, finding a balance between maximizing resection and minimizing injury to eloquent brain parenchyma is paramount. Technological advances have enabled the functional mapping of regions of the brain, which can be used for many clinical applications and investigative opportunities. Specifically, the advent of blood oxygenation level–dependent (BOLD) functional magnetic resonance (fMR) imaging has allowed researchers and clinicians to measure physiologic fluctuations in brain oxygenation related to neuronal activity with good spatial resolution. When coupled with intraoperative awake functional mapping, an avoidance of unanticipated resection-related deficits can substantially reduce the risk of morbidity of neurosurgical procedures. This article highlights the benefits that task-based fMR (tb-fMR) imaging has provided to surgical therapeutic practice.
Background of functional magnetic resonance imaging (blood oxygenation level–dependent signal, functional magnetic resonance imaging study design)
fMR imaging is typically performed using the BOLD contrast technique, described by Ogawa and colleagues in 1990. Deoxygenated hemoglobin, being paramagnetic, results in increased local susceptibility changes. In contrast, oxygenated hemoglobin is diamagnetic. Gradient echo techniques sensitive to these susceptibility changes can be used to determine relative oxyhemoglobin versus deoxyhemoglobin concentrations in blood. The variation of BOLD signal changes as a function of time in response to neural activity is called the hemodynamic response function. The principle of neurovascular coupling states that, for a given set of neurons that are active, there are changes in local field potential that induce regional neurovascular changes, which result in an overall influx of local oxygenated blood, thus increasing relative signal on BOLD magnetic resonance (MR) imaging. Therefore, during acquisition of BOLD MR imaging, a patient that is actively engaging in a particular task shows increased relative signal in brain areas subserving the task.
Using this principle, investigators designed specific functional tasks presented at regular or random intervals to study patients under alternating blocks of exposure or under the condition of sporadic measured events. Simple task-based experimental design allows the control of behavior in a manner that may be easily reproduced and from which clinicians can assign cortical functional regions. Through task structure previously measured in healthy persons, the same experimental design can be applied to patients that may have a lesion in the region responsible for a particular task.
Functional magnetic resonance imaging: current clinical applications
Clinical use of fMR imaging is a recent phenomenon with about 20 years of collective experience. The main clinical application is in the presurgical evaluation and mapping of patients with structural lesions such as brain tumors, and in patients with epilepsy. Preoperative fMR imaging studies have been shown to be helpful to surgeons for mapping of eloquent cortex and proximity to the target lesion, allowing informed decisions to be made regarding potential risks or safety of surgical resection and the surgical trajectory, and may influence the need for intraoperative cortical mapping. When fMR imaging is used for presurgical planning, the choice of task paradigms to be used depends mainly on the location of the lesion. For example, if the tumor is located in the posterior frontal lobe, motor paradigms can be used to map the motor strip.
Presurgical mapping has also been extensively used in patients with epilepsy, primarily for hemispheric language lateralization. In patients with epilepsy, a higher percentage of patients show atypical language lateralization than in normal individuals. Sometimes there is discordance between expressive and receptive language lateralization. It has also been used for motor and visual cortical mapping in cases where malformations of cortical development or other resectable epileptogenic lesions are thought to be in close spatial proximity to such eloquent cortical regions.
This evolution of fMR imaging in clinical practice has been facilitated by the development of a CPT (Current Procedural Terminology) code for this technique by the American Society of Functional Neuroradiology (ASFNR) as well as several other studies on this topic. Notwithstanding, it is not the scope of this article to discuss the clinical applications of presurgical fMR imaging mapping in patients with epilepsy, cortical developmental lesions, as well as cortical plasticity in patients with vascular malformations (such as arteriovenous malformations and cavernomas), which are amply reviewed by the authors and others elsewhere.
