The role of cardiovascular magnetic resonance (CMR) in the evaluation of infants and children with congenital heart disease is now widely accepted as a result of ongoing technologic advances and the growing realization of its clinical value. Improvements in CMR hardware and the development of new, highly efficient/faster imaging methods, have allowed for sufficient spatial and temporal resolution to comprehensively evaluate the complex cardiac anatomy and function in pediatric patients despite their small body size and rapid heart rate. Although transthoracic echocardiography (TTE) provides much of the necessary noninvasive diagnostic information for most patients in this age group, CMR offers an important alternative with added value in several circumstances: (1) when TTE fails to provide the required diagnostic information; (2) when clinical assessment and diagnostic tests are inconsistent; (3) as an alternative to diagnostic invasive x-ray cardiac catheterization with its associated higher risk and cost; and (4) to obtain diagnostic information for which CMR offers unique advantages (e.g., flow measurements, fibrosis, parametric mapping).
This chapter reviews the anatomy, clinical management, and CMR evaluation of common congenital heart disease lesions involving infants and children. Patient preparation, sedation, and monitoring strategies for children undergoing CMR are discussed in Chapter 12 .
Principles of Cardiovascular Magnetic Resonance Evaluation in Congenital Heart Disease
Preexamination planning is crucial given the wide array of CMR imaging sequences available and the often-complex nature of the clinical, anatomic, and functional issues in patients with congenital heart disease. It is essential to perform a careful review of the patient’s medical history, including details of all prior cardiovascular surgical procedures, interventional catheterizations, diagnostic test results, and current clinical status. As with TTE and invasive cardiac catheterization, CMR examination of congenital heart disease is a dynamic diagnostic procedure that is optimally performed with continuous review and interpretation of the data by the supervising physician. Unexpected findings or suboptimal image quality often require adjustment of the examination protocol, imaging planes, techniques, and sequence parameters. Reliance on standardized protocols and postexamination review alone in these patients may result in incomplete or even erroneous interpretation, or the need to repeat the CMR examination.
One of the strengths of CMR is its ability to accurately and precisely measure ventricular and vascular structures. Care must be taken, however, when determining whether these measurements fall within the normal range because adjustment for the variation in body size remains challenging. Simply dividing by body surface area (BSA) is inadequate for most parameters, particularly in infants and children but also in unusually large or small adults. Several investigators have demonstrated that linear dimensions (e.g., ascending aorta diameter) are best adjusted to the square root of BSA, area measurements to BSA, and volumetric measurements to BSA raised to the 1.3 to 1.4 power. Thus for example, one cannot simply apply to children a normative range for ventricular volume indexed to BSA that was derived in adults. CMR-based normative data from healthy children are available, although they are often limited by a smaller than ideal sample size, particularly in the youngest age range. Furthermore, it is essential to apply the same measurement technique (e.g., whether to include papillary muscles or myocardial trabeculations in the blood pool) as that which was used to derive the normative data.
Atrial Septal Defects and Other Interatrial Communications
Anatomically, five different defects can result in an interatrial shunt ( Fig. 40.1 ):
- 1.
A patent foramen ovale is bordered on the left by septum primum and by the superior limbic band of the fossa ovalis (septum secundum) on the right. A patent foramen ovale is seen in almost all newborns and decreases in frequency with age.
- 2.
A secundum atrial septal defect (ASD) ( Fig. 40.2 ) is the most common cause of an atrial level shunt after patent foramen ovale. Usually the defect is caused by deficiency of septum primum, but rarely, it results from a deficiency of septum secundum.
FIG. 40.2
Secundum atrial septal defect. (A) Oblique balanced steady-state free precession (bSSFP) cine showing a centrally located defect (arrow) . (B) Color-coded velocity-encoded cine showing flow from the left atrium (LA) to the right atrium (RA) through the defect. IVC, Inferior vena cava; RPA, right pulmonary artery; SVC, superior vena cava.
(From Geva T, Powell AJ. Magnetic resonance imaging. In: Allen HD, Shaddy RE, Penny DJ, Feltes FT, Cetta F, eds. Moss and Adams’ Heart Disease in Infants, Children, and Adolescents. 9th ed. Philadelphia: Wolters Kluwer; 2016:373–412.)
- 3.
A primum ASD is a variant of incomplete common atrioventricular canal and is the third most common interatrial communication. This defect involves the septum of the atrioventricular canal and is almost always associated with a cleft anterior mitral valve leaflet. Any associated defect within the fossa ovalis (e.g., secundum ASD) is regarded as a separate abnormality.
- 4.
