Fig. 1
Bio-continuum: Pancreatic acinar cells are known to be radioresistant to irradiation, even with larger doses and chemoradiation combo-based regiments, since modern radio techniques (IMRT, SERT) tend to spare a significant volume of this elongated organ, acute pancreatitis has not been reported clinically. Malabsorption has been reported but is transitory. With experimental radiation injury to the exocrine pancreas, islet of Langerhans appear to be spared, and although late diabetes has not been reported, it has been observed as a late effect in long-term survivors of childhood malignancies who have received abdominal irradiation. Oncogenesis with second malignant causes have been observed (with permission from Rubin and Casarett 1968)
2 Anatomy and Histology
2.1 Gross Anatomy
The pancreas arises embryogenically from endoderm. It is covered by a loose connective tissue capsule that penetrates the pancreas and separates the parenchyma into lobules. The pancreas is a long gland that lies transversely across the central posterior abdomen and extends from the duodenum to the splenic hilum. The organ is divided into three parts; the head with a small uncinate process, the body, and the tail. The head of the pancreas lies in the curve of the duodenum, while the body lies posterior to the transverse colon and greater curvature of the stomach. The pancreas lies anterior to the upper abdominal aorta, the first and second lumbar vertebral bodies, and the superior poles of the kidneys (Fig. 2).
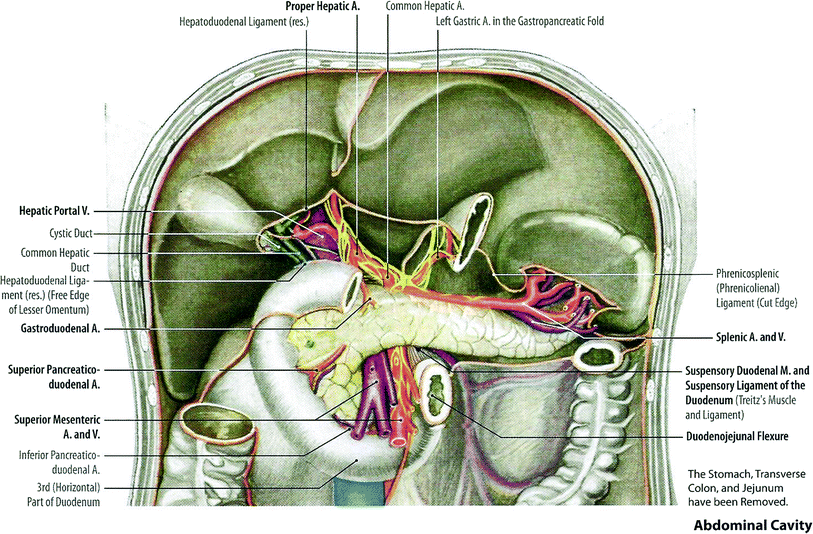
Fig. 2
Anatomy of pancreatic area (with permission from Tillman 2007)
Rich vascular and lymphatic networks surround the pancreas. Regional lymph node basins are associated with specific locations within the pancreas. The pancreatic head region drains primarily to the lymph nodes along the common bile duct, common hepatic artery, porta hepatis, anterior and posterior peri-pancreatic soft tissues, and the superior mesenteric chain. The pancreatic body and tail may drain to the common hepatic artery, celiac axis, splenic artery, and splenic hilum. The posterior pancreas also drains to the para-aortic lymph nodes.
2.2 Histology and the Functional Subunit
The exocrine component of the pancreas consists of closely packed secretory acini, which drains into a branched ductal system. Each acinus is made up of an irregular cluster of pyramidal secretory cells with apices projecting toward the lumen of a small central duct. The acinar cells are typically protein-secreting (zymogenic) cells with the apices packed with zymogen secretory granules, which secrete into the central duct in response to cystokinin, produced by enteroendocrine glands of the duodenum. In addition, a bicarbonate-rich fluid produced by terminal intercalated ducts located in the acinar cell walls is secreted into the central duct in response to secretin, also from the duodenal enteroendocrine glands. This secretion serves to neutralize acidic gastric chyme. (Fig. 3a, b).