Functional magnetic resonance imaging applications in brain tumor surgery
The clinical application of fMR imaging has been well established in preoperative brain tumor surgery planning and it is currently part of routine preoperative work-up in patients with brain tumors that are close to areas of presumed selected eloquent brain function. , In this context, it is essential to maximize the lesion resection while preserving the adjacent eloquent cortex to minimize the postoperative neurologic and functional deficits. , Beyond significant anatomic variations of the individual human brains, lesions may result in mass effect, with anatomic distortion limiting assessment of normal anatomic landmarks; furthermore, slow-growing lesions may result in brain plasticity and recruitment of brain regions that are not typically associated with a specific task or function. As a result, the eloquent cortex areas adjacent to the tumor may not be localized accurately merely by structural brain imaging. Clinical fMR imaging can identify the critical brain regions that are associated with patients’ daily functions, such as motor, language, and visual functions. Preoperative identification of eloquent cortices could help neurosurgeons to select the best surgical approach, to plan the extent of the resection, and to optimize intraoperative direct cortical stimulation (DCS). Notably, an important question for surgeons is to determine whether the lesion is ipsilateral or contralateral to the dominant language areas. Intraoperative DCS remains the gold standard in localizing the dominant language areas; however, this procedure is time consuming and has considerable procedural challenges and limitations. Taking these into consideration, using preoperative fMR imaging to determine the lesion and language laterality is a useful noninvasive alternative tool that is complementary to intraoperative DCS.
Preoperative fMR imaging has several other advantages in addition to determining language laterality. The anatomic relationship of the lesion to adjacent eloquent areas can be examined using fMR imaging; the data can be processed to optimize visualization of essential cortical structures, including three-dimensional displays to guide resection; and fMR imaging maps can be incorporated into the neurosurgical navigation systems to guide the operation.
The general workflow for clinical functional magnetic resonance imaging
To obtain fMR imaging brain maps effectively, several key measures should be considered in the process. The selection of the fMR imaging paradigms to be used is largely determined by the location of the lesion or intended surgical target, and the relevant local brain function at risk. Assessment of any existing neurologic deficits may inform the possibility of infiltrative neoplasm causing functional deficits that may not be recoverable. Alternatively, intact neurologic function could aid in cautious interpretation of signal voids in peritumoral regions that may be affected by neurovascular uncoupling (NVU), whereby underlying neuronal activity remains intact but the vascular response is attenuated or absent. This topic is discussed later in this article.
Prescan Interview and Training
Reviewing recent and prior imaging studies is crucial for accurate lesion location and spread assessment, planning of the fMR imaging study, and selection of paradigms tailored to the individual patient. Patients’ cooperation and performance are essential to achieve an optimal fMR imaging dataset. Therefore, preprocedure patient training involves necessary steps, including interviewing and assessing the clinical status of the patient, giving detailed instructions, and modifying the paradigm practice to fit the patient’s abilities and limitations, before the scan. MR imaging–compatible corrective glasses may be considered at this time if it is evident that the patient has a refractive error. A detailed explanation of the fMR imaging task commensurate with patient understanding is beneficial, serving to alleviate patient anxiety and increase patient compliance for the study. The incorporation of patient instruction before the fMR imaging scan has been shown to increase the reliability of the data.
Data Acquisition
Because current fMR imaging techniques are limited in spatial resolution, a high-resolution anatomic scan, typically T1 weighted, is necessary to subsequently map the functional activations to structural images. The inclusion of white matter imaging, most commonly diffusion tensor imaging, can be complementary to BOLD fMR imaging.
The stimulus paradigm is typically administered visually by displaying text or instructions via projector-mirror system or via specialized goggles, or by using specialized headphones for tasks with auditory presentation. During fMR imaging studies, patients may be monitored using a video camera to determine compliance for movement tasks, specifically the various motor tasks. Performance on selected language tasks may also be determined by using response systems such as button boxes. , However, the performance of covert language tasks cannot be monitored directly and should be assumed based on the patient performance during the prescan training. Real-time activation maps can be used for a general quality control check ( Fig. 1 ); motion or other artifacts can be readily discerned on real-time maps, and any suboptimal task paradigms may be repeated at this time.