A sinus venosus septal defect results from deficiency of the sinus venosus septum, which separates the pulmonary veins from the systemic veins and the sinus venosus component of the right atrium (RA) ( Fig. 40.3 ). Most commonly, a sinus venosus defect is between the right upper pulmonary vein and the cardiac end of the superior vena cava. Rarely, the defect involves the right lower and/or middle pulmonary veins and the inferior aspect of the RA near its junction with the inferior vena cava. From an anatomic standpoint, a sinus venosus defect is not an ASD because it does not allow direct communication between the left and right atria. Instead, the interatrial flow travels between the left atrium (LA), one or more of the pulmonary veins, the sinus venosus septal defect, the superior (or inferior) vena cava, and the RA. The defect usually allows pulmonary vein flow to drain to the RA through the defect as well. Patients with sinus venosus defects may have additional accessory right upper pulmonary veins connecting to the superior vena cava or azygous vein.
FIG. 40.3
Sinus venous atrial septal defect. (A) Volume rendered contrast-enhanced three-dimensional magnetic resonance angiography showing several pulmonary veins from the right upper lobe draining into the superior vena cava. (B) Cine balance steady-state free precession (bSSFP) in the sagittal plane showing the defect (*) between the right upper pulmonary vein (RUPV) and the superior vena cava (SVC). (C) Cine bSSFP in the axial plane showing the defect (*) between the RUPV and the SVC . The arrow points to the left atrial orifice of the RUPV. A left-to-right shunt results from drainage of the RUPV to the SVC and from left atrial blood entering the right atrium through the orifice of the RUPV (arrow) and the unroofed wall between the RUPV and the SVC (*). Ao, Ascending aorta; LA, left atrium.
(From Wald RM, Powell AJ. Congenital heart disease. In: Kwong R, ed. Cardiovascular Magnetic Resonance Imaging. Totowa, NJ: Humana Press; 2008:537–568.)
- 5.
A coronary sinus septal defect is a rare type of interatrial communication in which the septum between the coronary sinus and the LA is either partially or completely unroofed, allowing the RA and the LA to communicate through the defect and the coronary sinus orifice. Sometimes there is also a persistent left superior vena cava draining to the coronary sinus. If the coronary sinus is completely unroofed, the left superior vena cava will drain to the LA. The coexistence of a coronary sinus septal defect and persistent left superior vena cava is termed Raghib syndrome, and may result in cyanosis.
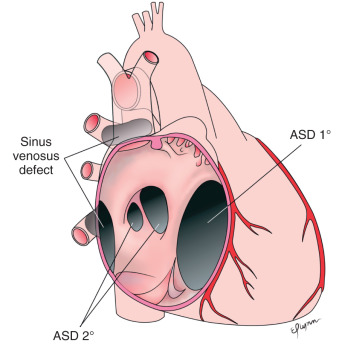
Regardless of the specific anatomic type, the amount of shunting through an interatrial communication is determined by the defect size and relative compliance of the right ventricle (RV) and left ventricle (LV). Over the first few months of life, RV compliance typically rises, leading to an increasing left-to-right shunt. During adulthood, LV compliance normally decreases further, augmenting the left-to-right flow. Shunt flow through the right heart and lungs leads to dilation of the RA, RV, pulmonary arteries, and pulmonary veins. Up to 5% to 10% of patients with a significant left-to-right shunt may develop pulmonary vascular disease by adulthood, leading to pulmonary artery hypertension. Adults with unrepaired atrial level defects are also at risk for exercise intolerance, atrial arrhythmias, and paradoxical emboli.
In general, current practice is to refer patients for interatrial defect closure if the patient is symptomatic or the defect results in a significant left-to-right shunt. Evidence for the latter includes a defect diameter >5 mm, RV cavity dilation, flattening of the interventricular septum in diastole (caused by elevated RV diastolic pressure from the volume load), and a pulmonary-to-systemic flow (Qp/Qs) ratio >1.5 to 2. Although a patent foramen ovale typically produces only a small shunt, closure may be indicated when there is a history consistent with a paradoxical embolus. All of these defect types can be closed surgically with a very low mortality and morbidity in centers with expertise. Over the past two decades, transcatheter treatment for secundum ASDs and patent foramen ovale by occluding them with various devices has become available at specialized centers.