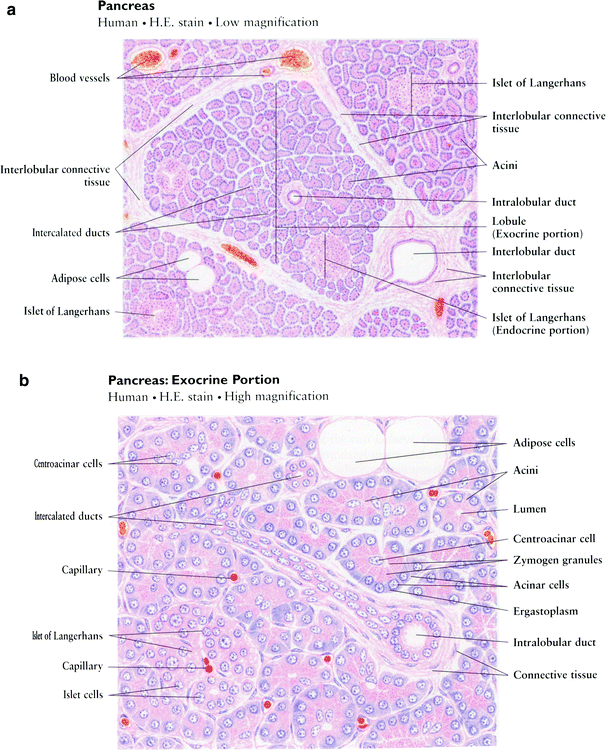
Fig. 3
Histology of pancreas. a Low magnification, b High magnification (with permission from Zhang 1999)
The small central ducts are lined with cuboidal epithelium, that becomes stratified as the ducts become progressively larger in size. The dense connective tissue that comprises the wall of the ducts becomes thicker as they increase in size, and the main pancreatic duct contains smooth muscle. The branched ductules join together and drain into the main pancreatic duct, which joins the common bile duct and drains into the duodenum via the ampulla of Vater through the duct of Wirsung and the more proximal duct of Santorini.
Endocrine secretions enter the blood by the way of a fine reticular network of fenestrated capillary beds surrounding the islets of Langerhans, which consist of groups of endocrine cells of various sizes. During embryologic development the endocrine cells migrate from the duct system and aggregate around capillaries to form isolated clumps of cells scattered throughout the exocrine pancreas. Islets of Langerhans are most numerous in the tail. Each islet is supplied by as many as three arterioles, which branch into the fine capillary network and is drained by about 6 venules passing between the exocrine acini into the interlobular veins.
Both sympathetic and parasympathetic nervous systems innervate the islets of Langerhans, influencing secretions. The endocrine pancreas is composed of four types of secretory cells. The alpha and beta cells mainly secrete polypeptide hormones, glucagon, and insulin, respectively, which mainly regulate carbohydrate metabolism and have a wide variety of other effects on energy metabolism, growth, and development. Insulin promotes the uptake of glucose by liver, skeletal muscle, and adipose tissue, which lowers plasma concentration of glucose. Glucagon has metabolic effects opposite to the actions of insulin, resulting in a balanced carbohydrate metabolism. Generally, the glucagon-secreting alpha cells tend to be distributed at the periphery of the islets. Somatostatin is secreted by the delta cells of endocrine pancreas and has a wide variety of effects on gastrointestinal function, and may also inhibit insulin and glucagon secretion. The F cells secrete pancreatic polypeptide, which is involved in the regulation of endocrine and exocrine secretions.
