The major part of this section is a pictorial review of cross-sectional fetal brain anatomy using magnetic resonance (MR) imaging matched as closely as possible with postmortem histologic sections. It should be appreciated that by the time the fetal brain has reached 19 to 20 weeks (the earliest fetal images shown in this text), all of the major structural components visible in routine neuroradiologic practice have formed and are clearly visible. Because of this, previous knowledge of adult neuroanatomy can be used to a large extent; indeed, the basic neuroanatomy does not change during this period. The difficulty in interpreting fetal and neonatal imaging arises in the evolving anatomic features of the brain. We discussed the evolution of the “sulcated brain” at the start of Section 1 and myelination is discussed in Section 3 , which covers postnatal imaging. The purpose of this section is to give an introduction to the transient structures in the wall of the developing fetal brain, that is, structures that are not found in the adult, pediatric, or even the term newborn brain.
Transient Structures in the Fetal Cerebral Hemispheres
The original Larroche atlas used anatomic terminology from the Nomina Anatomica of the International Anatomical Nomenclature Committee. As explained in the introduction of the Larroche atlas, some anatomic features are not fully covered by Nomina Anatomica, particularly structures peculiar to the developing brain. The authors made reference to more specific papers, such as the work of Rakic and Yakovlev and Angevine et al. in order to assist with nomenclature. The two main structures that are found in the fetus but not in the brain of adults or children are the subependymal germinative zones (“germinal matrix”) and the transient laminated structures found in the developing cerebral hemispheres. The latter arise from the ventricofugal migration of neurons and glia toward the future cortex and deep gray matter structures. Recently, there has been considerable interest and research in the development of the cerebral cortex in the human fetus. The second trimester is an exceptionally active period of neuronal/glial cell birth, proliferation, and migration. MR imaging has played a role because it allows visualization of the normal structures of the developing cerebral hemispheres, which appear to correspond to the features shown on histologic studies. This has had important clinical repercussions for classifying neocortical brain malformations on pediatric neuroimaging.
One of the striking macroscopic and histologic features of the fetal brain is the presence of large germinal matrices adjacent to the ventricles, which are particularly prominent in the second-trimester fetus. The primary germinal matrix or neuroepithelium is a cell-dense structure that lines the cerebral ventricles. Those cells proliferate extensively and produce neurons, glia, and the secondary germinal matrix. Bayer and Altman found that the secondary germinal matrix (or subventricular zone) produces mainly neurons that are destined to become cortical interneurons and astrocytes. Some of the secondary germinal matrices migrate away from the ventricles and complete their cell-producing role at sites distant from the ventricles. Leading among these are the matrices that form the granule, basket and stellate cells of the cerebellar cortex, and granule cells in the dentate gyrus of the hippocampus.
At some sites in the brain of the second-trimester fetus the germinal matrix is particularly large and is named by the structures that ultimately will be produced. For example, large neuroepithelial/subventricular zones are found around the lateral ventricles and are called the striatal matrices because they will form the putamen and caudate. Feess-Higgins and Larroche used terms such as matrix rhombencephalica , matrix mesencephalica, and matrix telencephalica in their atlas (but labeled simply as matrix in the figures of the original text) to distinguish the anatomic site of the germinal matrix and therefore imply the structures formed from those regions of neural/glial proliferation. In this atlas we simply use the term germinal matrix in our annotation in the hope that which portion of the ventricular system is adjacent is obvious. This simplification does not undervalue the importance of the germinal matrix from either a developmental or an imaging point of view. On the contrary, the germinal matrix is a prominent landmark on fetal MR imaging, particularly in the second trimester, and may hold the key to early detection of some disorders of cortical formation.
The germinal matrix is one of the few structures in the normal fetal brain that has very short T2 values (i.e., appears dark on T2-weighted images). This feature provides superb contrast between the high signal of the cerebrospinal fluid in the ventricles on its deep surface and the intermediate signal of the developing brain superficially. This tissue contrast is exceptionally well seen on postmortem MR (pmMR) because of the lack of time constraints that allow the use of sequences with low echo train lengths and high number of excitations. The contrast resolution of the germinal matrix is sometimes poor on in utero MR (iuMR) using single-shot fast spin echo (SSFSE) sequences. This is partly due to the low sensitivity to susceptibility changes because of the blurring brought about as a result of the high number of echoes in the sequence (T2 decay k-space filtering). The primary and secondary germinal matrices can be shown and differentiated on histologic studies. On MR imaging, however, the two structures cannot be resolved even on high-resolution pmMR because they are so closely opposed and have identical signal characteristics. The pmMR images in this atlas show the primary and secondary matrices clearly on 19- to 20-week, 22- to 23-week, and 25- to 26-week fetuses, and the matrices can often be seen on iuMR at these gestational ages. However, by 29 to 30 weeks, the germinal matrices are less distinct on pmMR, and by 32 to 33 weeks they can be seen only in a minority of sites.