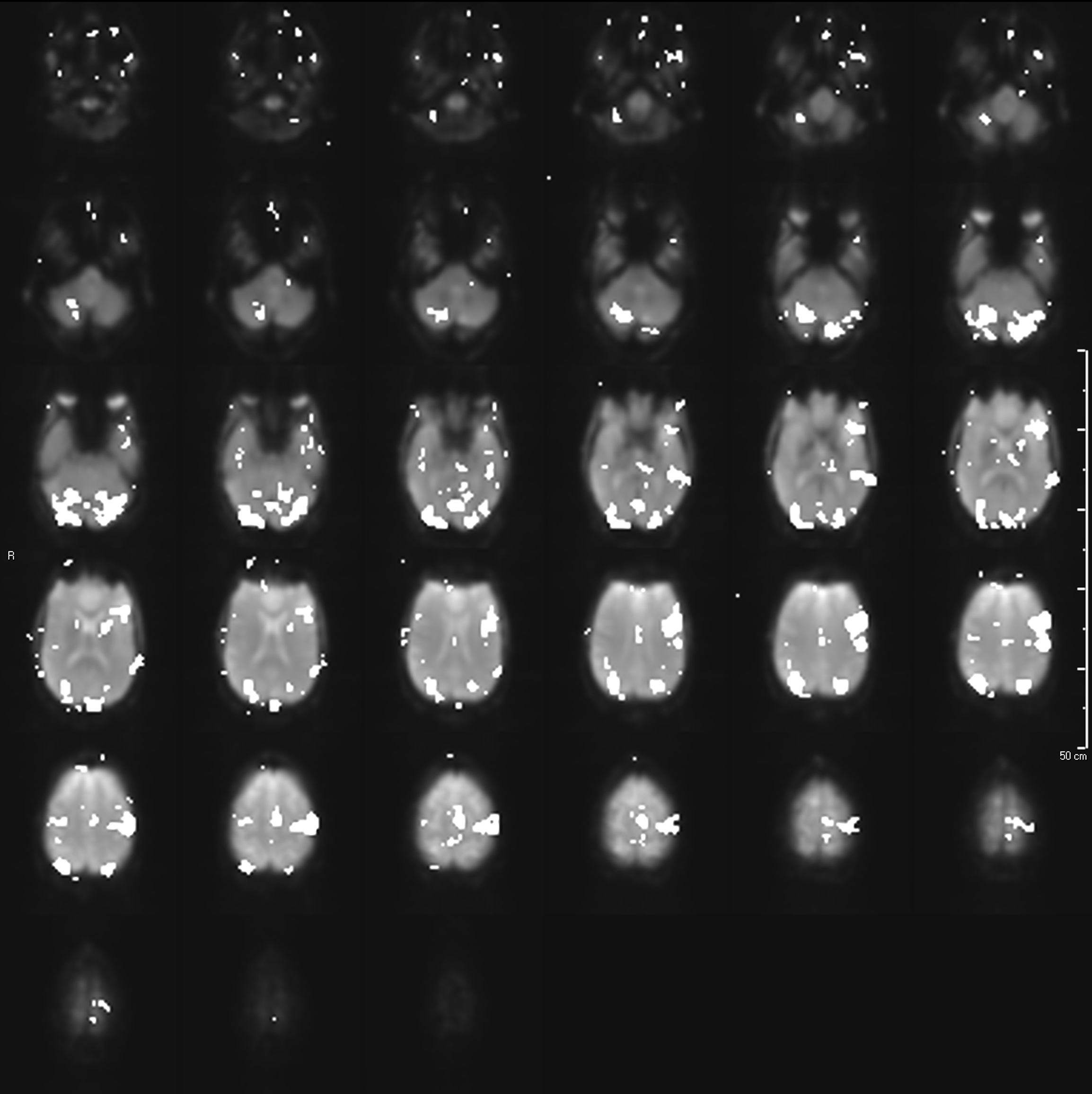
Clinical fMR imaging uses a block design, whereby a specific task is performed for a sustained period of time (routinely 20–30 seconds), called the active period (or task phase), followed by the same period of rest (control phase), where the subject is instructed to stay still (as in a motor task) or performed a control task; the active and rest blocks are then repeated for a specified number of times to generate ample signal. Block designs are generally easier to perform, more efficient, and produce more statistically robust results compared with event-related designs.