3 Biology, Physiology, and Pathophysiology
3.1 Biology (Molecular Changes)
As an early event, ionizing radiation produces free radicals that lead to DNA damage and mitotic cell death, which is unlikely to produce sufficient damage for prolonged progression of injury. It is believed that the initial radiation event requires additional mechanisms that lead to late radiation-induced normal tissue damage, including endothelial dysfunction, increased vascular permeability, inflammation, and fibrosis. Although this phenomenon has not been well studied for the pancreas, it has been extensively researched for a number of other tissues.
Table 1
Physiology of pancreas cell sub-types
Cells
|
Secretory product
|
Physiologic actions
|
---|---|---|
Acinar
|
Secretin
|
Stimulates high bicarbonate level (HCO-3)
|
Acinar
|
Amylase
|
Digestion of starch
|
Acinar
|
Lipase
|
Digestion of fat
|
Acinar
|
Trypsin
|
Digestion of protein
|
Alpha
|
Glucagon
|
Catabolic, stimulates glycogenolysis and gluconeogenesis, raises blood glucose
|
Beta
|
Insulin
|
Anabolic; stimulates glycogenesis, lipogenesis, and protein synthesis; lowers blood glucose
|
Delta
|
Somatostatin
|
Inhibits secretion of alpha, beta, D1, and acinar cells
|
D1
|
Vasoactive intestinal polypeptide (VIP)
|
Same as glucagon; also regulates tone and motility of the gastrointestinal tract and activates 3′,5′ cyclic adenosine monophosphate (cAMP) of intestinal epithelium
|
PP
|
Human pancreatic polypeptide (hPP)
|
Stimulates gastric enzyme secretion, inhibits intestinal motility and bile secretion
|
Oxidative tissue damage and apoptosis plays an important role in abdominal irradiation, as assessed by increased lipid peroxidation, myeloperoxidase activity, and caspase-3 levels in the intestinal and pancreatic tissue. Pancreatic, as well as hepatic and intestinal myeloperoxidase activity and malondialdehyde levels are significantly increased following irradiation in an animal model (Olgac et al. 2006; Erbil et al. 2005). These phenomena may provide an amplifying mechanism for continued oxidative stress after the initial production of reactive oxygen and nitrogen species leading to prolonged progression of oxidative injury. In this study, the imbalance between oxygen-derived free radicals and antioxidant capacity appeared to be mitigated by treatment with octreotide (Olgac et al. 2006) or glutamine (Erbil et al. 2005) prior to, and following radiation, with significantly less elevation in pancreatic myeloperoxidase activity and malondialdehyde levels and accompanying inflammation compared to untreated animals.
It is presumed that an inflammatory process in which various mediators, such as eicosanoids, cytokines, and reactive oxygen metabolites contributes to the pathogenesis of radiation-induced normal tissue injury. Radiation-induced activation of a number of cytokines has been shown to play a key role in the development of chronic normal tissue injury after exposure to radiation in a number of studies. Nuclear Factor Kappa B (NFκ-B) is activated by a wide variety of agents, including hydrogen peroxide, irradiation, reactive oxygen intermediates, interleukin-1 (IL-1), Tumor necrosis factor-alpha (TNF-α), among others, and depends on the cellular redox potential. Once activated, NFκ-B transcriptionally regulates many cellular genes involved in acute phase response, and inflammatory responses (Jobin and Sartor 2000; Schmid and Adler 2000; Schmid et al. 1996).
In the prior studies, elevated pancreatic myeloperoxidase activity and malondialdehyde levels along with histological evidence of inflammation and increased apoptosis of pancreatic tissue following radiation have been correlated with NFκ-B overexpression (Olgac et al. 2006; Erbil et al. 2005) and increased caspase-3 activity (Erbil et al. 2005), both of which may contribute to late radiation-induced injury through apoptosis, and prolonged inflammation. There is no direct evidence regarding long-term complications, as animals were sacrificed 10 days after treatment, however. Both glutamine and octreotide also decreased the overexpression of NFκ-B and increased caspase-3 activity in response to radiation (Olgac et al. 2006; Erbil et al. 2005).