The other transient structures that are of great interest to researchers in the field are the laminar, cellular compartments within the developing cerebral wall that ultimately will govern the organized formation of the cortex and other subcortical gray matter regions of the cerebral hemispheres. Bayer and Altman discuss the historical approach to describing the developing cerebral mantle and explain the new developments in understanding the process. The classic description of the second-trimester cerebral cortex involves only three layers: the deep, periventricular germinal matrix that forms the neurons and glia, the superficial cortical plate (which will become layers 2–6 of the neocortex), and an intermediate zone. The intermediate zone recently has come under particular scrutiny by some groups. Its microscopic anatomy reveals a highly complex, regionally specific pattern called “stratified transitional fields” by Altman and Bayer and the “transient fetal zones” by Kostovic et al. One component of the intermediate zone is the huge number of radial glial cells extending through the full thickness of the hemisphere. Furthermore, Kostovic et al showed that those glial structures guide the migration of cells formed in the germinal matrix to a predetermined site in the developing cortex by a process called fate mapping. Those radial fibers cannot be resolved on MR imaging, although their presence can be inferred in some developmental abnormalities such as focal cortical dysplasias and cortical tubers associated with tuberous sclerosis complex. The observations by Bayer and Altman stress, however, that the intermediate zone should not be viewed as a passive structure, merely allowing the transit of neurons and glia en passant . Instead it is an important region where cell migration is arrested to allow stratification and early synaptic contact. The authors suggest that “precortical” interactions are vital for normal cortical development. By implication the intermediate zone must be an important area of further imaging research in cases where the neocortex does not form properly.
Bland and Altman describe six different regions in the intermediate zone: stratified transitional fields 1 to 6 (i.e., STF1 [superficial] through to STF6 [abutting the germinal matrix]). These strata develop in the first trimester but undergo considerable growth in the second trimester and for the most part have undergone involution in the third trimester, although some portions persist to become the established mature white matter. Histologically, significant differences between the STF strata in regions will become “sensory regions,” with large numbers of granular cells in layer IV (granular cortex) in the mature brain, and the “motor regions” with greater numbers of pyramidal cells in layer V (agranular cortex). In principle, knowledge of the strata and their contents can help explain the regional differences in MR signal seen in the second-trimester fetal brain on pmMR and, to a lesser extent, on iuMR.
STF1 lies just below the cortical plate and is a relatively thick structure. It contains mainly fibrous structures with a large proportion of free extra-cellular fluid and few cell bodies. It has high signal on T2-weighted images in contrast to the low-signal cortical plate superficially. It is seen in both granular and agranular cortices and will become the subcortical white matter. STF2 and STF3 are cell-rich regions and are the last “sojourn” site before neurons and glia enter the cortical plate. STF2 is most prominent in agranular cortex; STF3 is found only in granular cortical regions. Both of these structures have disappeared in the mature brain. STF4, STF5, and STF6 are fibrous, cellular, and fibrous, respectively. STF5 is thought to be the first “sojourn” site of migrating cells; STF4 will become the deep white matter; and the last-to-form STF6 contributes primarily to callosal fibers. These structures are seen well on histologic studies, and STF2 to STF6 can be clearly delineated from STF1 superficially and the deeper germinal matrix on pmMR. Areas of regional heterogeneity within STF2 to STF6 are seen on pmMR, but how they relate to the histologically defined regions has not yet been determined.
It would be of great value if the wealth of information from histologic studies on human fetuses could be used to improve our understanding and interpretation of fetal imaging studies. Interpretation of MR images without direct comparison with histologic studies is fraught with problems; fortunately, Rados et al. have made significant inroads into the subject. Many of the details described here are seen well on pmMR images, particularly on the coronal sections of 19- to 20-week and 22- to 23-week fetuses. Rados et al. used a different nomenclature system for the transient layers in the wall of the cerebral hemispheres of the second- and third-trimester fetus than did Bayer and Altman, what might be considered a more “classic” system. They studied fetuses from all three trimesters, and their overall view of the development of the cerebral cortex is summarized in Table 2-1 .
