Fig. 1
Biocontinuum of adverse and late effects of the stomach (with permissions from Rubin and Casarett 1968)
1.2 Anatomy and Histology
1.2.1 Anatomy
The stomach is a J-shaped, large, distensible portion of the digestive tract between the esophagus and small intestine, usually located in the left upper quadrant, where it occupies parts of the epigastric, umbilical, and left hypochondriac region (Fig. 2). It can expand considerably and can hold 2–3 L of food and/or liquid. The stomach can be divided into five parts: cardia, fundus, body, pyloric part, and pylorus. The cardia is the region around the cardiac orifice, where the esophagus enters the stomach. The fundus is the most superior portion of the stomach, often related to the left dome of the diaphragm and containing a bubble of gas seen on radiographs. The body is the major portion of the stomach between the fundus and the pyloric antrum. The pyloric part of the stomach consists of a wider portion, the pyloric antrum, that leads to a narrow portion, the pyloric canal, that ends at the final portion of the stomach, the pylorus. The pylorus serves as the distal sphincter that controls the discharge of stomach contents into the duodenum.
The stomach is covered entirely by peritoneum except where the blood vessels run along its curvatures and at a small bare area posterior to the cardiac orifice where the fundus of the stomach is in contact with the diaphragm. The stomach “bed” is formed by the posterior wall of the omental bursa and the retroperitoneal structures (pancreas and left kidney) between it and the posterior abdominal wall. Superiorly, the bed is bounded by the diaphragm, the spleen, and the left suprarenal gland. Inferiorly, it is bounded by the body and tail of the pancreas and the transverse colon.
1.2.2 Histology
The general histologic structure of the stomach is maintained throughout its length, with unique components within each substructure accounting for the differences. The basic structure is as follows (in order from outer to innermost layer): mucosa (epithelium, lamina propria, muscularis mucosa), submucosa, muscularis externa, and serosa.
The first layer of mucosa consists of epithelium, the lamina propria underneath, and a layer of smooth muscle, the muscularis mucosa. Underneath the mucosa lies the submucosa, a layer of fibrous connective tissue separating the mucosa from the muscularis externa. The Meissner’s plexus is in this layer.
The muscularis externa in the stomach differs from that of other GI organs, as it has three layers of smooth muscle instead of two. The inner oblique layer, a layer not seen in other parts of the digestive system, is responsible for creating the motion that churns and physically breaks down the food. The middle layer is composed of a thick circular muscular wall concentric to the longitudinal axis of the stomach. This is thicker at the pylorus, forming the pyloric sphincter, the portion of the stomach that controls the movement of food into the duodenum. The Auerbach’s plexus is found between the outer longitudinal layer and the middle circular layer. Finally, the serosa underneath the muscularis externa is made up of layers of connective tissue continuous with the peritoneum (Fig. 3a, b).
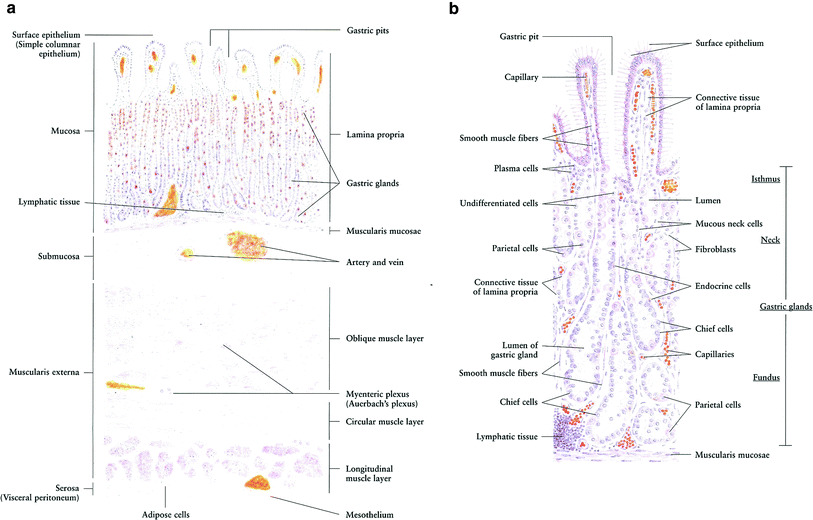
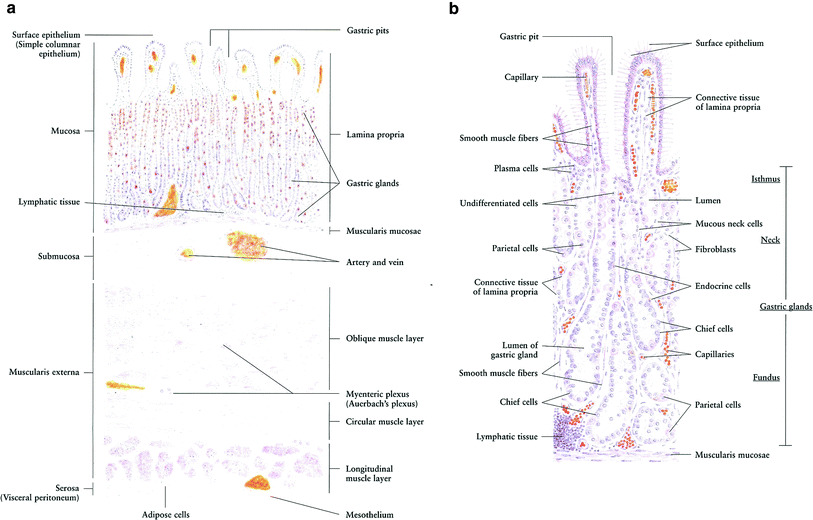
Fig. 3
Histology of a Stomach: low magnification. b Stomach: gastric glands, high magnification (with permissions from Zhang 1999)
1.3 Physiology and Biology
1.3.1 Physiology
The stomach functions by mixing the food stream with acid and other digestive secretions. These movements are coordinated by both intrinsic and extrinsic neural control, with the intrinsic myenteric plexi being the Meissner plexus (at the base of the submucosa) and Auerbach plexus (between the middle and outer muscle layers of the muscularis mucosa). The acid and digestive secretions are secreted from the mucosa.
The gastric mucosal surface is primarily composed of a simple layer of columnar epithelial cells 20–40 mm in height that secrete mucus, which provides luminal protection from acid, pepsin, ingested substances, and pathogens. The surface epithelial lining is invaginated by gastric pits, or foveolae, in which the gastric glands reside and which provides the glands access to the gastric lumen. The gastric glands of different anatomic regions of the stomach are lined with different types of specialized epithelial cells. The first region, the cardia, has glands populated by mucous, endocrine, and undifferentiated cells. There is a gradual transition from cardiac glands to the second region (fundus and body), which predominantly has parietal (oxyntic or fundic) glands. Parietal, chief (also known as peptic), endocrine, mucous neck, and undifferentiated cells compose the parietal glands. The antrum and pylorus contains pyloric glands, composed of endocrine cells, including gastrin-producing G cells and mucous cells.
The parietal glands are responsible for the secretion of acid, intrinsic factor (IF), and most gastric enzymes. A typical gland is subdivided into three areas: the isthmus (where surface mucous cells predominate), the neck (where parietal and mucous neck cells predominate), and the base (where chief cells predominate, along with some parietal and mucous neck cells). The principal cell type of the parietal gland is the parietal cell, responsible for the parietal mucosal secretion of 3 × 106 hydrogen ions/second, at a final HCl concentration of around 150 mmol/L. Acid secretion begins within 5–10 min of stimulation. Additionally, parietal cells are the site of intrinsic factor secretion via membrane-associated vesicle transport. Endocrine cells, somatostatin-containing and secreting D cells, and histamine-secreting enterochromaffin-like (ECL) cells are scattered throughout the parietal epithelium.
Closely associated with parietal cells are mucous neck cells, which appear singly, close to parietal cells or in groups of two or three in the parietal gland neck or isthmus. Mucous neck cells differ from their surface counterparts in their synthesis of acidic, sulfated mucus rather than the neutral mucus. Function of the two cell types appears different in that surface mucous cells are cytoprotective, whereas the mucous neck cell functions as a stem cell precursor for surface mucous, parietal, chief, and endocrine cells.