BCL (B Cell Lymphoma)-2 (bcl-2) is the prototype for a family of mammalian genes and the proteins they produce which govern mitochondrial outer membrane permeabilization, and can be either proapoptotic or antiapoptotic. BCL (B Cell Lymphoma)-associated X (bax) promotes apoptosis by competing with Bcl-2. Bcl-2 and bax expression have also been implicated in injury and apoptotic cell death following radiation. Radiation-induced effects on cell proliferation, expression of bcl-2 and bax proteins, and apoptosis in pancreatic tissue have been investigated utilizing a mouse model. Cell proliferation and bcl-2 expression decreased by exposure to a single dose of radiation, whereas the cell apoptosis rate and bax expression were increased (Liu and Zhong 2004).
Although the molecular mechanisms of radiation injury to the pancreas are not well characterized, they appear to be complex and involve prolonged oxidative stress, increased apoptotic death through cytokine activation and disturbed regulation of certain genes. Further investigation is warranted.
3.2 Physiology
The pancreas has two functional unites: the acinar glandular system and the islets of Langerhans, these are exocrine and endocrine components respectively. Pancreatic secretions are under neural and hormonal control (Table 1).
Acinar: Secretin provides high (HCO3) to neutralize the acid from the stomach. Enterokinases are key enzymes that are essential for digestion of starch, fat, and protein in diets. Cholicystokinin allows for reabsorption of bile.
Islet hormones: the islets are scattered and well vascularized aggregates of endocrine cells. There are four major cell types, each secreting one specific peptide hormone. Each are responsible for important physiologic functions. Most widely discussed is insulin, essential for glucogenesis and regulation gylcogenolysis.
3.3 Pathophysiology
Radiation-induced injury has traditionally been divided into an early inflammatory phase, which typically occurs between 6 and 16 weeks after radiation exposure, and a late fibroproliferative phase, which occurs much later and is characterized by diffuse fibrosis. The pathogenesis of radiation injury of the pancreas has been proposed to arise from endothelial cell damage of the microcirculation supplying acinar cells, adding ischemia to direct radiation cell damage. Acute stromal edema as a result of increased capillary permeability also plays a role (Fajardo et al. 2001). Radiation injury to myoepithelial cells and intimal fibroblasts also result in progressive fibrosis and ischemia from arterial and venular lesions and add to long-term pancreatic damage (Fajardo et al. 2001) (Fig. 4a, b, c).
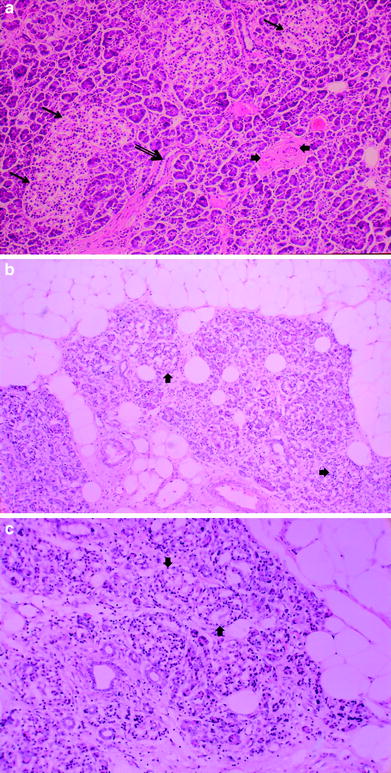
Fig. 4
a Normal pancreas. Hematoxylin and eosin (H and E) prepared normal pancreas from postmortem specimen. Most of the field consists of small, dark acini, the exocrine galnds. Three islets of Langerhans are in the upper-left half of the field (thin arrows). A small duct (thick arrow) is in the low center. Various blood vessels are in the right center (arrow heads). b Late radiation injury. Figures 4b and c demonstrate H and E prepared human pancreas several months after exposure to several thousand centigray (cGy) of megavoltage radiation to the pancreas delivered for an adjacent abdominal neoplasm. There is marked loss of the parenchyma, especially of the exocrine glands, with replacement by adipose tissue (fat cells framing the picture) in the midst of atrophic acini. The atrophic acini are dilated and separated from each other. Several islets appear to be intact (arrow heads)
3.3.1 Animal Studies