Gestational Age (Converted to Post Last Menstrual Period) | Main Features | |
---|---|---|
Embryonic | Phase I: 6–9 weeks | Universal embryonic zone |
Early fetal | Phase II: 10–14 weeks | Formation of cortical plate |
Mid fetal | Phase III: 15–17 weeks | Formation of transient fetal zones |
Phase IV: 18–26 weeks | Peak of subplate zone | |
Late fetal | Phase V: 27–38 weeks | Dissolution of transient fetal zones |
Neonate | Phase VI | Immature six-layer cortex |
Rados et al. describe the early fetal brain (10–13 weeks postovulatory weeks, therefore approximately 12–15 weeks post last menstrual period) as having the standard three-layer structure, namely, cortical plate, intermediate zone, and ventricular zone. By the midfetal period (which they defined as 15–22 weeks postovulatory weeks, approximately 17–24 weeks post last menstrual period) the transient zones have developed, and the authors describe seven layers demonstrable on histologic studies. From superficial to deep they are as follows ( Figure 2-1 ):
- 1.
Marginal zone: Not visible in neocortical regions on pmMR studies
- 2.
Cortical plate
- 3.
Subplate zone
- 4.
Intermediate zone
- 5.
Subventricular zone
- 6.
Fiber-rich periventricular zone
- 7.
Ventricular zone: Equivalent to the primary and secondary germinal matrices of Bayer and Altman
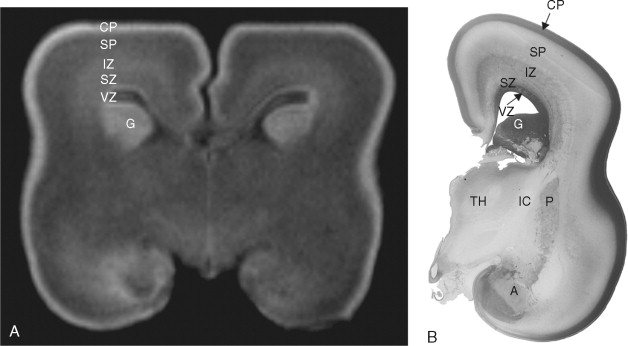
Rados et al. place great importance on the subplate zone in the normal development of the cerebral cortex; it reaches its developmental peak at 27 to 30 weeks postovulatory weeks (approximately 29–32 weeks post last menstrual period). They note that the subplate is the largest single component of the cerebral wall in the second-trimester fetus and that it is proportionally much larger in human fetuses than in fetuses of other mammalian species. Although the subplate does contain cell bodies of both neurons and glia, Rados et al. consider the subplate to be the major “waiting” compartment for fibers that are destined to project to the future mature cerebral cortex. They detailed the major axonal contributors to the subplate in earlier publications , which include thalamocortical projections, projections from the basal forebrain, and ipsilateral and cortico–cortical projections via the corpus callosum. It is suspected that modeling and parcellation of the future cerebral cortex is instigated at this time.
The subplate zone also appears to have a significant effect on the morphologic development of the sulcal/gyral pattern of the future cerebral cortex. Kostovic cites the following supporting evidence:
- •
The subplate zone is much thinner in species that have smooth oligogyric brains in mature animals.
- •
Within the human brain, there is greater gyration in the regions that have the thickest subplate zone.
- •
The frontal lobes continue to develop tertiary gyri postnatally, and this is accompanied by persistence of the subplate zone in those regions.