Chief cells, also known as zymogen cells, predominate in deeper layers of the parietal glands. These pyramid-shaped cells play a role in synthesis and secretion of pepsinogens I and II. Once secreted into the gastric lumen, pepsinogens are converted to pepsin and aid in digestion.
The final region of the stomach encompasses the antrum and pylorus and contains antral glands composed of endocrine and epithelial cells. The epithelial cells are predominantly mucous cells, and there are small numbers of pepsinogen II-secreting parietal cells. Although small in number, gastrin-secreting (G) cells play a vital physiologic role, as gastrin release is stimulated by gastric distention, vagal stimulation, dietary amino acids, and peptide, with rapid appearance of the hormone into the bloodstream in the postprandial period.
The function of the GI tract is controlled by both neural and hormonal mechanisms. Five major GI hormones have been identified. In addition, there are a number of other hormones, produced by neuroendocrine cells scattered through the mucosa of the stomach and small intestines, which play an important role in regulating and coordinating GI tract function. Once the bolus of food is propelled into the stomach, it is the stimulus to secrete HCl, pepsinogen, and pepsin, to begin the digestive process. In addition to the stimulus of secretions, the vagus nerve stimulates motility and as the food enters the pylorus, gastrin is stimulated and the bolus is further propelled into the duodenum. This stimulates the pancreas, which then secretes enzymes further the digestive process of the food (See Fig. 10 and Table 1).
1.3.2 Biology
1.3.2.1 Mucositis
The molecular biologic mechanisms that induce proinflammatory cytokines, chemokines, and profibrotic cytokines, are illustrated in Fig. 4. Involvement of the intestinal immune system and microvascular endothelium in the regulation of acute radiation mucositis and subsequent adverse tissue remodeling (intestinal fibrosis). When the mucosal barrier becomes disrupted, as after radiation exposure, bacterial products, and other activating agents gain access to subepithelial intestinal tissue where they stimulate a variety of immune cells to produce cytokines and other proinflammatory and anti-inflammatory mediators. Moreover, radiation-induced endothelial dysfunction with loss of thromboresistance, resulting in thrombin formation, neutrophil recruitment and activation, and stimulation of mesenchyme cells is seen.
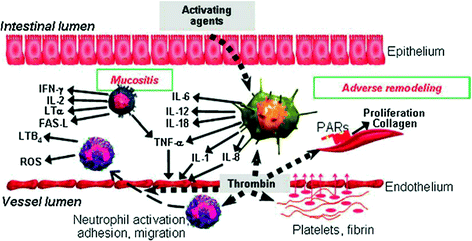
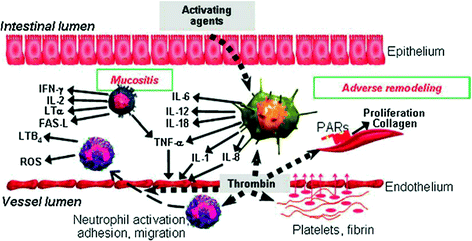
Fig. 4
Molecular biologic mechanisms associated with mucositis
1.3.2.2 Fibrosis
Recent studies have evaluated molecular mechanisms of radiation-induced fibrosis. Global gene expression profiles have shown induction of genes coding for multiple matrix components, matrix metalloproteinases, and tissue inhibitors of metalloproteinases, suggesting late radiation enteritis may be an active process of fibrogenesis and fibrolysis, with a balance toward fibrogenesis (Strup-Perrot et al. 2004). Ionizing radiation activates the translation of the gene coding for TGF-β (Herskind et al. 1998) and overexpression of transforming growth factor β1 (TGF-β1) has been associated with the development of fibrosis after radiation in the lung, liver, kidney, skin, and intestine. In the intestinal wall, TGF-β promotes fibrosis by stimulating collagen formation and the expression of fibronectin genes and the chemotaxis of fibroblasts and inhibits the degradation of the extracellular matrix. In a study of irradiated rat intestine, the TGF-β1 immunoreactivity score was significantly elevated in segments of bowel with intestinal fibrosis after radiation when compared to sham-irradiated segments (Hauer-Jensen et al. 1998). Interferon gamma (IFN-γ) inhibits the effects of TGF-β1 at the nucleus and has been proposed as a potential treatment for radiation enteritis (Nguyen et al. 2002).
1.3.2.3 Cell Kinetics Renewal Time
Cell kinetic turnover times of the gastrointestinal system determine the latent period before the clinical syndromes and manifestations occur. Thus, the acute effects will appear first in the small intestine, and then in the pylorus of the stomach, the body, in the first week of radiation. In the second week, the large intestine, first in the rectum, and then the colon reacts, as well as the cardia of the stomach and esophagus, which occurs simultaneously. It is noteworthy that the oral cavity and anus develop their mucositis in the first week (Table 2).
Table 2
Turnover times of epithelial cells of the alimentary tract of the rat (adapted from Rubin and Casarett 1968)
Cell population | Turnover time (days) |
---|---|
Digestive system | |
Lip | 14.7 |
Oral cavity | |
Buccal mucosa | 4.3 |
Tongue surface | |
Superior | 4.9 |
Inferior | 7.7 |
Esophagus | |
At thyroid gland level | 8.8 |
At cardiac junction | 11.6 |
Stomach | |
Cardia | 9.1 |
Body | |
Surface epithelium | 2.9 |
Gland | 6.4 |
Pylorus | |
Surface epithelium | 1.9 |
Gland | 1.8 |
Small intestine | |
Duodenum | 1.6 |
Jejunum | 1.3 |
Ileum | 1.4 |
Large Intestine | |
Colon | 10.0 |
Rectum | 6.2 |
Anus | 4.3 |
Cell renewal systems have been accurately estimated by Tritium Thymidine studies based on mitotic indices. On the basis of comparative values, it is apparent that the small intestine has the most rapid cell turnover of the various epithelial cells of the alimentary tract, and manifests the effects of irradiation earlier. From the view of cell kinetics, the time of appearance of the small intestine radiation injury compared to the stomach and to the oral mucosa is explained. The stem cells are in the Cripps of the Lieberkuhn, forming the proliferating or amplifying pool of cells. The differentiating cells lose proliferating capacity and move to the surface where they mature and ultimately, with extrusion, pass into the lumen (Vertalanffy and Lau 1962).
1.4 Pathophysiology of Radiation Damage
The damage to the stomach by radiation is best described in animal models. Breiter et al. (1989) demonstrated after irradiation with single doses, three distinct gastric disorders that occurred at different latency times. Acute death 2–3 weeks after irradiation was caused by an erosive and ulcerative gastritis and occurred in 17 % of the animals given 28.5 Gy with a protective, absorbable liquid diet, in 100 % of animals given 28.5 Gy without the protective liquid diet, and in 13 % of the animals given 23 Gy. Subacute to chronic fatal disorders that occurred 4 weeks to 7 months after irradiation included stomach dilatation and gastroparesis, associated with the replacement of the normal gastric mucosa by a hyperkeratinized multilayered squamous epithelium. These disorders occurred in 40–100 % of the animals after doses between 16 Gy and 28.5 Gy (+diet). Late gastric obstruction occurring at more than 7 months after irradiation resulted from profound changes in the gastric wall in 13–18 % of the animals after doses between 14 and 23 Gy. In animals surviving long term, an atrophic mucosa and intestinal metaplasia developed. It is unclear to what extent these findings in the rat, given in a single high-dose fraction, relate to humans. Friedman (1942) described acute gastric changes due to radiation in animal experiments. Initial early edema takes place shortly after irradiation, followed by degenerative changes (hyperemia, hemorrhage, and leukocytic infiltration) in the epithelial cells of the mucosa and in the stromal cells about 1 week after initiation. These changes can then progress to ulceration, with a peak at 1–2 months. Chen and Withers (1972) evaluated the characteristics of the stem cells of the gastric mucosa in mice after radiation and noted that the cells have a relatively large shoulder on the cell survival curve. Sublethal damage was about two-thirds complete after 1 hour. Regeneration was rapid, with surviving clonogenic cells doubling every 43 h. The relationship of these repair characteristics to that of the cells that produce late stomach injury remains unclear.