Because BOLD fMR imaging is inherently noisy and the signal changes caused by activation are on the order of 3% to 5% from baseline, the repetition of the tasks is necessary to ensure adequate signal to noise. , , , The use of a high field strength is essential; although a 1.5-T MR system is acceptable, the current standard is the use of a 3-T MR imaging scanner. Most functional MR imaging performed clinically uses acquisition of ultrafast images; for example, single shot T2∗ gradient recalled echo echo-planar technique, with a temporal resolution of approximately 2000 milliseconds and spatial resolution of 3 to 5 mm. A typical fMR imaging study paradigm generally acquires between 90 and 120 whole brain volumes in 3 to 4 minutes.
Postprocessing and Quality Control
After data acquisition, several preprocessing steps are needed before the statistical analysis and creation of activation maps. Quality control (QC) analysis is also an essential step, which includes assessment of patient head motion, functional-anatomic alignment, susceptibility artifacts, and data outlier volumes. ,
The minimum processing steps for fMR imaging analysis are motion correction and spatial smoothing. Motion correction is performed on each fMR imaging volume, with the first acquired volume typically as the target. Spatial smoothing is performed using a spatial gaussian filter, typically of the order of 1.5 to 3 times full width at half maximum the native voxel size. fMR imaging data are often detrended to account for linear drifts during scanning. If field maps are obtained, they can be used to correct for susceptibility-related distortion. As an alternative to gradient echo–based imaging, spin echo methods can also be used to minimize these susceptibility artifacts; however, they provide lower BOLD effect and lower contrast and have not been widely used. Slice timing correction can be useful to correct for temporal shifts of image acquisition within a repetition time; however, in clinical practice with block paradigm design, this is not necessary.
Functional Magnetic Resonance Imaging Data Analysis
A regression analysis using a general linear model (GLM) is most commonly used for fMR imaging analysis. From the fMR imaging data, a signal intensity curve is generated over time for each voxel within the brain. As noted, any area of the brain that is active during the task period results in slightly increased BOLD signal because of relative increase in oxyhemoglobin compared with deoxyhemoglobin. An idealized hemodynamic response curve is convolved with the task design to generate a task-specific reference waveform across the acquisition period. Simply put, any voxel that shows similar modulation of signal with the reference curve is determined to be correlated with the task. For each voxel, this reference curve is fitted and those voxels exceeding a preset statistical threshold are considered active. During this regression analysis, additional regressors may be added as confounds. In clinical practice, motion confounds are often not included, because motion time courses may be highly correlated with a task. A design matrix is then constructed that designates beta coefficients of the variables of interest, followed by calculation of contrasts, or weighted combinations of the beta coefficients, to determine the signal of interest. , , For example, in a typical motor task, the contrast of interest is the time period during which the patient moved a specific part of the body. If 2 different active states are present in the task (for example, a widely used 3-phase hand motor task paradigm described later in this article), the contrast may specify activation during movement of 1 of the hands, or activation during movement of either hand.
A typical fMR imaging study comprises approximately 100,000 voxels. A separate GLM analysis is performed for each voxel, which is also referred to as a massive univariate analysis. Given the large number of calculations performed, various methods are used to minimize the activation errors because the surgical resection of areas labeled as no activation may result in significant morbidity. These methods include (but are not limited to) multiple correction methods, such as family-wise error, Bonferroni correction, and false discovery rate, or cluster-based thresholding methods.