Rados et al. used their extensive experience in fetal histology to explain the signal characteristics of the transient fetal layers on MR imaging. It should be appreciated that major differences exist between their methods of pmMR and those we present in this atlas. They performed pmMR on brains that had been fixed with aldehyde after removal from the body, whereas we used pmMR on unfixed tissue with the fetal brain still in situ. In those circumstances, they found that T1-weighted images were best for second-trimester fetuses, whereas T2-weighted images gave better results in more mature fetuses. In contrast, we used T2-weighted sequences throughout the gestational age ranges studied. In spite of this, similar interpretation of the signal characteristics likely is valid for our studies as well. The two regions of the second-trimester fetal cerebral hemisphere that have the highest cellular density are the ventricular zone (germinal matrix) and the cortical plate. Those regions returned high signal on the T1-weighted pmMR studies of Rados et al. and low signal on our T2-weighted pmMR studies. This is not surprising because we know from other imaging studies that regions that have high cellular density and high nuclear-to-cytoplasmic ratios (e.g., primitive neuroectodermal tumors and lymphoma) have T1 and T2 shortening in comparison to normal brain. In contrast, the subplate zone has a high proportion of extracellular components that are intensely hydrophilic. Therefore the high water content in the subplate zone is responsible for the low signal on T1-weighted images and the high signal on T2-weighted images. This is true at least in fetuses at 30 weeks’ gestation post last menstrual period, but from then on the disappearance of the extracellular, hydrophilic matrix produces blurring between the subplate and intermediate zone (and to a lesser extent between the subplate and cortical plate).