Goldgraber et al. (1954) described the results of a study of serial gastric biopsies after irradiation for peptic ulcer and defined the acute response of the mucosa to radiation. First, coagulation necrosis of the chief and parietal cells developed, then the tubules sloughed and were replaced by neck cells and a round cell infiltrate. Finally, at the peak of the reaction, there was loss of the glands associated with mucosal thinning, edematous interstitial tissue, and chronic inflammation. A decrease in gastric secretion occur secondary to irradiation. Radiation was used in the past to decrease acid production in patients with peptic ulcer disease (Rubin and Casarett 1968). This suppression persisted for a variable amount of time but is likely related to the total radiation dose. Examples of post-RT gastric ulcers are shown in Fig. 5a, b.
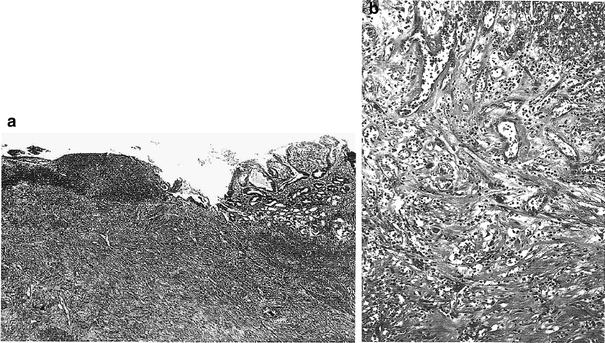
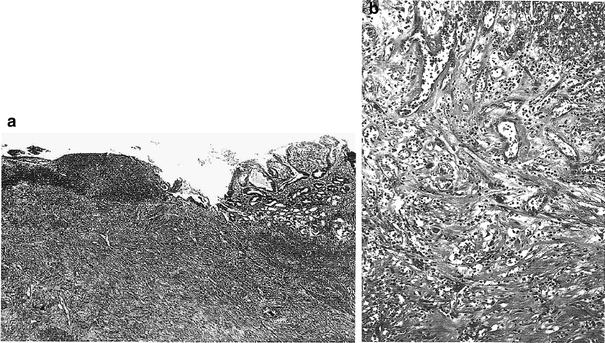
Fig. 5
a Chronic postradiation gastric ulcer. Beneath the necrotic ulcer base there is active chronic and atypical granulated tissue with enlarged vascular channels containing prominent and atypical endothelial cells. The gastric mucosa at the ulcer margin shows mild atypia of the glands. b This photomicrograph shows exuberant granulation tissue beneath the necrotic base of postradiation ulcer. Note the bizarre vascular channels with large, hyperchromatic endothelial cells (with permission from Fajardo et al. 2001)
1.5 Clinical Syndromes (Endpoints)
The clinical syndromes are described in LENT SOMA (Table 3) and CTC V4.0. The endpoints can be similarly segregated based on their focal versus global and clinical versus subclinical nature (Tables 4, 5). The nonacute clinical syndromes resulting from gastric irradiation have been described by Sell and Jensen (1966) consists of four types:
Dyspepsia: arising 6 months to 4 years after irradiation as vague gastric symptoms without clinical or radiographic signs.
Gastritis: arising 1–2 months from the completion of radiation and accompanied by radiological evidence of spasm or stenosis of the antrum. Gastroscopy reveals smoothened mucosal folds and mucosal atrophy, the pathologic basis being fibrosis of the submucosal tissue.
Acute ulceration: occurring shortly after the completion of the radiation, but rarely perforates.
Late ulceration: arising typically at 5 months after irradiation. The ulcer is indistinguishable from an ordinary ulcer. It can heal spontaneously, but can be accompanied by submucosal fibrosis, which can produce antral fibrosis.
Table 3
Clinical syndromes: gastric endpoints as described by the LENT SOMA system
Grade 1 | Grade 2 | Grade 3 | Grade 4 | |
---|---|---|---|---|
Subjective | ||||
Epigastric distress | Occasional and minimal | Intermittent and tolerable | Persistent and Intense | Refractory and excruciating |
Emesis | Occasional | Intermittent | Persistent | Refractory |
Pain | Occasional and minimal | Intermittent and tolerable | Persistent and Intense | Refractory and excruciating |
Objective | ||||
Hematemesis | Occasional | Intermittent | Persistent | Refractory |
Weight loss from time of treatment | ≥5–10 % | >10–20 % | >20–30 % | >30 % |
Melena | Occult/occasional normal hemoglobin | Intermittent <10 % decrease in hemoglobin | Persistent 10–20 % decrease in hemoglobin | Refractory or frank blood >20 % decrease in hemoglobin |
Ulceration | Superficial, ≤1 cm2 | Superficial,>1 cm2 | Deep ulcer | Perforation, fistulae |
Stricture (antro-pyloric region) | >2/3 normal diameter | 1/3–2/3 normal diameter | <1/3 normal diameter | Complete obstruction |
Management | ||||
Epigastric distress Emesis | Diet modification antacids | Intermittent prescription medication | Persistent medical management | Surgical intervention |
Pain | Occasional non-narcotic | Regular non-narcotic | Regular narcotic | Surgical intervention |
Bleeding | Iron therapy | Occasional transfusion | Frequent transfusions | Embolization, Coagulation or surgical intervention |
Ulceration | Medical intervention | Surgical intervention | ||
Stricture | Medical intervention | Surgical intervention | ||
Analytic | ||||
Barium radiography | Assessment of lumen and peristalsis | |||
Endoscopy | Assessment of lumen and mucosal surface | |||
CT | Assessment of wall thickness, sinus and fistula formation | |||
MRI | Assessment of wall thickness, sinus and fistula formation |
Table 4
Representative endpoints for gastric effects: segregated based on their global versus focal nature, and whether they are subclincal versus clinical
Stomach | ||
---|---|---|
Focal | Global | |
Subclinical | Atrophy of mucosa Asymptomatic ulceration Localized reduced compliance | Malabsorption Asymptomatic reduced capacity |
Clinical | Bleeding, ulceration | Bleeding, anemia Weight loss Early satiety |
Table 5
Benign versus malignant gastric ulcers: differential radiologic features
Features | Benign | Malignant |
---|---|---|
Hampton’s line | Yes | No |
Carman-Kirklin complex | No | Yes |
Crescent sign | Yes | No |
Project beyond gastric wall | Yes | |
Convergence of folds | To edge of crater | Stop short of crater |
Radiating folds thickened, irregular | Smooth | Club-shaped |
Ulcer shape | Linear, round, oval | Irregular |
Position of ulcer mound | Central | Eccentric |
Depth | Considerable depth in relation to size | Shallow in relation to overall size |
at mucosal surface | ||
Ulcer collar | Smooth, symmetrical | Eccentric |
Margin | Smooth | Nodular, irregular |
Peristalsis | Preserved | Diminished or absent |
Healing | Complete in 8 week | Very rare |
Multiplicity | 10–30 % | 20 % |
Associated duodenal ulcer | 50–60 % | Uncommon |
Location | Rarely in fundus or proximal greater curvature | Anywhere |
1.5.1 Detection: Endoscopy
As is true for esophageal cancer, the late effects endpoint scale is based on symptoms as well as intervention. The major interventional study that is useful for evaluating late effects in the stomach is an upper endoscopy. This allows thorough evaluation of gastritis and ulceration and can give worthwhile information regarding obstruction, although this is usually a secondary effect. Biopsies can also be obtained through endoscopy, which is very useful if there is concern regarding tumor recurrence. Upper GI radiography can also be useful for the same endpoints and is simpler and cheaper for the patient, but the result is often not definitive.
1.5.2 Diagnosis
The radiographic features of benign gastric ulcers (BGU) begin with excluding an associated mass. The characteristics of the BGU are (Fig. 6):
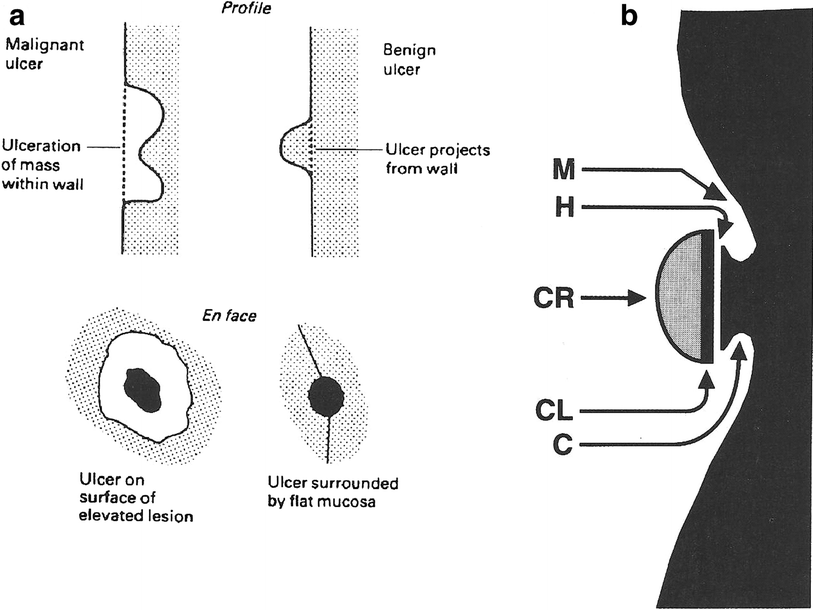
‘En face’ BGU are round or oval craters with surrounding gastric folds that may be enlarged secondary to edema.