With regard to surgical technique, secundum ASDs are either closed primarily or with a patch. For primum ASDs, the defect is closed with a patch and, in the majority of cases, the associated mitral valve cleft is partially sutured. Sinus venosus ASDs can often be closed by placing a patch to reconstruct the missing portion of the sinus venosus septum, thereby eliminating interatrial and right pulmonary vein to right atrial flow (“single patch repair”). A second patch may be placed anteriorly on the superior vena cava to enlarge it (“two patch repair”). In some cases, especially when a right upper pulmonary vein drains relatively high to the superior vena cava, the superior vena cava is transected superior to the anomalous veins and the distal caval end anastomosed to the right atrial appendage (Warden procedure). The sinus venosus septal defect is then closed in such a way that the proximal superior vena cava and anomalous veins drain to the LA. Short-term outcomes for all three techniques are excellent, but caval or pulmonary venous obstruction may develop over time. For a coronary sinus septal defect, the os of the coronary sinus is usually patched closed. If a left superior vena cava is present, it is redirected to the right atrial side either through ligation when an adequate left innominate vein is present, or via a baffle to the RA.
Cardiovascular Magnetic Resonance
Before Closure
In children, TTE is the primary imaging technique for the evaluation of ASDs and is usually sufficient for clinical decision making. However, CMR can be helpful in older patients, usually adolescents and adults, with a known or suspected ASD and inconclusive clinical or TTE findings. CMR is also a useful noninvasive alternative to transesophageal echocardiography for determining which patients are candidates for percutaneous device closure rather than surgery.
Studies have shown that cine balanced steady-state free precession (bSSFP) imaging and velocity-encoded cine imaging are able to accurately identify ASD size, shape, proximity to adjacent structures, and associated findings (e.g., anomalous pulmonary venous return). Similarly, CMR is useful for diagnosis and preoperative planning in patients with sinus venosus ASDs. Moreover, multiple reports have demonstrated that calculation of the Qp:Qs ratio by measuring flow in the main pulmonary artery (Qp) and ascending aorta (Qs) agrees closely with invasive catheterization-based oximetry measurements of Qp:Qs. In the absence of significant valve regurgitation or an additional shunt, the Qp:Qs ratio can also be derived from the right (Qp) and left (Qs) ventricular stroke volumes measured from the short-axis cine ventricular stack. In clinical practice, it is recommended to measure the Qp:Qs ratio by both of these methods and check the data for consistency. In addition, review of the ventricular short-axis cine images also allows one to make a qualitative estimate of RV systolic pressure based on the configuration of the ventricular septum. The septal geometry is concave toward the RV when the RV-to-LV pressure ratio is low and assumes a flat configuration, or even a concave shape toward the LV, as the RV-to-LV pressure ratio increases. Interpretation of the septal configuration may be confounded by factors such as dysynchronous contraction, conduction delay (e.g., right or left bundle branch block and preexcitation), and a high LV pressure.
The goals of the CMR examination include (1) delineating ASD number, location, size, and rim length; (2) evaluating pulmonary venous return; (3) estimating the RV systolic pressure based on septal configuration; (4) measuring the Qp:Qs ratio; and (5) quantifying biventricular volumes, mass, and function. These goals can be achieved by the following protocol :
- •
Cine bSSFP: stack of contiguous thin slices in an axial (sinus venosus defect) or four-chamber plane (secundum ASD) to span the interatrial septum and cardiac ends of the vena cavae.
- •
Cine bSSFP: stack of contiguous thin slices in an oblique sagittal plane perpendicular to the interatrial septum to span the septum and entry of the vena cavae ( Fig. 40.4 ).
FIG. 40.4
Atrial septal defect (ASD) imaging protocol. (A) Axial cine balanced steady-state free precession (bSSFP) image showing a large secundum ASD (white arrow) . (B) The axial bSSFP image is used to plan a stack of oblique sagittal cine bSSFP images to visualize the ASD and the superior and inferior defect margins. (C) The axial and oblique sagittal images are used together to plan a stack of velocity-encoded phase contrast (PC) cine images to visualize the ASD flow en face. This provides insight into the oval shape of the defect and may demonstrate additional ASDs.
(From Fratz S, Chung T, Greil GF, et al. Guidelines and protocols for cardiovascular magnetic resonance in children and adults with congenital heart disease: SCMR expert consensus group on congenital heart disease. J Cardiovasc Magn Reson . 2013;15:51.)
- •
Velocity-encoded cine: 1 to 3 contiguous slices positioned parallel to the interatrial septum with through-plane velocity encoding to obtain an en face view of the defect (see Fig. 40.4 ).
- •
Velocity-encoded cine: stack of contiguous thin slices in a four-chamber and/or in an oblique sagittal plane to span the interatrial septum and with velocity encoding in the direction of ASD flow to visualize the ASD (see Fig. 40.2B ).
- •
Cine bSSFP: ventricular long-axis planes and a ventricular short-axis stack from base-to-apex for quantitative assessment of ventricular volumes and ejection fraction (EF).