The appearance of the other transient layers of the second-trimester fetus (intermediate zone, fiber-rich periventricular zone, and subventricular zone) is more difficult to resolve on MR. This is because of the reduced inherent contrast resolution between the adjacent layers and intense regional and temporal variations. Judging by the images of the second-trimester fetus shown in the Rados paper, T1-weighted images of fixed tissue appear to discriminate between those structures better than T2-weighted images of unfixed tissue. However, the basic principles appear to hold true: regions with high cellularity (e.g., cortical plate) have comparatively high signal on T1-weighted images and low signal on T2-weighted images, whereas the reverse signal pattern is seen in cell-sparse regions (subplate zone). These features are summarized in Table 2-2 .
Zone | Predominant Histology | T1W Signal | T2W Signal |
---|---|---|---|
Cortical plate | Cell dense | ↑ | ↓ |
Subplate | Extracellular hydrophilic matrix | ↓ | ↑ |
Intermediate | Cellular | ↑ | ↓ |
Subventricular | Cell sparse | ↓ | ↑ |
Ventricular | Cellular | ↑ | ↓ |
The reasons why the rapid SSFSE T2-weighted sequences used for in utero fetal imaging discriminate between germinal matrix and fetal brain with less clarity than do short echo train length FSE T2-weighted sequences used for pmMR have already been discussed. Those arguments also hold true for the transient fetal structures of the developing cerebral hemisphere. That is not to say that they cannot be seen in utero, because in some cases they can, but in our experience not in a robust fashion. The development of the germinal matrix and transient hemispheric structures must hold the key to the abnormal development of many cortical malformations, and this warrants further research and development of imaging methods to show those structures with greater clarity. This is not a problem in fetuses postmortem, and interesting features can be shown in developmental abnormalities. An example is shown in Figure 2-2 .
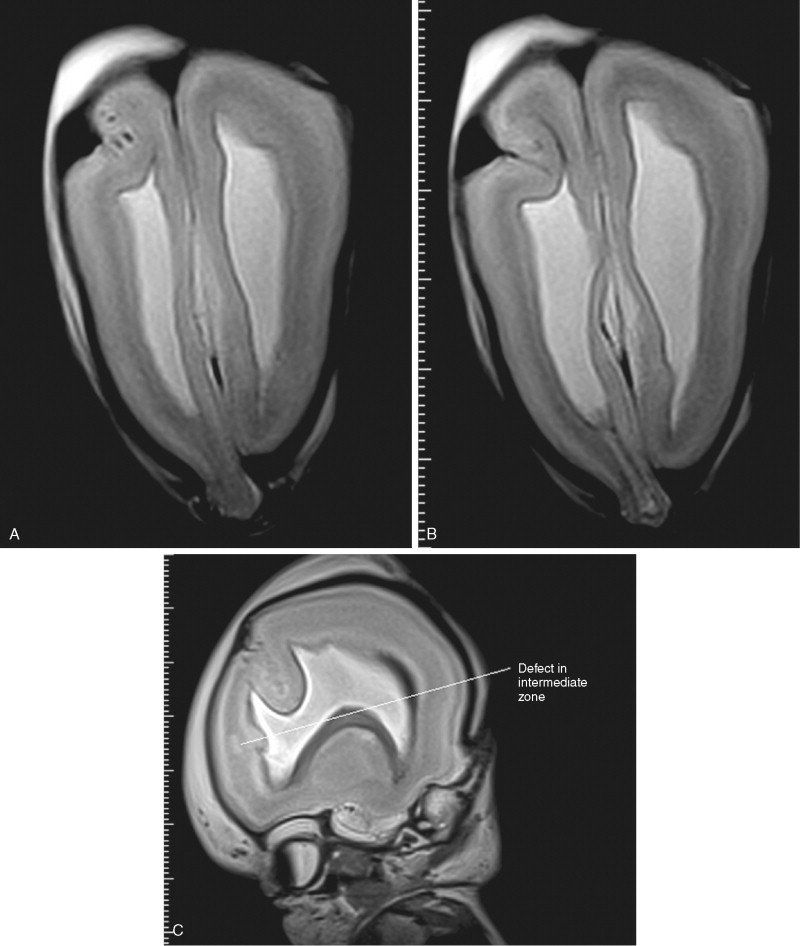