Following statistical analysis, activation maps can then be created, and the fMR imaging data coregistered to the patient’s high-resolution anatomic images for localization. At this point, the final activation maps are thresholded, to strike a balance between sensitivity of localization and specificity. No single method of thresholding is widely used, and typically the activation maps are thresholded in a qualitative fashion, to visualize robust activation in an area of interest while minimizing depiction of noisy spurious foci. There have been methods to attempt to standardize the thresholding of activation maps, such as using a percentage of the maximum activation in a region.
Role of Streamlined Postprocessing Tools
Research-level BOLD fMR imaging postprocessing software is very accurate but requires significant experience in image processing as well as computer programming for generation of custom-made scripts for postprocessing; the main disadvantage of using this software is the lack of built in functions to export postprocessed images to PACS (Picture Archiving and Communication System) servers and neuronavigation systems efficiently. There are several commercial US Food and Drug Administration–approved software packages available that are more user friendly, allow better image overlays on high-resolution anatomic images, and are compatible with both PACS servers and neuronavigation software. The coregistered images can be downloaded to the neurosurgical computer. The neurosurgeon can then visualize three-dimensional reconstructions that define the relationship between the lesion and the eloquent cortices to guide the brain tumor resection. Many of these packages, being built on top of research software engines, also provide multiple options for customization such as statistical thresholding, image registration, as well as for QC analysis. At the same time, research software allows for additional processing in situations where the processing with the commercially available packages is inadequate. ,
Commonly used tasks
Motor Tasks
tb-fMR imaging for motor function is frequently used to identify the primary motor cortex, by localizing the 3 main motor areas: foot, hand or fingers, and face/tongue. These areas are arranged in the precentral gyrus medially to laterally following the motor homunculus. Because the hand motor tasks are relatively easy to perform and show robust activation of the motor cortex, these tasks should be performed if the primary motor cortex is to be localized. There are also secondary motor areas that may be important for preoperative planning (ie, the supplementary motor area [SMA] along the medial surface of the frontal lobe) that are also shown on these motor tasks.
Most of the motor tasks that we use at our institution have a block design paradigm with 30 seconds of rest alternating with 30 seconds of activity within each cycle, with visual instructions to stop or go. For foot motor activation, we instruct patients to continuously and gently plantar flex and dorsiflex the foot at the ankle bilaterally during the task period. Occasionally, this causes motion transfer to the head. As an alternative, patients may be instructed to curl their toes (or perform flexion and extension of the toes bilaterally) to minimize bulk body motion. Orofacial tasks can also be challenging because of induction of head motion during the task. We usually perform a vertical tongue movement task where the patient is asked to perform continuous gentle up and down movement of the tongue (alternately touching the roof and floor of the mouth with the tongue), taking care not to move the jaw. If the patient has difficulty with this task, lip puckering may be used as an alternative for eliciting activation in the lower face sensorimotor cortex. For hand sensorimotor activation, a finger movement task including bilateral simultaneous sequential finger tapping is performed with a similar block design, eliciting activation of the somatosensory cortex. The movement tasks often show good activation of the supplemental motor areas in addition to the primary motor cortex in the precentral gyrus. We often use a more complex 3-phase hand-grasp task as an alternative or complementary task for hand motor activation while minimizing sensory activation. In this task, we use a dynamic visual display and the patient is instructed to gently open and close each hand sequentially corresponding with the block length (typically 20 seconds), with an identical rest period following these, repeated multiple times. Fig. 2 shows activation in the primary hand motor area and SMA in addition to the premotor SMA (language).