- •
Contrast-enhanced three-dimensional (3D) magnetic resonance angiography (MRA) and/or electrocardiogram (ECG) and respiratory navigator-gated 3D bSSFP imaging of the thorax.
- •
Blood flow measurements: ascending aorta (Qs) and main pulmonary artery (Qp); optional, superior vena cava and descending aorta at the diaphragm (Qs), and left and right pulmonary arteries (Qp).
After Closure
The CMR examination goals after ASD closure are similar to the preprocedure ones. Additional aims in patients who have undergone transcatheter device closure of an ASD include excluding device malposition, device interference with the atrioventricular valves and venous blood flow, and thrombus formation. Patients who have undergone a repair of a sinus venosus defect are at risk for superior vena cava and right pulmonary vein obstruction. Mitral regurgitation from a residual mitral valve cleft is common after primum ASD repair, and should be assessed quantitatively. All of these aims can be accomplished using the imaging protocol above before closure.
Ventricular Septal Defects
A ventricular septal defect (VSD) is a communication between the RV and LV through an opening in the interventricular septum. Several VSD anatomic classification systems are in use. Fig. 40.5 shows one such system modified from Van Praagh et al., which includes the following: (1) defects at the junction between the conal septum and the muscular septum bordering the membranous septum (referred to as membranous defects) or associated with malalignment of the conal septum (conoventricular defects) ( Fig. 40.6 ); (2) defects in the muscular septum (called muscular or trabecular defects) ( Fig. 40.7 ); (3) defects in the inlet septum (known as atrioventricular canal-type or inlet defects); and (4) defects in the outlet septum (variably called outlet, doubly committed subarterial, subpulmonary, conal septal, or supracristal defects).
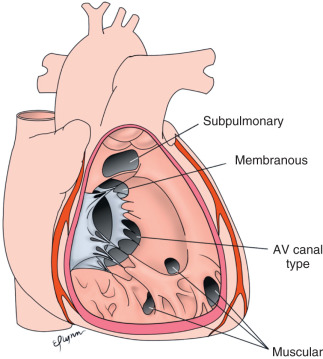
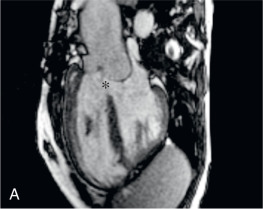
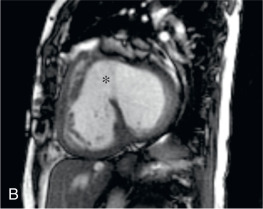
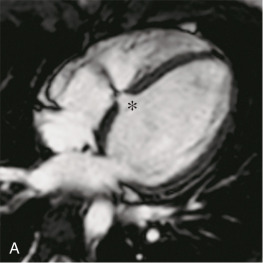
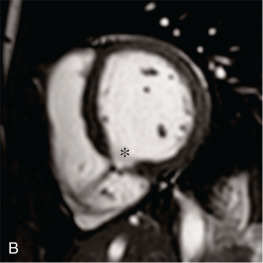
The natural history of a VSD relates to the size and location of the defect. Defects in the membranous or muscular septum often become smaller over time and may spontaneously close. In contrast, malalignment conoventricular defects, outlet septum defects, and atrioventricular canal-type defects are usually large and rarely spontaneously close. Consequently, these patients often undergo surgical closure in infancy. Venturi effects associated with VSDs in the membranous or outlet septum may cause aortic valve leaflets (usually the right coronary leaflet) to prolapse through the defect. Although the leaflet prolapse typically reduces the effective orifice size of the defect, it may also lead to aortic regurgitation. VSDs, particularly those associated with turbulent flow jets, also predispose patients to the development of endocarditis.
Symptoms are predominantly determined by the size of the shunt through the VSD, which, in turn, is related to the defect size and the relative resistances of the pulmonary and the systemic vascular beds. In most situations, pulmonary resistance is lower than systemic resistance, and there is a left-to-right shunt. The resulting increased blood flow to the lungs and left heart may lead to dilation of the pulmonary arteries, pulmonary veins, LA, and LV. Because most of the shunt flow passes through the VSD into the RV during systole, RV dilation is usually not present. If the shunt is small and the patient is asymptomatic, an intervention to close the defect is not warranted. When the shunt is large, symptoms from pulmonary overcirculation (e.g., tachypnea, diaphoresis, poor feeding, and slow weight gain) may develop in the first few months of life. If the defect is unlikely to become small over time or if these symptoms cannot be managed medically, surgical closure in infancy is recommended. Untreated, patients with VSDs and large left-to-right shunts may develop irreversible pulmonary artery hypertension from elevated pulmonary vascular resistance. In such cases, the flow through the defect will become increasingly right-to-left, resulting in cyanosis (Eisenmenger syndrome).