A few animal studies have focused on radiation-induced vascular and ductal damage. The vascular and connective tissues appear to have similar radiosensitivity as tissues elsewhere in the body. Zook et al. observed moderate radiation-induced occlusive vascular disease in dogs receiving abdominal neutron or photon radiation and postulated that this phenomenon could contribute to acinar and duct cell changes (Zook et al. 1983). In another study, 80 Gy was delivered to rat abdomens and histological changes were observed at intervals following exposure (Kovacs 1976). In the acute phase of radiation injury during the first 2 weeks following radiation, most of the acini remain intact with rare small necrotic foci. Edema, inflammation, and dilatation of efferent ductules with stasis consequently leads to atrophy of the acini associated with the efferent ducts over the next several months. During this period, the number of capillaries diminishes, and the number of necrotic foci increase in areas where no capillaries are visible (Kovacs 1976). These progressive late structural changes are thought to contribute to functional changes over time.
Early radiation-induced pancreatic injury results in the decline in bicarbonate and enzyme secretion shortly following radiation to the pancreas in canines and other animals (Corring et al. 1975; Pieroni et al. 1976; Volk et al. 1966). One canine study utilizing intraoperative pancreatic radiation doses of 17.5–40 Gy resulted in a progressive decrease in exocrine pancreatic function, which was evaluated by measuring N-benzoyl-l-tyrosyl-para-aminobenzoic acid, which correlated with weight loss and percentage of normal appearing acinar cells (Ahmadu-Suka et al. 1988). Autopsies performed on the dogs at 135 days following radiation demonstrated a dose-dependent degree of pancreatic atrophy in the irradiated dogs (Ahmadu-Suka et al. 1988).
In contrast islet cell damage appears to be rapidly repaired without alterations in blood glucose levels (Sarri et al. 1991). Most investigators believe that islet cells are more resistant than the acinar tissue to radiation effects (Fajardo and Berthrong 1981; Cameron and Flecker 1925; Zook et al. 1983; Ahmadu-Suka et al. 1988; Spaulding and Lushbaugh 1955). One study demonstrated that degeneration of beta cells occurs approximately 1–4 days after a single large fraction of pancreatic radiation with complete regeneration at 18 days (Cameron and Flecker 1925). Another investigator delivered single large doses of radiation to monkey pancreas and found necrosis of islet cells at 8 h, with beta cells more resistant that alpha cells (Spaulding and Lushbaugh 1955). In both studies there were no morphologic changes noted in the acinar cells during a short follow-up period (Cameron and Flecker 1925; Spaulding and Lushbaugh 1955). In addition, data suggest that the insulin function of a pancreas in rats exposed to a single 4 Gy dose is maintained at a level sufficient for ensuring adequate regulation of glucose homeostasis and of carbohydrate metabolism (Shkumatov 2004).
Similar studies evaluating histological changes and endocrine function have been performed in primates who underwent irradiation in preparation for pancreatic transplantation. Eight or 10 Gy whole body radiation was delivered over 4–5 weeks, with a maximum of 2 Gy weekly. Although the animals remained normoglycemic, insulin release was significantly reduced in both groups during irradiation and was associated with mild glucose intolerance. Histological changes included necrosis of the islet cells and acinar tissue, and cytocavitary network changes of alpha and beta cells, including degranulation, vacuolization, mitochondrial destruction, and an increase in lysosomes (Du Toit et al. 1987).