In profile BGU are identified by Hampton’s line, an ulcer collar, and an ulcer mound, which separates it from the gastric lumen.
Benign ulcers tend to be located in the distal half of the greater curvature and respond to complete healing with antacid receptor antagonist.
Suspicion for malignant ulcer increases if the ulcer is located in the fundus and or proximal half of the greater curvature, especially if there is an associated mass.
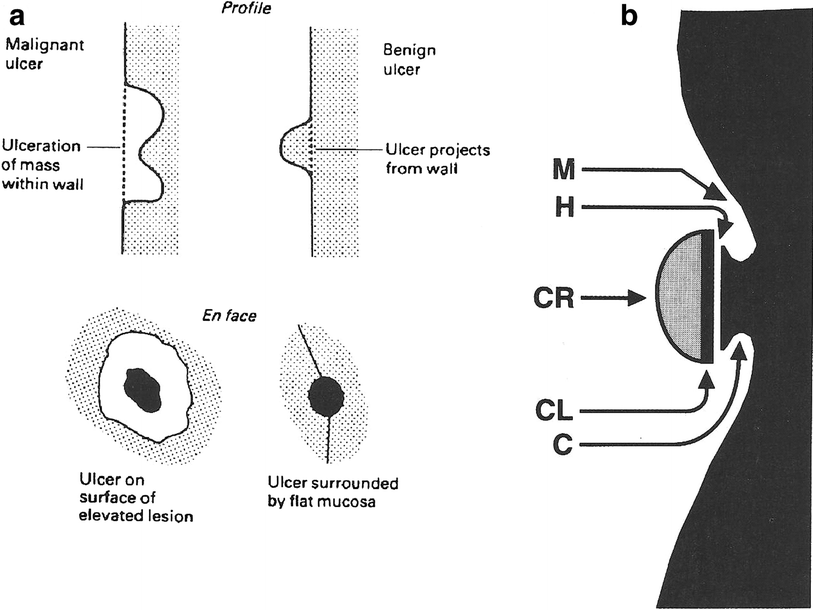
Fig. 6
Diagnosis. a Malignant versus benign gastric ulcers. Diferentiating radiologic features on double-contrast barium studies (from Bartram et al. 1981). b Profiles of a benign gastric ulcer. The crater (CR) is globular, sharp and distinct. The neck is sorrounded by a collar (C) that joins the crater to the lumen. The collar is parallel to the long axis of the stomach and does not project transversely into the lumen. A lucent or Hampton’s line (II) intervenes between the collar and crater and marks the mucosa at the entrance to the ulcer. Deep to Hampton’s line there may also be a parallel slitlike cleft (CL) that fills with barium. The ulcer is located in the center of a symmetrical mound (M) that projects into the lumen but merges gradually with the adjacent, normally distensible, gastric wall (with permission from Wolf 1973)
1.6 Radiation Tolerance
1.6.1 Dose-Time Fractionation
1.6.1.1 Acute Radiation-Induced Toxicity to the Stomach
Acute nausea due to radiation delivered to the stomach and small bowel are difficult to quantify and separate, as many studies that involve gastric and small bowel irradiation often are confounded by concurrent chemotherapy. A Japanese study of patients with diffuse large B cell lymphoma of the stomach treated with CHOP chemotherapy followed by 40.5 Gy to the stomach and regional nodes reported a 4 % (2/52 patients) incidence of grade 3 or greater acute nausea (Ishikura et al. 2005). No hemorrhage or perforation of the stomach was seen. In the GITSG study (Moertel et al. 1981) of locally unresectable pancreatic carcinoma treated with AP-PA fields, patients receiving radiation alone to 6,000 rads had a 36 % incidence of nausea (grade not specified). A review of single hemibody irradiation demonstrated a 83 % incidence of nausea and vomiting in patients treated to the upper abdomen (Danjoux et al. 1979). In this study, increased dose per fraction (≥600 rad vs. ≤500 rad) resulted in increased nausea and vomiting incidence (87 % vs. 58 %). The Italian Group for Antiemetic Research in Radiotherapy Observational Study (1999) demonstrated a 67 % incidence of nausea, 38 % incidence of vomiting, and a 71 % incidence of both nausea and vomiting in patients treated to the upper abdomen. In general, early effects of radiation (e.g., nausea, vomiting, and diarrhea) are dependent on dose per fraction and are likely to occur in the majority of patients treated with conventional doses of radiation to the stomach.
1.6.1.2 Late Radiation-Induced Toxicity to the Stomach
Cosset et al. (1988) reported late gastric complications (ulcer of stomach/duodenum, severe gastritis, obstruction) in two successive EORTC trials of radiotherapy for Hodgkin’s disease. This retrospective review of 516 patients, of whom 25 developed ulcers, two severe gastritis, six small bowel obstruction or perforation, and three with both, suggested complications were related to fractionation schedules and the use of laparotomy. The effect of total dose could not be evaluated because all patients received 40 Gy with only small variations. Patients with higher fraction sizes were significantly more likely to develop complications (4 % in weekly doses of 5 × 2 Gy, 9 % in 4 × 2.5 Gy, and 22 % in 3 × 3.3 Gy).
Goldstein et al. (1975) noted radiological abnormalities of the distal stomach in 8.3 % (10/121) of women who underwent 50 Gy of radiotherapy to the paraaortic nodes for metastatic cervix cancer. Five patients had prepyloric or pyloric ulcers, and the other 5 were located in the antropyloric region. These abnormalities occurred between 1 and 25 months after irradiation. Only two of these ten patients required surgical intervention. One of 52 men who received 40–50 Gy of paraaortic nodal irradiation for testicular tumors developed gastric outlet obstruction secondary to a pyloric ulcer 3 months after completion radiation. These data are similar to the toxicities observed in the EORTC Hodgkin’s trial.
Experience from Walter Reed Army Medical Center from the 1940s and 1950s in over 230 patients with testicular tumors treated primarily with 1 MV X-rays (but one-third were treated with 200 kVp) were reported (Amory and Brick 1951; Brick 1955; Hamilton 1948). There was substantial dose variation, but the majority of patients received between 4,000 and 6,000 r within 50–60 days. Though there were small numbers at the higher doses, there may be a suggestion for increased major complications of ulcer and perforation with increasing dose (see Tables 6 and 7).
Table 6
A radiation dose-response for major gastric complications following irradiation of testicular cancers
Dose (r) | Number of patients | Gastritis | Ulcer | Perforation |
---|---|---|---|---|
<3,000 | 82 | 0 | 2 (2 %) | 0 |
3,000–3,999 | 29 | 0 | 1 (3 %) | 0 |
4,000–4,999 | 50 | 10 (20 %) | 3 (6 %) | 3 (6 %) |
5,000 5,999 | 48 | 15 (31 %) | 8 (17 %) | 5 (10 %) |
>6,000 | 8 | 0 | 1 (13 %) | 3 (38 %) |
Table 7
Details of major complications (resulting in surgical intervention) in six patients following irradiation of testicular cancer
Dose (r) | Days to gastrectomy after irradiation | Reason for surgery | Operative findings |
---|---|---|---|
6,456 (32 days) | 95 | Hemorrhage | Perforated ulcer on postantrum |
5,880 (44 days) | 169 | Hemorrhage | Thickened wall |
Generalized petechiae | |||
5,304 (55 days) | 155 | Hemorrhage | Pyloric ulcer |
Petechial hemorrhage | |||
6,105 (49 days) | 190 | Pain | Perforated ulcer on postantrum |
5,096 (54 days) | 189 | Pain | Post pyloric ulcer |
4,800 (53 days) | 223 | Pain | Peritonitis from perforated antral ulcer |
The late gastric effects of radiation are summarized by Coia et al. (1995). It appears that for radiotherapy doses in the vicinity of 45 Gy, late effects (primarily ulceration) occur in approximately 5–7 % of patients. Specifically regarding gastric bleeds, Pan et al. (2003) reported on 12 gastric bleeds after radiation to 92 patients with liver tumor. Median time to bleeds was 3.5 months (range: 1.2–7.6 months). Average mean dose to the stomach was 14.06 Gy (range: 0.10–67.55 Gy). The LKB model was fit to the complication data for the stomach for the TD50 (1), “m,” and “n” LKB model parameters. For the stomach, the parameters for the stomach were 62, 0.30, and 0.07 Gy, respectively. Multivariate analysis demonstrated that in addition to NTCP, mean dose to stomach was significantly associated with GI bleed. This study confirmed that the stomach and duodenum do not have a large volume effect, and that dose thresholds exist for gastric bleeding risk.