A surgical approach is by far the most common technique used to close VSDs and carries a low mortality risk even when performed in the first few months of life. Typically, the surgeon works through the tricuspid valve and applies a patch to cover the defect. In selected patients, such as those with apical defects, a limited right ventriculotomy may be required for effective closure. Experience with transcatheter delivery of occlusion devices is growing, and this approach may be appropriate in selected circumstances.
Cardiovascular Magnetic Resonance
As mentioned, TTE is the primary clinical diagnostic imaging modality in patients with suspected or known VSD, and is usually adequate. Occasionally, in larger patients, acoustic windows may be insufficient and CMR is indicated to define the defect size and location as well as identify associated conditions such as aortic valve prolapse. CMR may also be of use when the hemodynamic burden of a defect is uncertain by providing reliable quantitative data on the Qp:Qs ratio, and on ventricular volumes and function. Moreover, CMR has been shown to be an important, noninvasive test in the diagnosis and management of defects in the outlet septum when TTE assessment of this region is inadequate.
VSD location and size can be demonstrated by cine gradient recalled echo (GRE) or spin echo sequences. Very small defects may be difficult to resolve; however, the associated turbulent flow can be made conspicuous on GRE sequences provided the echo time (TE) is long enough to allow for sufficient spin dephasing (see Fig. 40.7 ). It is useful to assess the ventricular septum using stacks of images oriented in at least two planes. The four-chamber plane provides base-to-apex localization, whereas the short-axis plane shows the location in the superior-to-inferior axis (see Figs. 40.6 and 40.7 ). Also, as described in the section on ASDs, ventricular septal configuration can be used to estimate RV systolic pressure. Finally, quantification of the VSD shunt should be performed by calculating the Qp:Qs ratio. This can be accomplished by measuring the net blood flow in the main pulmonary artery (Qp) and the ascending aorta (Qs) using velocity-encoded cine CMR. Alternatively, in the absence of significant valve regurgitation or other shunts, the ventricular volumetric data can be used. The Qp:Qs ratio is equal to the RV stroke volume divided by the LV stroke volume. In practice, both approaches are recommended and the two results should be compared for consistency.
The goals of the CMR examination include (1) delineating VSD number, location, and size; (2) measuring the Qp:Qs ratio; (3) estimating the RV pressure by evaluating the interventricular septal configuration; (4) calculating ventricular volumes, mass, and EF; and (5) quantifying aortic valve regurgitation if significant. These goals can be achieved by the following protocol:
- •
Cine bSSFP: stack of contiguous thin slices in a four-chamber plane to span the interventricular septum for VSD visualization.
- •
Cine bSSFP: ventricular short-axis stack from base-to-apex for VSD visualization and quantitative assessment of ventricular volumes and EF.
- •
Velocity-encoded cine: 1 to 3 contiguous slices positioned perpendicular to the ventricular septum with velocity encoding in the direction of VSD flow to visualize the defect.
- •
Blood flow measurements: ascending aorta (Qs) and main pulmonary artery (Qp); optional, superior vena cava and descending aorta at the diaphragm (Qs), and left and right pulmonary arteries (Qp).
Patent Ductus Arteriosus
The ductus arteriosus is a vascular channel that usually connects the aortic isthmus with the origin of the left pulmonary artery ( Fig. 40.8 ). During fetal life, the ductus arteriosus allows the majority of the RV output to bypass the lungs by carrying blood flow to the descending aorta. Normally, the ductus arteriosus closes shortly after birth. A persistent patent ductus arteriosus (PDA) is common in premature infants and is associated with increased morbidity. In full-term infants and children, the clinical course and sequelae of an isolated PDA are usually related to the ductus size and the direction of blood flow. In the absence of elevated pulmonary vascular resistance, isolated PDAs have left-to-right flow, leading to increased pulmonary artery flow, and a volume load on the left heart. Larger PDAs cause a significant left-to-right shunt and, if untreated, lead to pulmonary overcirculation, respiratory distress, growth failure, and, eventually, pulmonary vascular disease. Smaller PDAs pose little hemodynamic burden but place the patient at risk for infective endarteritis; the benefit of closure in older children or adults is debated. When indicated, PDA closure can be accomplished either surgically or in the catheterization laboratory using occluding devices. The latter is far more common in situations of an isolated PDA.