Other animal studies utilizing fractionated or single doses of radiation have demonstrated acinar gland damage (Pieroni et al. 1976; Volk et al. 1966; Wellmann et al. 1966). Early ultrastructural changes include acinar cell degeneration, mitochondrial injury, and vesicles in endoplasmic reticulum cisternae, which are evident within 1 h following single dose radiation exposure (50–90 Gy) on electron microscopy and are associated with reduced enzyme secretion (Volk et al. 1966). After 8 days following radiation, numerous membrane-bound cytoplasmic bodies, endoplasmic reticulum containing entrapped degenerating mitochondria, and reduced zymogen granules are observed (Volk et al. 1966).
Acini exposed to radiation are known to exhibit increased autophagy, however, these changes do not appear to be related to radiation-induced lysosomal degradation of intracellular proteins (Somosy et al. 1996; Telbisz et al. 2002). Islet cells show similar degenerative organelle changes that are repaired efficiently and last up to 3 weeks, during which time there is no alteration in blood glucose levels (Cameron and Flecker 1925; Volk et al. 1966; Spaulding and Lushbaugh 1955). Longer follow-up demonstrates a recovery period from 3–5 weeks, consisting of increasing endoplasmic reticulum and gradual disappearance of autophagic remnants. After about 5–12 months, the ultrastructure of the cells returns to normal morphologic appearance with the exception of persistent interstitial fibrosis. Conversely, pancreatic function does not completely recover, and continues to exhibit a persistent decrease in enzyme production compared to pretreatment (Wellmann et al. 1966).
In another early study utilizing fractionated pancreatic radiation (45–50 Gy over 30 days) in nine dogs whose pancreas had been surgically mobilized in the abdomen, Archambeau et al. performed functional studies of blood glucose and pancreatic secretions obtained through fistulae (Archambeau et al. 1966). Samples of the pancreatic head and tail were also obtained at 2, 3, and 6 months after radiation demonstrated progressive interstitial fibrosis with distorted normal pancreatic architecture, and preserved acini and the islet morphology. No changes in secretory volume, serum, and pancreatic electrolytes or blood glucose were detected, but there was an initially elevated serum amylase, which declined progressively (Archambeau et al. 1966).
Injury to small- and medium-sized ducts resulting in occlusion of the lumen by cell debris has been demonstrated after 50 Gy fractionated radiation combined with 25 Gy intraoperative radiation to the pancreas in dogs. Over time, severe interstitial fibrosis was observed and by 135 days small- to medium-sized arteries and veins were narrowed with medial and adventitial fibrosis (Ahmadu-Suka et al. 1988). Others have demonstrated that local radiation impairs microcirculatory blood flow in healthy pancreas in a rat ductal pancreatic tumor model. A single fraction of 15 Gy resulted in increased tumor apoptosis but no change in tumor blood flow, whereas blood flow and functional capillary density in the normal pancreas was impaired 5 days after radiation, resulting in hypoperfusion (Ryschich et al. 2003). These changes are thought to contribute significantly to decline in pancreatic exocrine dysfunction and late parenchymal structural damage.
3.3.2 Human Studies
There is even less data describing radiation damage in humans following radiation to the pancreas. As mentioned earlier, Case and Warthin described atrophy of the acinae and degeneration and necrosis of pancreatic ducts in 3 patients with radiation-induced hepatitis (Case and Warthin 1924). Subsequently, in 1955, Brick observed pancreatic fibrosis during autopsies of three men who received radiation to the para-aortic lymph nodes for testicular cancer (Brick 1955). In another report, autopsies of patients treated with abdominal radiation for lymphoma demonstrated peripancreatic stromal fibrosis, sometimes severe, with myointimal proliferation in arteries. The authors described the appearance as similar to chronic pancreatitis with lack of active inflammation and necrosis (Fajardo 1982). Woodruff et al. performed postmortem studies of 22 patients who received radiation for pancreatic cancer using 50–60 Gy over 30 fractions delivered with helium ions. After a median of 9 months following radiation, approximately 85 % of patients demonstrated radiation injury in the nontumor bearing pancreas consisting of dense fibrosis, mainly located in the exocrine parenchyma. They reported that no patient died of pancreatic insufficiency and only one patient developed hyperglycemia (Cohen et al. 1985; Woodruff et al. 1984).