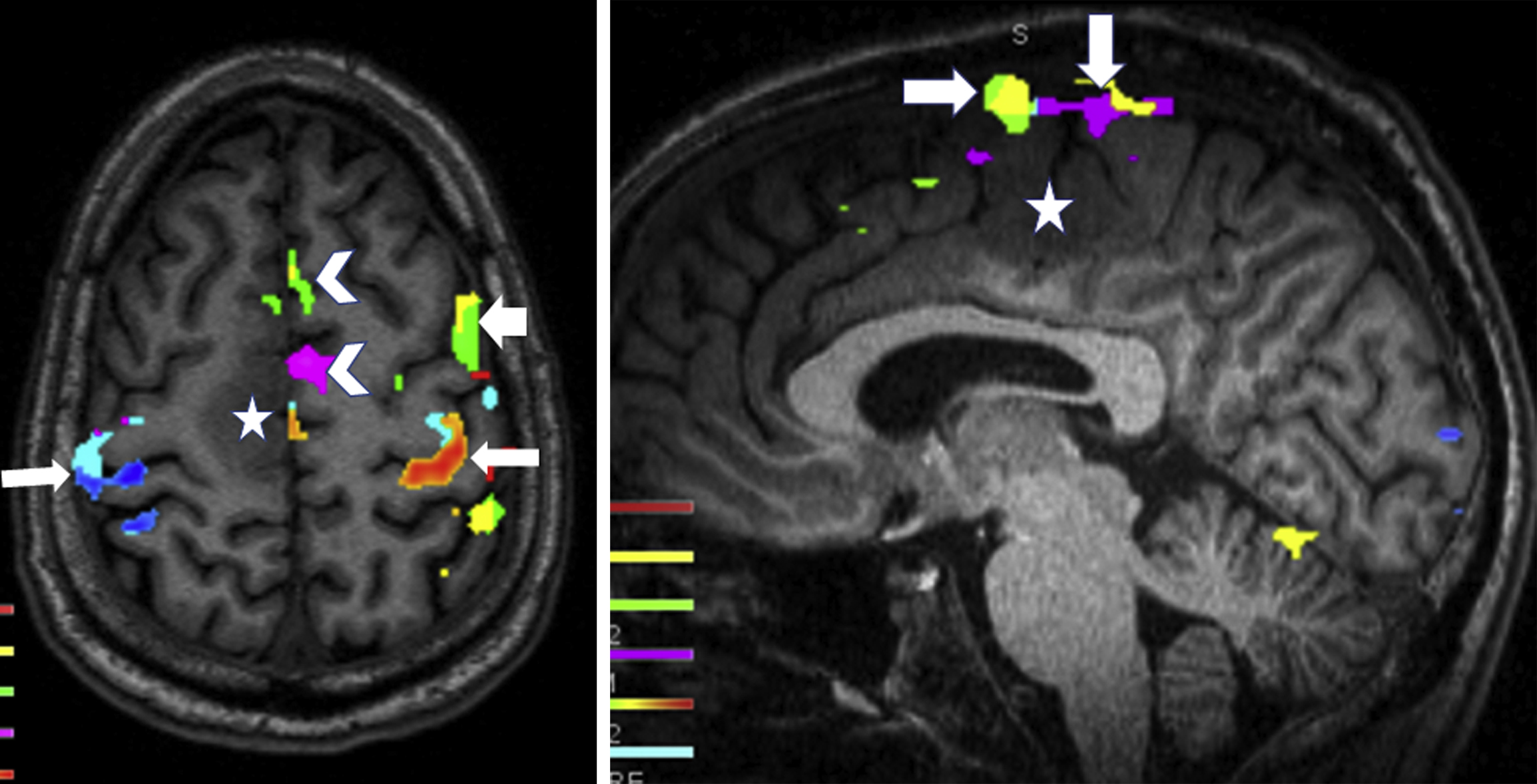
Language Tasks
fMR imaging has been shown to be reliable for the prediction of hemispheric language dominance. Numerous paradigms are typically used to elicit the activation in the eloquent cortex related to language function. Certain paradigms have been specially designed to elicit activation primarily in the speech production areas (expressive paradigms), and in the receptive language regions (receptive paradigms), whereas others may activate both regions. Language activation paradigms may be further divided into semantic, phonological, or verbal fluency paradigms.