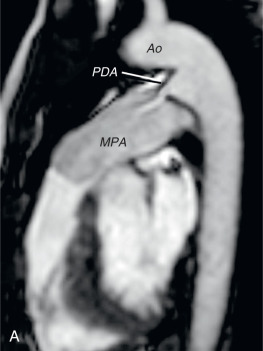
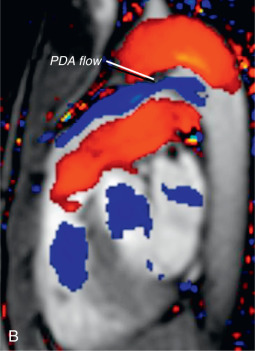
Cardiovascular Magnetic Resonance
CMR is seldom requested primarily for assessment of an isolated PDA because this usually presents in childhood and is a straightforward TTE diagnosis. In several types of complex congenital heart disease, evaluation of the ductus arteriosus is an important element of the examination. For example, in patients with tetralogy of Fallot (TOF) and pulmonary atresia, the ductus arteriosus may persist and be a significant source of pulmonary blood supply. PDAs can be visualized using a gadolinium-enhanced 3D MRA or ECG and respiratory navigator-gated 3D bSSFP imaging of the thorax. Cine CMR is also useful in detecting PDAs, particularly those that are small with turbulent flow. As with VSD jets, a longer TE will allow more time for spin dephasing and make the turbulent flow more conspicuous. Blood flow measurements are useful to quantify the Qp/Qs ratio. Note that with a PDA and no other shunting lesions, systemic flow (Qs) is equal to the main pulmonary artery flow and pulmonary flow (Qp) is equal to ascending aorta flow. It is also helpful to measure ventricular volumes and function, and assess RV systolic pressure by evaluating ventricular septal position in systole.
The goals of the CMR examination include (1) delineating the PDA size, (2) measuring the Qp:Qs ratio, (3) assessing the direction of PDA flow, (4) estimating the RV pressure by evaluating the interventricular septal configuration, and (5) calculating biventricular volumes, mass, and EF. These goals can be achieved by the following protocol:
- •
Cine bSSFP: stack of contiguous thin slices in an oblique sagittal plane positioned between the transverse aortic arch and the left pulmonary artery for PDA visualization (see Fig. 40.8 ).
- •
Velocity-encoded cine: in the same location as above, with velocity encoding in the direction of PDA flow to visualize the defect and direction of flow (see Fig. 40.8 ).
- •
Contrast-enhanced 3D MRA and/or ECG and respiratory navigator-gated 3D bSSFP imaging of the thorax.
- •
Cine bSSFP: ventricular short-axis stack from base-to-apex for quantitative assessment of biventricular volumes and EF.
- •
Blood flow measurements: ascending aorta (Qp) and main pulmonary artery (Qs); optional, superior vena cava and descending aorta at the diaphragm (Qs), and left and right pulmonary arteries distal to the PDA (Qp).
Partially Anomalous Pulmonary Venous Connection
In partially anomalous pulmonary venous connection (PAPVC), one or more but not all of the pulmonary veins connect to a systemic vein. PAPVC may be seen in isolation or as a component in complex congenital heart lesions, particularly heterotaxy syndrome with polysplenia. Common anomalous pulmonary vein connection sites are the left innominate vein, the right superior vena cava, the azygous vein, and the inferior vena cava. Anomalous connection of some or all of the right pulmonary veins to the inferior vena cava is termed “scimitar syndrome” ( Fig. 40.9 ). The name is derived from the curvilinear shadow in the right lung on chest radiography caused by the anomalous vein as it descends toward the right hemidiaphragm, which resembles a scimitar, or Turkish sword. Other abnormalities commonly seen in scimitar syndrome include hypoplasia of the right lung and pulmonary artery, secondary dextrocardia, and anomalous systemic arterial collateral vessels, usually from the descending aorta to the right lung.
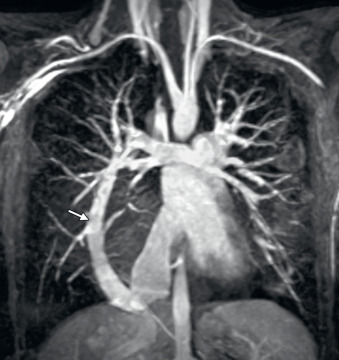
PAPVC results in a left-to-right shunt because blood draining from the lungs returns to the lungs via the systemic veins and right heart without passing through the systemic arterial circulation. This physiology leads to increased pulmonary blood flow and resembles that of an ASD. The magnitude of the shunt is determined by the number and size of the involved veins, the site of their connections, the pulmonary vascular resistance, the size of their vascular bed, and the presence of associated defects. Dilation is commonly seen in the systemic veins downstream of the anomalous pulmonary vein insertion site, the RA and RV, and the pulmonary arteries. Young patients are usually asymptomatic, but dyspnea on exertion becomes increasingly common in the third and fourth decades of life. Development of pulmonary hypertension is very rare. Patients may come to attention after auscultation of a pulmonary flow murmur or when diagnostic imaging is performed for another indication. Evidence of RV volume overload with no apparent intracardiac shunt should prompt a search for PAPVC. The presentation of scimitar syndrome varies widely depending on the severity of the associated abnormalities. Infants may be critically ill with respiratory compromise, whereas adults may have minimal symptoms.