1.6.2 Dose-Volume Factors
The exact volume of stomach irradiation is important to understanding symptoms and for the diagnosis of radiation-induced ulcer. First the location of ulcer within the radiation (PTV) corresponds to high doses delivered in treating neighboring tumor volumes. Second, if the fundus and body are spared or shielded, acid secretion can still occur and contribute to formation of radiation-induced ulcer. The pliability and ability of stomach to alter its volume is a challenge to accurately determine the radiation dose received to a specific region or volume of stomach.
1.7 Chemotherapy Tolerance
Chemotherapy alone does not appear to produce significant late GI complications with any frequency, despite the well-documented acute toxicity caused by a long list of agents (Table 8) (Mitchell 1992). Drugs, such as 5-fluorouracil (5-FU), produce diarrhea and, occasionally, small bowel toxicity (Fata et al. 1999). More severe late effects, such as GI bleeding, generally have been seen only in combined-modality therapy, which has led to severe acute complications, particularly in the small bowel (Minsky et al. 1995; Bosset et al. 1997).
Table 8
Chemotherapy: gastrointestinal chemotoxic agents and their late effects
Agent | Late effect |
---|---|
5-Fluorouracil | Enteritis |
5-Fluorouracil + mitomycin C (+ RT) | Late benign esophageal stricture |
5-Fluorouracil + MeCCNU(+ RT) | Fistulas, perforation |
Actinomycin D (+ RT) | Enteritis |
Bleomycin (+ RT) | Esophagitis |
Cisplatin (+ RT) | Enteritis |
Cyclophosphamide (+ RT) | Esophagitis |
Doxorubicin (+ RT) | Esophagitis |
Methotrexate | Enteritis |
Procarbazine | Enteritis |
The impact of chemotherapy on late tolerance of the stomach to irradiation has not been studied formally. There has been increasing utilization of combined modality therapy with radiation therapy and 5-FU-based chemotherapy in the treatment of pancreatic tumors where the stomach is of necessity in the radiation field. Although no formal comparisons have been done there is not an obvious increase in late gastric injury after doses in the range of 45–50 Gy to the pancreas in conjunction with concurrent 5-FU compared to similar doses given without chemotherapy. Other agents such as adriamycin and mitomycin C have been used in the treatment of pancreatic cancer without any obvious adverse effects. In many of these studies the chemotherapy was given after the completion of radiation therapy rather than concurrently. The data from Mohioddin et al. (1992) of patients treated to high radiation dose with chemotherapy has already been mentioned. It is not possible to ascribe the GI bleeding in this series to the use of chemotherapy and not to the relatively high radiation dose. All of these studies are difficult to analyze because of the short follow-up inherent in the treatment of patients with pancreatic cancer. However, the data that are available do not suggest a major increase in late gastric toxicity with the combined modality approach of radiation plus 5-FU compared to radiation alone.
1.7.1 Combined Modality
There is little information to support increased toxicity from chemoradiation therapy. In pancreatic cancers, the use of agents such as doxorubicin and mitomycin C in combination with radiation has been well tolerated (Coia et al. 1995). Since most radiation oncology protocols avoid doses higher than 45–50 Gy, gastric late effect toxicity is uncommon.
1.8 Special Topics
1.8.1 Surgery
There was a significant increase in complications in patients who underwent laparotomy prior to radiotherapy (2.7 % without and 11.5 % after laparotomy). The highest complication rate of 39 % was seen in patients given three weekly fractions of 3.3 Gy after laparotomy. There was no documentation on the volume of stomach irradiated.
1.8.2 Peptic Ulcer
Among the numerous benign disease entities treated by irradiation, peptic ulcer was managed prior to the introduction of medical inhibitors of gastric secretion. Relatively modest doses of radiation, i.e., 1,000–2,000 cGy fractionated in 10 days induced a prolonged suppression of the gastric HCl secretions by inhibiting parietal cells. A 20-year experience was documented by Palmer and Templeton. Approximately 50 % of patients treated with the higher doses of 1,500–2,000 cGy, had prolonged suppression of a year or more, allowing for peptic ulcers to heal. A small percent (15 % of patients) had prolonged depression of HCl secretion for 1–5 years (Palmer and Templeton 1939).
1.9 Prevention and Management
1.9.1 Prevention
Stomach sparing intensity-modulated radiation therapy (IMRT) is helpful in reducing the volume of the stomach being included in the treatment beam when irradiating surrounding structures involved with cancer, i.e., liver, spleen, and pancreas. Efforts at decreasing the risk of stomach complications from abdominal radiotherapy have been aimed at limiting the dose to the stomach while maintaining an adequate dose to the target. IMRT offers the means of limiting the radiation dose to the stomach by creating a more conformal dose distribution. This treatment approach has been introduced into treatment of pancreatic and hepatic malignancies (Cheng et al. 2003; Milano et al. 2004). However, long-term results regarding gastric toxicity is not yet available.
1.9.2 Management
For acute stomach toxicity (nausea and vomiting), decreasing the radiation dose per fraction and eating a light meal prior to radiation therapy can help minimize acute symptoms. Additionally, antacids, H2 blockers, proton pump inhibitors (PPI), and antiemetics are often used. Antiemetic regimens include 5HT3 inhibitors, phenothiazines, metoclopramide, corticosteroids, benzodiazepines, antihistamines, and anticholinergics. Prophylactic 5HT3 inhibitors have been shown in randomized trials to have efficacy compared to placebo in preventing radiation-induced nausea and vomiting (Franzen et al. 1996; Horiot and Aapro 2004). Acute radiation-induced nausea and vomiting typically appear within the first 24 h after treatment.
Patients with bleeding and ulceration require endoscopy. Argon laser coagulation has been used to temporarily control bleeding. Other local therapies include injections of epinephrine and vasoconstrictor agents. At times repeat endoscopy, and blood transfusions or eventually surgery are needed. For patients with intractable bleeding, perforation, fistulae, or obstruction, surgery may be indicated.
For severe complications of perforation, severe bleeding, or gastric outlet obstruction, the appropriate therapy is usually surgical. Generally it is necessary to do a partial gastrectomy to remove the effected area of the stomach. If there is no other significant radiation injury in the abdomen, gastrectomy is usually effective in resolving the morbidity.
1.10 Future Studies
In developing a system by which one can analyze complications to the gastrointestinal tract from therapeutic irradiation, it is important to keep in mind some of the information that is needed for a good analysis and to raise certain issues that it would be helpful to address:
1.
The relationship of radiation injury to dose per fraction and whether improved normal tissue sparing by the use of radiation doses of less than 1.8–2 Gy per fraction is a possibility.
2.
Find meaningful volume effect relating to radiation injury of the gastrointestinal tract. The development of 3D treatment planning and availability of dose-volume histograms will be important in this regard.
3.
Identify variable radiation sensitivity of different anatomical portions of the GI tract.
4.
Identify biological manipulations that could be used to decrease the likelihood of radiation-induced gastrointestinal injury.
5.
Assess radiation-induced gastrointestinal ulcerations healed satisfactorily with present day medical management. Assess the apparent decrease in incidence of this complication a reflection of more routine aggressive management of early ulcer disease with medical therapy.
6.