Some improvements have been made in delineating the transient zones of the fetus using iuMR, particularly with refinements of diffusion-weighted imaging (DWI). This is a difficult sequence to use in utero but has been shown to be possible by many groups. Most frequently DWI is performed with an echoplanar imaging method using its “ultrafast” capability. The signal contrast produced on DWI and the associated apparent diffusion coefficient (ADC) map is dependent on how freely water can diffuse on a microscopic scale. In regions where water diffusion is restricted, DWI shows very high signal matched by low-signal (low-diffusion) regions on the ADC maps ( Figure 2-3 ). It is possible that further refinement of such techniques will contribute to improved early detection of subtle abnormalities of neocortical development.
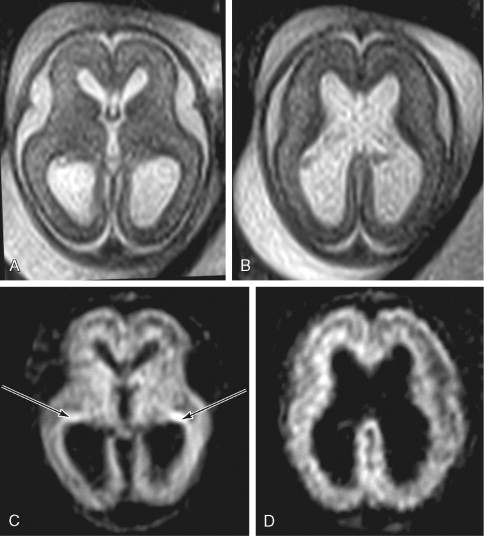
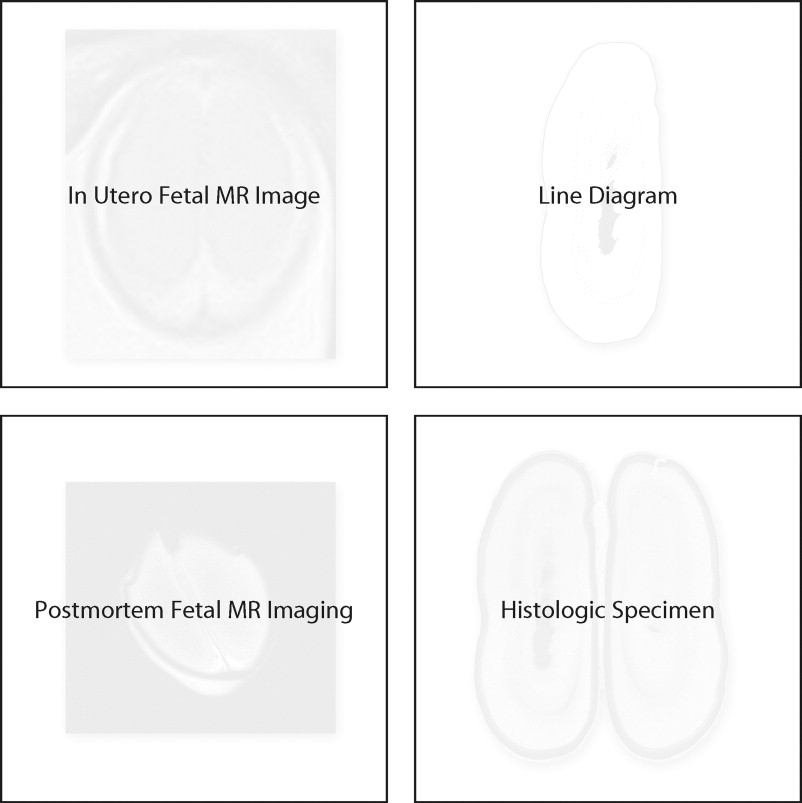
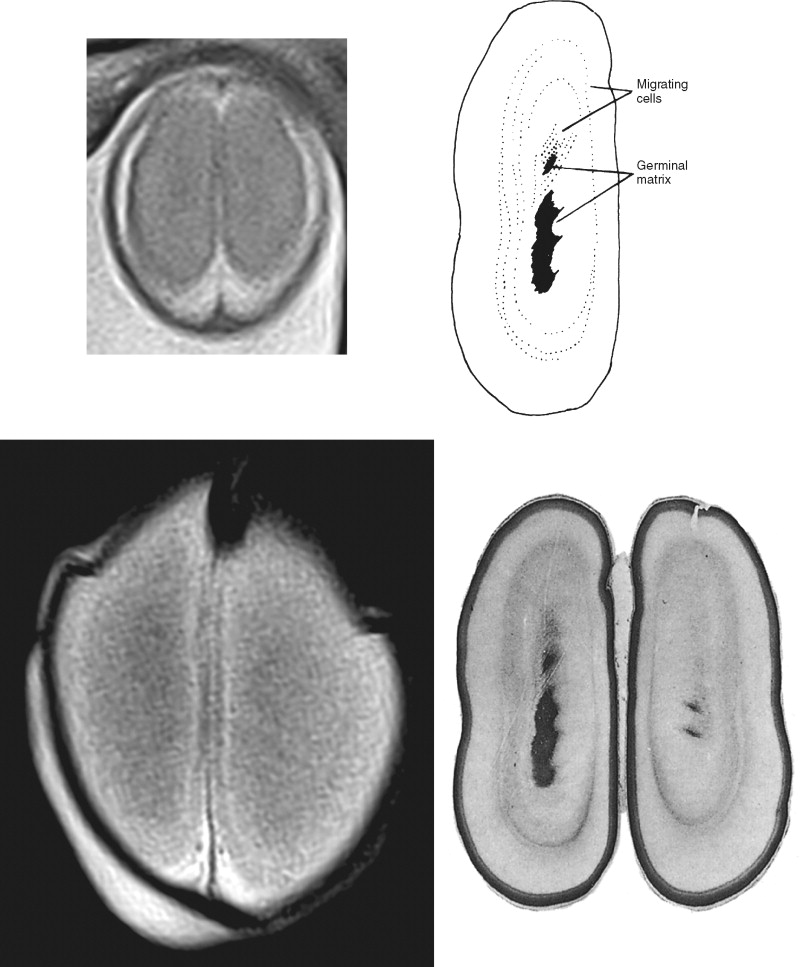
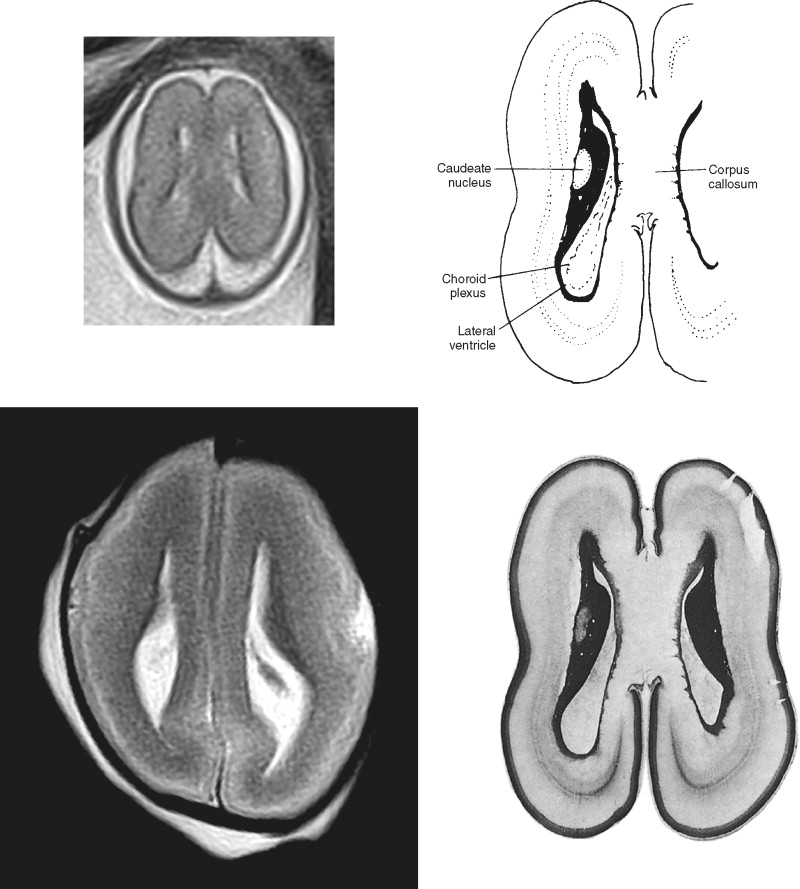
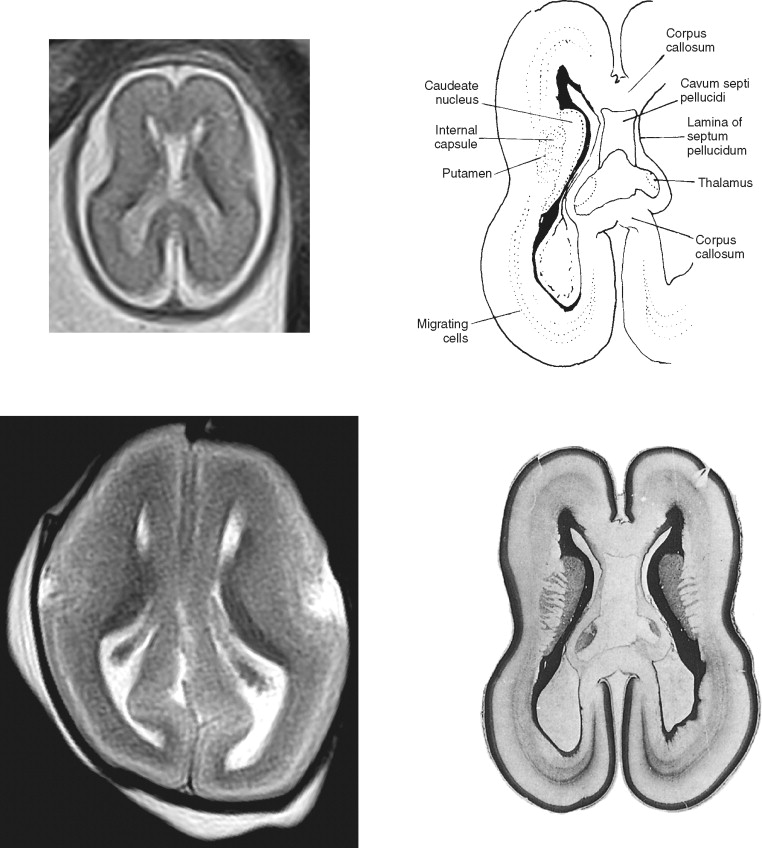
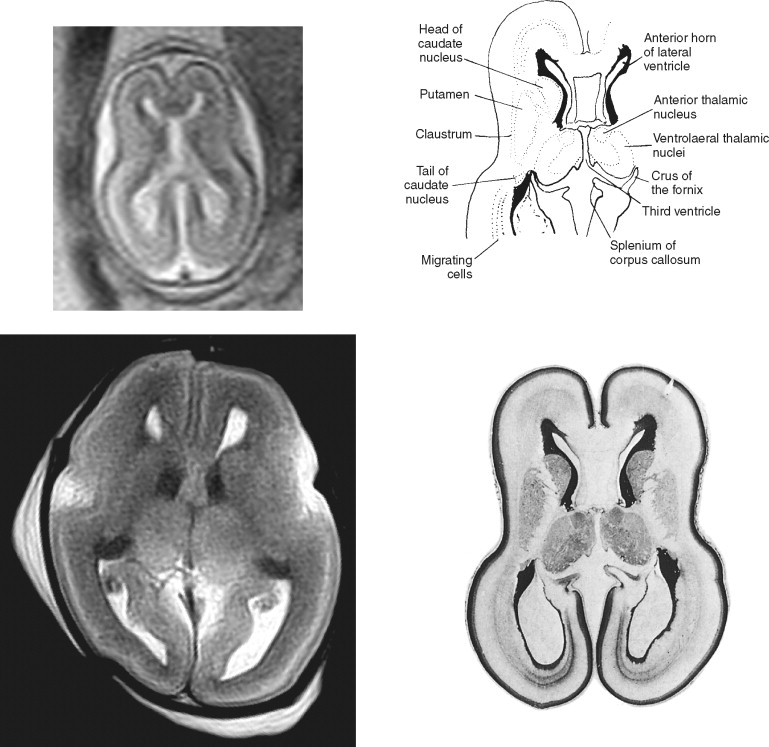
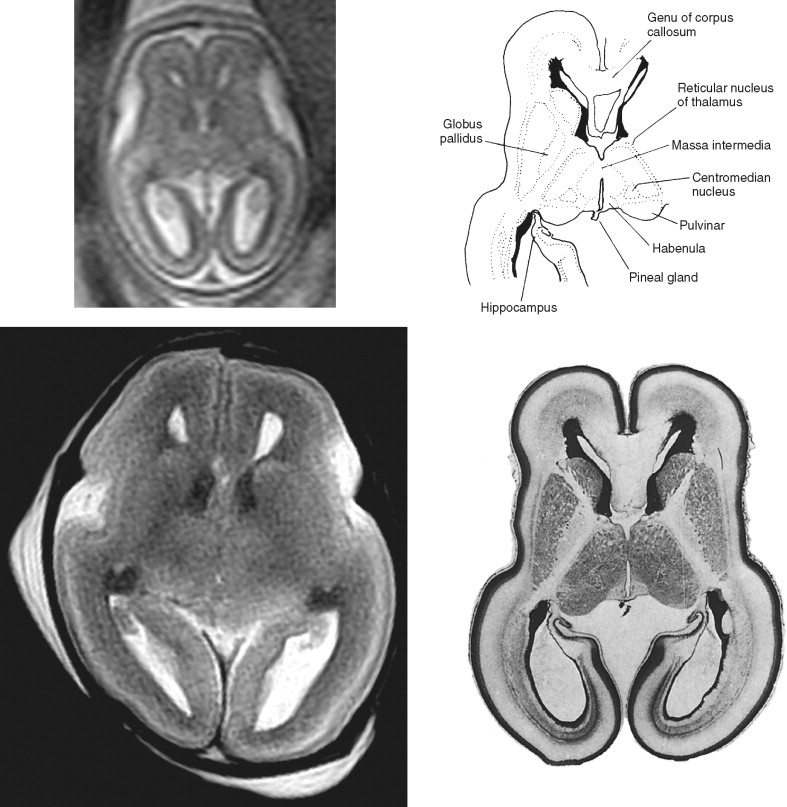
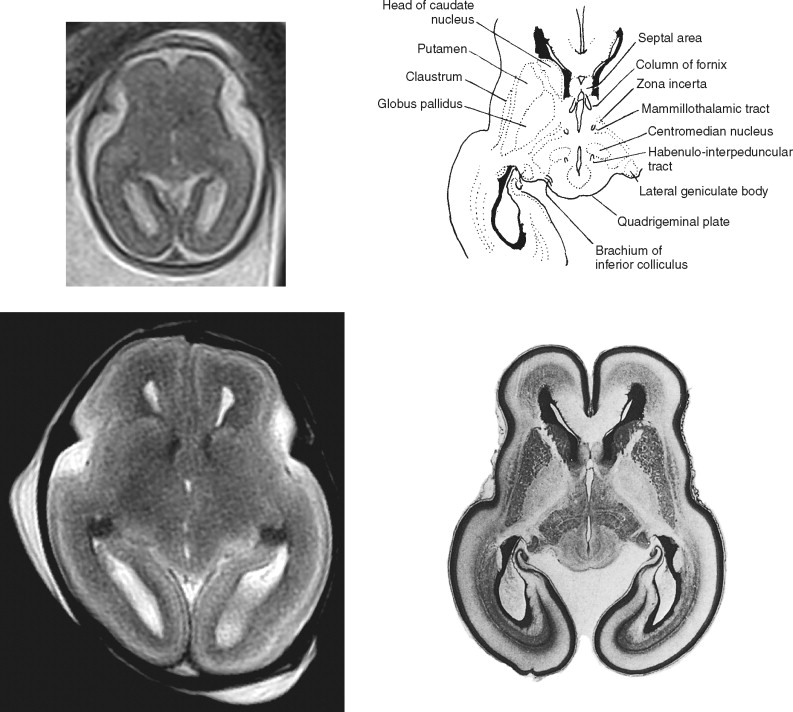