For the most part, PAPVC can be surgically corrected, but the likelihood of success and probable benefits must be weighed carefully. A single small anomalous pulmonary vein is associated with a modest left-to-right shunt and does not require intervention. In those with much or all of the left pulmonary veins returning to the left innominate vein, the connecting vertical vein is usually large and long enough to detach from the innominate vein and anastomose to the LA. For veins connecting to the superior vena cava, a baffle within the superior vena cava and across the atrial septum can be constructed to channel the pulmonary venous return to the LA similar to sinus venosus defect repair. Alternatively, the superior vena cava can be transected superior to the anomalous veins and the caval end anastomosed to the right atrial appendage (Warden procedure). A baffle across the atrial septum is then created to direct the pulmonary venous flow in the cardiac end of the superior vena cava to the LA. Pulmonary veins entering the inferior vena cava can either be baffled through the RA to the LA or directly anastomosed to the LA. The most common postoperative complications seen in patients with PAPVC correction are obstruction and residual defects in the surgically created pathways.
Cardiovascular Magnetic Resonance
Preoperative
The acoustic properties of lung tissue may make it difficult by TTE to trace anomalous veins back into the lungs to confirm that they are pulmonary rather than systemic veins. If a clinical concern for PAPVC cannot be resolved by TTE with confidence, CMR is usually the most appropriate diagnostic imaging test. Several studies have demonstrated that CMR angiography techniques for PAPVC have a high level of agreement with surgical inspection and invasive x-ray angiography, and are more accurate than TTE and transesophageal echocardiography. In addition to defining anatomy, the size of the shunt can be calculated directly by measuring blood flow in the main pulmonary artery (Qp) and the ascending aorta (Qs). In some cases, it may also be possible to measure the flow in the anomalously draining vein itself. In the absence of significant valvular insufficiency, the ventricular stoke volume differential should be equal to the shunt size and thus serves as a useful check. For cases in which there is a hypoplastic pulmonary artery or pulmonary venous pathway obstruction, it is also important to measure blood flow in the branch pulmonary arteries to assess differential flow.
The goals of the CMR examination include (1) delineating pulmonary venous drainage, (2) measuring the Qp:Qs ratio and pulmonary artery flow distribution, (3) estimating the RV systolic pressure by evaluating the interventricular septal configuration, and (4) calculating biventricular volumes, mass, and EF. These goals can be achieved by the following protocol:
- •
Cine bSSFP: axial stack from the mid liver to the top of the aortic arch for pulmonary venous and arterial anatomy
- •
Cine bSSFP: ventricular long-axis planes and a short-axis stack from base-to-apex for quantitative assessment of biventricular volumes and EF
- •
Contrast-enhanced 3D MRA and/or ECG and respiratory navigator-gated 3D bSSFP imaging of the thorax
- •
Blood flow measurements: ascending aorta (Qs) and main pulmonary artery (Qp); left and right pulmonary arteries (Qp) and anomalous vein
Postoperative
The goals of a postoperative assessment include those of the preoperative assessment along with the need to carefully assess for obstruction and defects along the surgically created pulmonary venous drainage pathway. All of these aims can be accomplished using the preoperative imaging protocol above and tailored cine bSSFP imaging planes to visualize the pulmonary venous connection to the LA.
Coarctation of the Aorta
Coarctation of the aorta is a discrete narrowing most commonly located just distal to the left subclavian artery, at the ductus arteriosus insertion site ( Fig. 40.10 ). Hypoplasia and elongation of the distal transverse arch is a frequent association. Coarctation may be present alone or in combination with other heart lesions, including bicuspid aortic valve, subvalvar and valvar aortic stenosis, mitral valve abnormalities, ASD, VSD, persistent PDA, and conotruncal anomalies.