Identify the impact of the use of combined modality therapy with chemotherapy and radiation therapy on the incidence of gastrointestinal toxicity. This issue is especially important because at present a large number of patients being treated to the esophagus or abdomen for gastrointestinal malignancies receive combined modality therapy with, as a minimum, 5-fluorouracil containing chemotherapy.
7.
Evaluate radiation sensitivity of the stomach differ substantially from that of the small bowel, which is typically in the same radiation field. As most of the information on small bowel tolerance has come from pelvic irradiation, decide whether that information applies to the somewhat different anatomy of the upper abdomen. This also is relevant with the increased interest in using high-dose radiation therapy for upper abdominal malignancies.
8.
Identify the actual mechanism of the various types of radiation injury to the gastrointestinal tract. Virtually all of the data in this area is very old and most have not approached the issue of the mechanism of the injury, rather just an anatomical description of the injury.
9.
Choose the scale for early and late complications scoring both useful and reproducible. After establishment of a reliable and self-consistent scale for toxicity, studies can be accomplished that establish dose-volume relationships for complications. The most appropriate disease sites for these ancillary studies should be sites that have a strong track record of protocol accrual where intestinal injury can be a dose-limiting factor and where radiation techniques are fairly well standardized.
10.
Identify the most effective method for distinguishing late effects from tumor recurrence. Oftentimes, clinical recurrence cannot be readily distinguished from late effects. Standardized approaches and improved techniques in distinguishing these two competing factors must be developed. It is important additionally to report the time adjusted rate of complications at regular intervals.
It is the goal of the radiotherapist to achieve the highest degree of local regional control with acceptable and defined morbidity. In answering the above questions regarding radiation-related gastrointestinal morbidity, we equip ourselves to design treatment regimens that assist us in maximizing the therapeutic ratio. It is clear from this review that much remains to be determined regarding gastrointestinal morbidity of radiation, but the tools for toxicity measurement and potential means for management have been identified.
1.11 Literature Landmarks
Desjardins (1931): presented a general review of the depressive and injurious effects of irradiation reported up to that time.
Engelstad (1938): conducted careful and complete studies in the rabbit stomach after varying doses of irradiation. A solitary exposure of 1,500 R produced ulcers in 2–4 weeks.
Warren and Friedman (1942): described the pathology of intensive irradiation of stomach and other viscera.
Bowers and Brick (1947): presented the indications and techniques of surgery in radiation gastric ulcer.
Friedman (1952): described four types of stomach injury produced by high-dose radiation.
Goldgraber et al. (1954): Presented beautifully detailed serial studies of histologic changes following irradiation of the stomach.
Jennings and Arden (1960): conducted a serial histopathologic study of radiation changes in the esophagus of rats following single dose exposure to 3,000 R.
Rubin and Cassarett (1968): presented the bio-continuum paradigm to chart clinical pathophysiologic events in an early/late timeline.
Rubin (1995): presented the LENT-SOMA toxicity scales for radiation effects to evaluate the grade of severity.
Trotti and Rubin (2003): modified and developed the common toxicity criteria CTC V3.0 which applied similar scales to grade adverse effects of all major modalities—surgery and chemotherapy in addition to irradiation.
2 Small Intestine
2.1 Introduction
Chronic radiation injury of the intestine was first described shortly after the discovery of ionizing radiation. In 1895, Walsh concluded that radiation caused an “inflammation of the intestinal mucosa.” (Walsh 1897) Further reports in the early twentieth century first described stenosis and fistula development after pelvic radiation (Futh 1915) (Fig. 7).
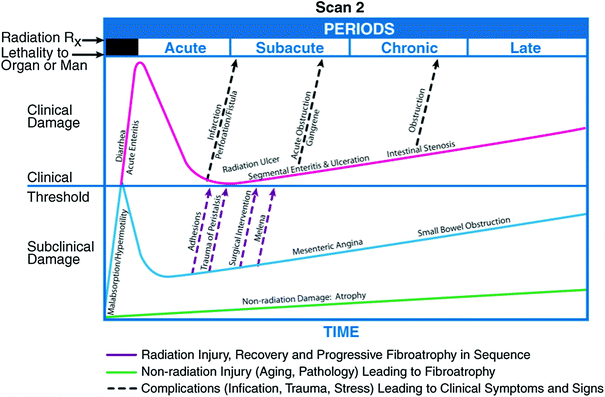
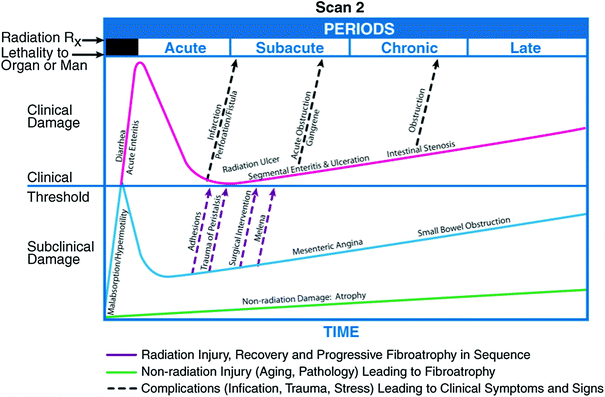
Fig. 7
Biocontinuum of adverse and late effects of the small intestine (with permission from Rubin and Casarett 1968)
The genesis of combined-modality treatments have led to increased risk of treatment-related toxicity (Phillips and Fu 1977). Balancing the risks and benefits of treatment has become increasingly difficult for the practicing oncologist. However, with improved survival seen in many malignancies, cognizance and avoidance of late treatment toxicities will become even more important to patients and their physicians. A thorough knowledge of the risk factors associated with late toxicity in a variety of clinical situations is paramount to optimization of the therapeutic ratio. The Biocontinuum of adverse acute and late effects are shown in Fig. 1.
2.2 Anatomy and Histology
2.2.1 Anatomy
The small intestine is a hollow tube approximately 2.5 cm in diameter and 6–7 m in length, coiled into tubes and filling the majority of the abdominal cavity (Fig. 8). It begins at the pyloric sphincter in the stomach and extends to the ileocecal valve where it makes its connection with the large intestine. It is comprised of three anatomically and histologically distinct subunits: the duodenum, jejunum, and ileum. The duodenum is the shortest portion of the small bowel and pursues a C-shaped course around the head of the pancreas. It is partly intraperitoneal and extraperitoneal, but is mostly fixed by peritoneum to structures on the posterior abdominal wall. It is divisible into four separate parts: superior, descending, horizontal, and ascending. The duodenum connects to the jejunum at the duodenojejunal flexure, supported by the ligament of Treitz. The jejunum comprises about two-fifths of the remaining small bowel length and lies primarily in the left upper quadrant of the abdomen. The ileum comprises the remainder of the small bowel and lies primarily in the right lower abdominal quadrant, where it terminates at the ileocecal valve. The jejunum and ileum are both intraperitoneal structures.
2.2.2 Histology
The mucosal pattern of the small intestine is basically the same with numerous finger-like villi, which have their core of connective tissue of the lamina propria covered by intestinal epithelium. The intestinal glands (crypts of Lieberkuhn) are housed in the lamina propria. The muscularis mucosae is formed of a layer of smooth muscle fibers. The entire coat of mucosa is thrown into plicae circulares (Fig. 9a).