Data published on language presurgical fMR imaging studies are varied because of the complexity of language function and different paradigms applied for this purpose in different institutions. Recent studies emphasize the need to evaluate all components of linguistic functions in preoperative mapping, because language evaluations do not account for particular aspects of grammar, even if patients may have postoperative language deficits. Therefore, it is necessary to identify a specific battery of linguistic tests to characterize cortical language representation at the individual level. , It may be necessary to perform additional tasks in multilingual patients.
Based on recent clinical experience using lexical semantic tasks, at least 6 core clinically relevant language areas can be identified using fMR imaging: (1) the Broca’s area, in the posterior third of the inferior frontal gyrus; (2) the Exner’s area, in the posterior middle frontal gyrus; (3) Supplementary speech area (pre-SMA); (4) angular gyrus; (5) the Wernicke’s area, inferior (mid to anterior superior temporal gyrus [STG]) and superior (posterior STG and supramarginal gyrus) components; and (6) basal temporal language area.
In 2017, Benjamin and colleagues studied 22 patients with epilepsy and tumor who underwent Wada test and fMR imaging to determine a clinician-driven individualized thresholding, to reliably identify these 6 language regions. Their results suggest that experienced clinicians can form conjunctions from 2 sets of 3 language tasks to generate equivalent maps by individualized thresholding which these maps differ from and are rated as of better quality than those generated with a fixed threshold. They concluded that a fixed threshold, without expert clinician input, may lead to inaccurate mapping in presurgical planning for language localization.
Language tasks may differ in their sensitivity and specificity, which is another important factor to consider during paradigm selection. Some studies suggest that the use of expressive language paradigms could be associated with greater specificity than receptive and semantic tasks based on this study.
Choice of task paradigm may need to be adjusted for patients with neurologic deficits such as speaking and reading difficulties. Specifically, in patients with reading or visual difficulties, a language task paradigm could be tested via auditory input rather than visual, still activating language, visual, and auditory pathways. Although language dominance is usually left sided, many variations may occur. Surgery involving the mesial temporal lobe ipsilateral to the side of language dominance can result in postoperative memory-related word-finding difficulty, highlighting the value of preoperative fMR imaging assessment.
The ASFNR recommended task algorithms with the aim of balancing varying levels of sensitivity and specificity as well as strengths in lateralization and localization and balancing paradigms that primarily activate frontal/expressive regions (silent word generation [SWG], antonym generation, object naming) and those that primarily activate temporal/receptive areas (sentence completion [SC], passive story listening). In a study using the ASFNR paradigm, SC and SWG tasks were used for language localization and hemispheric lateralization to identify the primary language cortex. The standardized language tasks used were shown to have a high level of intrasubject intrascan repeatability for language mapping in a large cohort of patients undergoing presurgical fMR imaging across several years using both threshold-dependent and threshold-independent approaches. On the ASFNR Web site, parameters for language tasks paradigms are listed, as well as direct download of relevant task PowerPoint files for ASFNR members that can be directly incorporated into the stimulus presentation software.
As per the ASFNR recommendations, the most robust task for language activation is the sentence completion task, which shows good expressive and receptive language activation. The SWG task is a good choice for productive language activation as well as for language lateralization. Additional tasks include the rhyming task, noun-verb association task for productive language activation, and several tasks that preferentially activate receptive areas such as the reading comprehension, listening comprehension, or the passive story listening tasks. The object naming task is also an expressive task that can be used to elicit activation in visual recognition areas centered in the fusiform gyrus; however, this activation can be inconsistent.
There have been recent review articles discussing the current standards of presurgical language mapping using task-based fMR imaging in greater detail. Figs. 3 and 4 show convergent activation on these maps performed with a battery of expressive (productive) and receptive tasks in patients with inferior frontal gyrus (see Fig. 3 ) and STG (see Fig. 4 ) lesions.