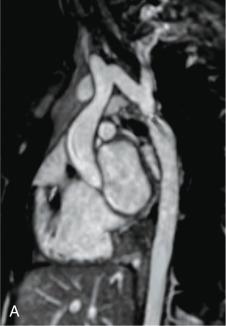
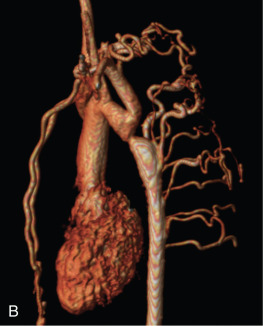
Infants tend to present with symptoms of heart failure and systemic hypoperfusion as the ductus arteriosus closes; if untreated, they may progress to shock or death. Older children and adults typically have isolated coarctation and may have minimal symptoms. Even in asymptomatic patients, relief of the aortic obstruction is indicated for hemodynamically significant lesions because of the high rate of late complications, including heart failure, systemic hypertension, premature coronary artery disease, ruptured aortic or cerebral aneurysms, stroke, aortic dissection, infective endarteritis, and premature death.
Therapeutic options for coarctation include surgical repair and percutaneous balloon angioplasty and stent placement. Currently, resection of the coarctation with an end-to-end anastomosis and augmentation of the transverse arch, if needed, is the most widely practiced surgical repair and has the lowest incidence of recurrent obstruction. Other approaches have included subclavian flap aortoplasty, patch augmentation, and conduit interposition. Coarctation in infants is treated surgically in the majority of centers because of the lower risk of residual obstruction, recurrence, and technique-related complications compared with percutaneous interventions. In the event of recurrent coarctation following surgical repair, balloon angioplasty with or without stent placement is often the preferred approach. Coarctation in older children or adults is increasingly being treated primarily by percutaneous interventions. Regardless of the initial treatment, subsequent surveillance for restenosis, aneurysm formation, and dissection is warranted.
Cardiovascular Magnetic Resonance
Preintervention and Postintervention
TTE is usually the only diagnostic imaging needed for evaluation of young children with suspected coarctation or following intervention for coarctation. With increasing age, acoustic windows typically deteriorate, leading to an incomplete assessment by TTE. In these circumstances, CMR is able to provide high-quality anatomic imaging of the aortic arch in its entirety ( Fig. 40.10 ), an assessment of the hemodynamic severity of the obstruction, and an evaluation of LV mass, volumes, and function. In a retrospective study of 84 adult patients following intervention for coarctation of the aorta, Therrien et al. showed that the combination of clinical assessment and CMR on every patient was more “cost-effective” for detecting complications than combinations that relied on TTE or chest radiography as imaging modalities. Other studies have shown the utility of CMR in infants and children with coarctation and other anomalies of the aortic arch. Computed tomography (CT) can also provide excellent anatomic imaging of the aorta but has the disadvantage of ionizing radiation exposure and the need for iodinated contrast make it a less attractive modality for serial follow-up.
With regard to aortic anatomy, careful attention should be given to the transverse aortic arch and isthmus, brachiocephalic vessels, collateral vessels that may bypass the obstruction, and possible aneurysms or dissections at the repair sites. Regions of vessel narrowing should ideally be measured in cross section as elliptical lesions are common. If a coarctation is present, its diameter, length, and distance to neighboring vessels should be reported, as this may influence decisions regarding percutaneous intervention. Given the association of a bicuspid aortic valve with coarctation, the aortic valve morphology should be noted as well as the dimensions of the aortic root and ascending aorta, as they may be dilated.
Blood velocity and flow measurements with CMR have been used to gain insight into the functional significance of a coarctation. One approach has been to assess the flow pattern in the descending aorta distal to the coarctation, preferably at the level of the diaphragm (see Fig. 40.11 ). Characteristics suggestive of a hemodynamically significant coarctation include decreased peak flow, decreased time-averaged flow, delayed onset of descending aorta flow compared with the onset of flow in the ascending aorta, decreased acceleration rate, and prolonged deceleration with increased antegrade diastolic flow. Another approach to assessing coarctation severity is to measure the peak coarctation jet velocity and estimate a pressure gradient using the modified Bernoulli equation. Note, however, that such measurements may be technically difficult in a long, tortuous coarctation segment and that the pressure estimates may not be indicative of anatomic severity because of collateral flow. Finally, CMR has been used to quantify collateral flow entering the descending aorta distal to the obstruction via retrograde flow from the intercostal arteries or vessels arising off the aortic arch and arch branches. With this technique, proximal flow is measured just proximal or distal to the site of obstruction, and distal flow in the descending aorta at the level of the diaphragm. A greater distal-to-proximal flow ratio is seen with increased collateral flow and suggests more severe obstruction. If little collateral flow is found, the surgeon may elect to perform a left heart bypass to the descending aorta during the repair to reduce the risk of spinal cord ischemic injury when correction requires interruption of aortic flow.