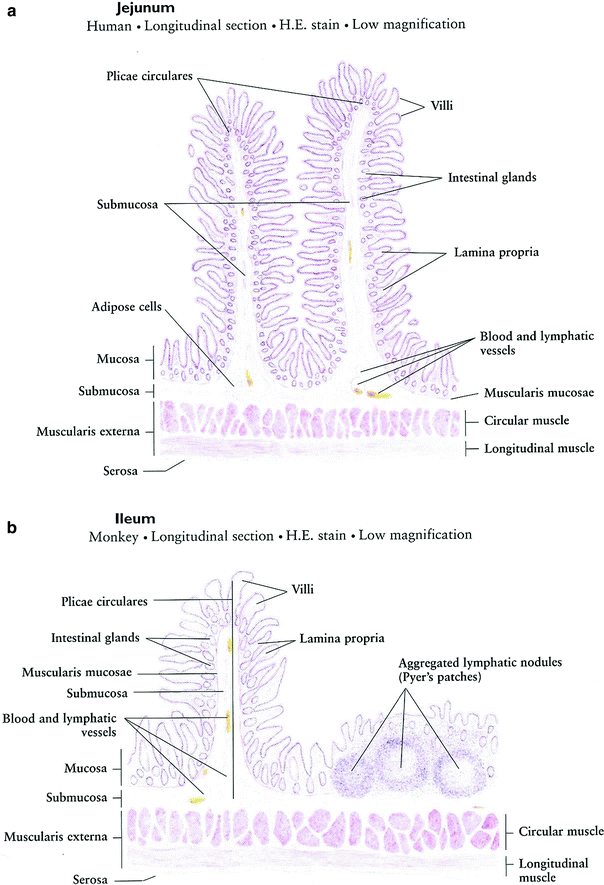
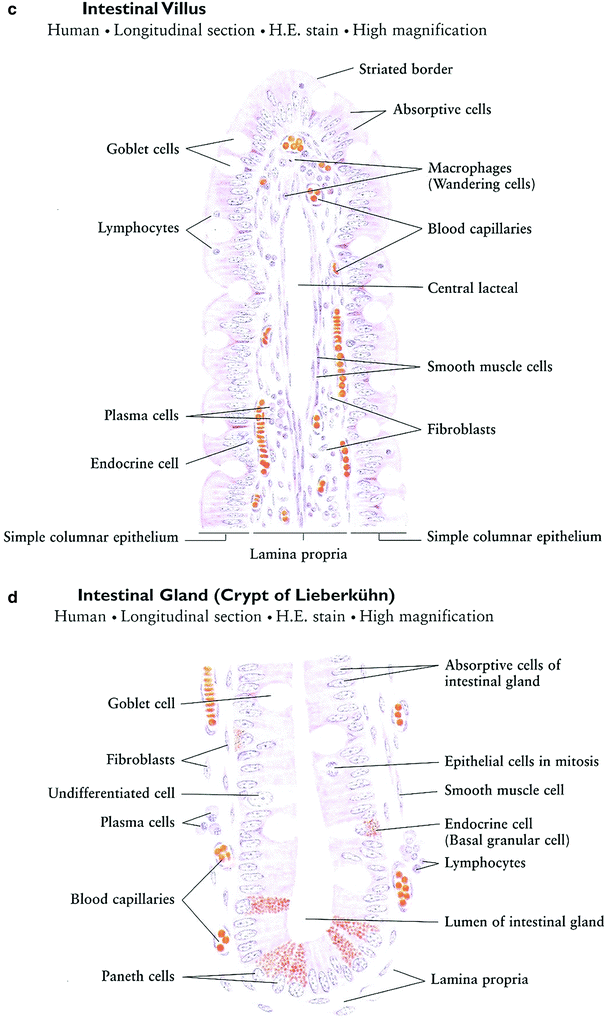
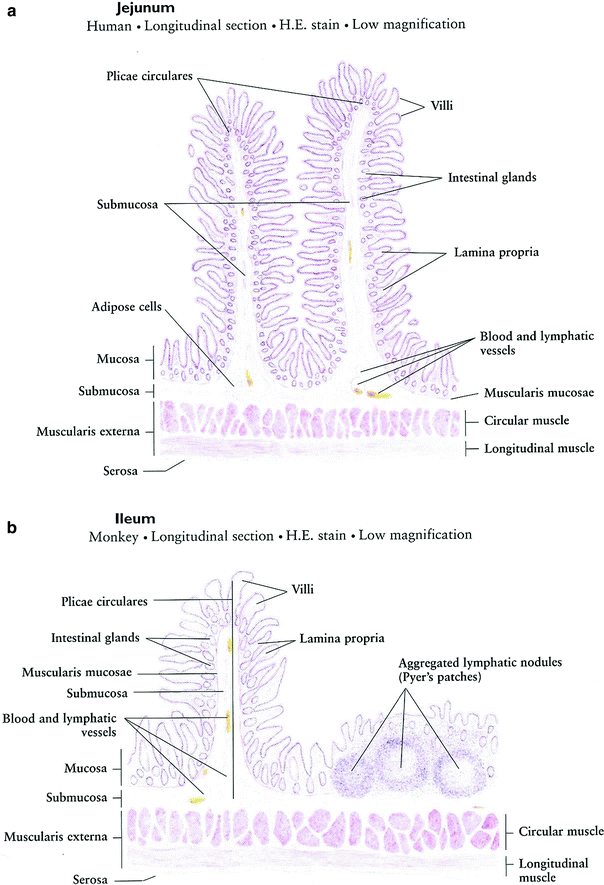
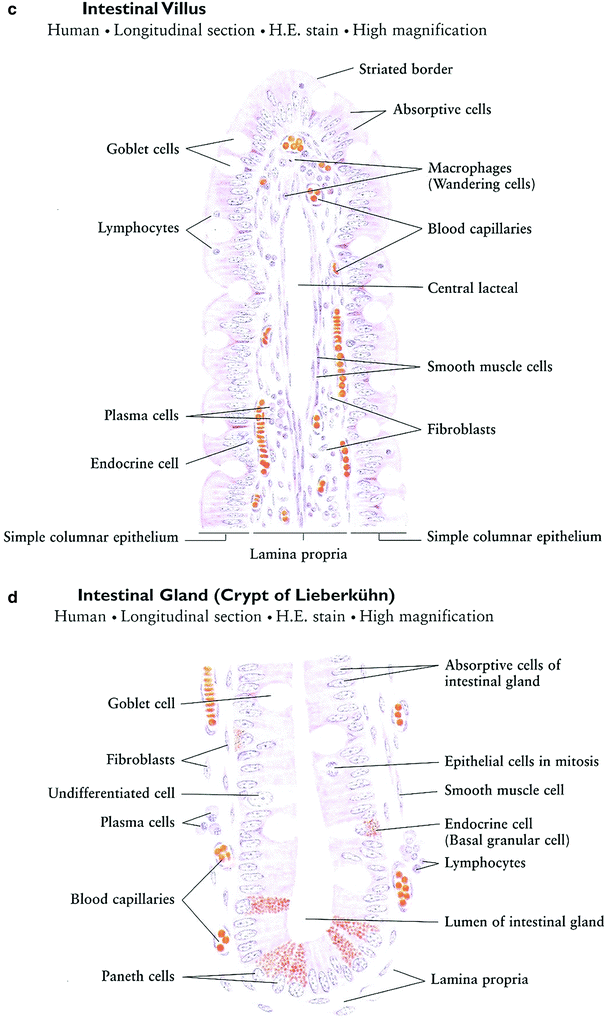
Fig. 9
Histology of the small intestine: a Jejunum. b Ileum. c Intestinal Villus. d Intestinal Gland (with permissions from Zhang 1999)
Intestinal villi are finger-like or leaflike projections of the mucosa, 0.5–1.5 mm in length, found only in the small intestine. They vary in form and length in different regions. Those of the duodenum are broad, spatulate structures, but they become finger-like in the ileum. Each has a core of lamina propria covered with simple columnar epithelium (Fig. 9b).
Intestinal glands (crypts of Leiberkuhn) are tubular structures within the lamina propria, 0.3–0.5 mm in depth, which open between the bases of the intestinal villi. They are lined with simple columnar epithelium composed of absorptive cells, goblet cells, endocrine cells, and undifferentiated cells (Fig. 9c, d).
The general histologic structure of the small intestine is maintained throughout its length: the duodenum, the jejunum, and the ileum, intestinal villi decrease in number; each villus has less villi and intestinal glands. The basic structure is as follows (in order from outer to innermost layer): mucosal epithelium, lamina propria, muscularis mucosa, submucosa, muscularis externa, and serosa. The serosa and muscularis externa are similar throughout the small intestine with the muscularis being characterized by both longitudinal and circular layers, with Auerbach’s myenteric plexus in between. The submucosa of the duodenum is distinct from the jejunum and ileum by the presence of Brunner’s glands and Meissner’s submucosal plexus, which function together to secrete an alkaline solution for combination with the acidic chyme from the stomach. The ileum is histologically distinct due to the presence of Peyer’s patches within the lamina propria, which are oval or round aggregations of lymphoid tissue. The mucosal lining throughout the small bowel is simple columnar, with longer villi in the jejunum and progressive villus shortening in the ileum.
2.3 Physiology and Biology
2.3.1 Physiology
The intestinal tract functions grossly through both anterograde and retrograde peristalsis, which serve to mix the food stream and promotes maximal contact of nutrients with the mucosa. These movements are coordinated by both intrinsic and extrinsic neural control, with the intrinsic myenteric plexi being the Meissner plexus (at the base of the submucosa) and Auerbach plexus (between the inner and outer muscle layers of the bowel wall). As the food stream progresses distally through the intestinal tract, nutrients are absorbed and the fecal matter is gradually condensed, with each subunit having a distinct role in the process. Although the shortest portion of the bowel, the duodenum is largely responsible for the breakdown of food in the bowel through mixture of secreted digestive enzymes from the gall bladder and pancreas, as well as coordination of the emptying of the stomach via hormonal pathways. The jejunum and ileum, with their large surface areas, serve primarily to transport nutrients across the epithelial cells. The ileum also plays a role in immune function through Peyer’s patches and is also the site where vitamin B12/intrinsic factor complexes are absorbed.
Enteroendocrine cells are specialized cells present in the mucosa of the digestive tract. They account for less than 1 % of all epithelial cells in the gastrointestinal tract, but as a whole, they collectively constitute the largest endocrine “organ” in the body. Most of these cells are not grouped as clusters in any specific part of the gastrointestinal tract. Rather, enteroendocrine cells are distributed singly throughout the gastrointestinal epithelium. For that reason, they are described as constituting part of a diffuse neuroendocrine system (DNES). Enteroendocrine cells secrete their products into either the lamina propria or underlying blood vessels. In general, two types of enteroendocrine cells can be distinguished throughout the GI tract. Enteroendocrine “closed cells” do not reach the lumen, whereas enteroendocrine “open cells” are exposed to the gland lumen (Fig. 10 and Table 1).
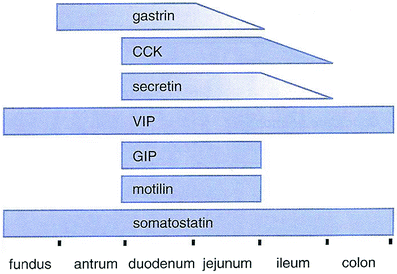
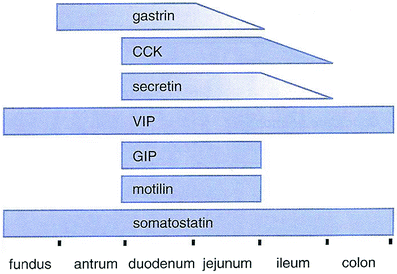
Fig. 10
Physiology. Gastrointestinal hormones. This schematic diagram shows the distribution of gastrointestinal peptide hormones produced by enteroendoorine cells in the alimentary canal.CCK,cholecystokinin; VIP, vasoactive intestinal peptide; GIP, gastric inhibitary peptide. (Modified from Johnson LR 1997)
2.3.2 Biology
2.3.2.1 Molecular Mechanisms
For the molecular mechanisms of the acute inflammatory mucositis and the late fibrosis stage, the proinflammatory cytokines and chemokines for the inflammatory mucositis and the profibrotic cytokines for the later fibrosis stage are described in Sect. 1.3.2 (Fig. 4).
2.3.2.2 Cell Kinetics Renewal Times
Cell kinetic turnover times of the gastrointestinal system determine the latent period before the clinical syndromes and manifestations occur. Thus, the acute effects will appear first in the small intestine, and then in the pylorus of the stomach, the body, in the first week of radiation. In the second week, the large intestine, first in the rectum, and then the colon reacts, as well as the cardia of the stomach and esophagus, which occurs simultaneously. It is noteworthy that the oral cavity and anus develop their mucositis in the first week (Table 2).
2.4 Pathophysiology
The majority of clinical symptoms, including abdominal pain, diarrhea, steatorrhea, and obstruction are related to decreased intestinal motility and malabsorption. Decreased motility is a direct result of functional impairment from collagen proliferation within the bowel wall. Malabsorption is a multifactorial process resulting from mucosal atrophy, vascular insufficiency, and decreased motility resulting in bacterial overgrowth. Increased fibrosis within the bowel wall and the surrounding connective tissues can lead to focal narrowing with resultant bowel obstruction.
The subacute to chronic radiation toxicity can be rapid in onset due to obstruction of small intestinal arteriole(s) resulting in bowel wall ulceration and necrosis. If progressive, it will result in perforation of the bowel wall and peritonitis (Fig. 11a, b).
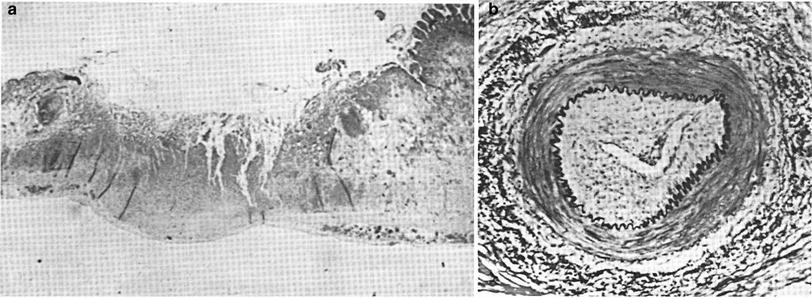
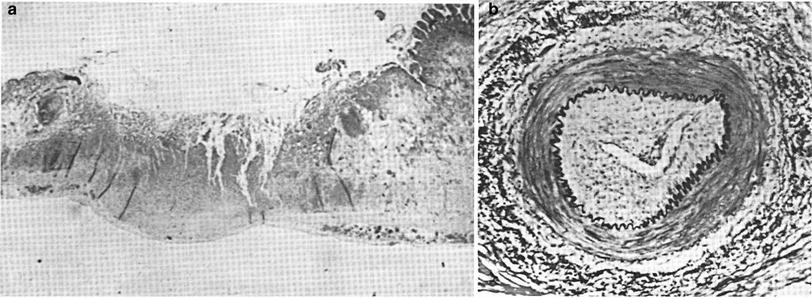
Fig. 11
a Pathology of subacute radiation ulcer of the small intestine. 6 months after radiation therapy (about 60 Gy; low mag). b Cross section of a small artery underlying the ulcer shown in a, showing obstruction of the lumen by marked endothelial proliferation (endarteritis obliterans) and other changes in the arterial wall (high mag) (from Rubin and Casarett 1968, with permission)
Late radiation toxicities are typically classified as those occurring more than 60–90 days after the completion of treatment. Late radiation toxicity of the small bowel can occur after a variable latency period, with the majority of symptoms occurring 6 months to 3 years after completion of treatment. However, patients are at risk of complication many years and even decades after treatment, and patients who recover from a first complication are at increased risk of further complications (Galland and Spencer 1985).
Gross examination of affected bowel reveals gray, roughened peritoneal surfaces with telangiectasias, fibrinous deposits and adhesions. The bowel wall and mesentery appear thickened and indurated and mucosal ulceration and luminal stenosis are common (Berthong and Fajardo 1981; Berthong 1986). Typical microscopic findings include mucosal atrophy, wall fibrosis, obliterative vascular sclerosis and lymphatic dilation. The majority of chronic radiation damage is seen in the submucosa, which is in contrast to acute injury which is most prominent in the mucosa. Stromal tissue within the bowel wall contains an abundance of atypical fibroblasts with collagen proliferation. These fibroblasts are characterized by large, irregular nuclei and cytoplasmic projections. Arterioles are characterized by a chronic vasculitis with hyalinized thickening of the vessel wall. Thickening of the vessel wall can lead to occlusion or necrosis within the wall with resultant rupture.
2.5 Clinical Syndromes (Endpoints)
The clinical syndromes are described in LENT SOMA (Table 9) and CTC V4.0. The endpoints can be similarly segregated into Global versus Focal and acute versus subacute (Table 10).
Table 9
Clinical syndromes: LENT SOMA system for categorizing and grading toxicities: small intestine/colon
Grade 1 | Grade 2 | Grade 3 | Grade 4 | |
---|---|---|---|---|
Subjective | ||||
Stool frequency | 2–4 per day | 5–8 per day | >8 per day | Refractory diarrhea |
Stool consistency | Bulky | Loose | Mucous, dark, watery | |
Pain | Occasional and minimal | Intermittent and tolerable | Persistent and intense | Refractory/rebound |