Because of the paucity of human specimens, very little is known regarding early radiation injury to the human pancreas and we predict early radiation damage by extrapolating from the animal studies previously described. After about 10–20 Gy, acinar injury is observed, similar to animal studies. Inflammatory cells are abundant and the number of secretory granules is reduced and the cytoplasm becomes vacuolated. Similar to other tissues, the amount of cellular damage increases with increasing fraction size. Zonal necrosis and ductule degeneration are observed at 60 Gy. Although larger ducts may only demonstrate mild cell changes and enlargement, smaller ducts develop distended lumens and plugging by cellular debris. Acute injury to arterioles and venules may also be present and associated with edema of the stroma and acute inflammation in regions of early parenchymal necrosis. In contrast, islet capillaries show relatively normal anatomical structure during the early injury phase without endothelial enlargement or fibrin thrombi, and islets of Langerhans appear normal (Fajardo et al. 2001).
Pathologic examination of patient pancreas specimens examined at 6 months or more after treatment with radiation demonstrate shrunken, fibrotic pancreas with thickening of the fibrous capsule and adhesions to the surrounding tissues grossly. Lobulation becomes less prominent, and large ducts may be dilated (Fajardo et al. 2001). Microscopic examination of irradiated pancreas at the same time interval demonstrate fibrotic parenchyma with severe acinar cell loss, abundant collagen, hyaline, and atypical fibroblasts similar in appearance to chronic pancreatitis. Despite these structural changes, islet cells appear normal. Occasionally fat necrosis and calcifications may be found (Case and Warthin 1924; Levy et al. 1993). At this time point, very little inflammation is found with the exception of small collections of lymphocytes trapped within the dense fibrous connective tissue. Arteriole walls are thickened with fibrosis of the intima and narrowed lumens while smaller arteries and veins demonstrate fibrous intimal plaques comprised of foam cells (Berthrong 1986). The number of ductules are reduced or atrophied, and plugged with cellular debris while larger ducts are dilated with enlarged nuclei (Fajardo et al. 2001).
Others have studied pancreatic function following abdominal radiation. In one study, the early and late effects of intraoperative radiation on the exocrine and endocrine functions of the residual pancreas were examined in 54 patients with pancreatic head resection, 20 whom underwent intraoperative radiation, and 34 who did not (Yamaguchi et al. 2000). Fasting blood sugar levels and glucose tolerance tests demonstrated no change at 2 months, but at 6 months the glucose tolerance was compromised compared to the preoperative baselines in both groups, while fasting blood sugar levels remained normal. The total amount of pancreatic juice drainage in the irradiated patients was about half as much as that in nonirradiated group. In addition, univariate and multivariate regression analyses demonstrated that intraoperative radiation was a significant independent factor in the decline of pancreatic exocrine function (N-benzol-L-tyrosyl-p-aminobenzoic acid excretion) in the early postoperative period (Yamaguchi et al. 2000).
4 Clinical Syndromes (Endpoints)
A variety of endpoints can be considered to reflect RT-associated pancreatic dysfunction, as shown in Table 2. It is sometimes useful to categorize the endpoints, albeit somewhat arbitrarily, as shown.
Table 2
Representative endpoints that might reflect radiation-induced pancreas injury, segregated (somewhat arbitrarily) as shown
Focal
|
Global
|
|
---|---|---|
Subclinical
|
Regional imaging abnormalities; e.g., calcifications on planar images, hyperenhancement on CT, or biliary duct abnormalities on retrograde cholangiopancreatography
|
Latent diabetes mellitus, glucose intolerance, malabsorption of proteins and fats, reduced levels of pancreatic enzymes in small intestine, or abnormalities in urinary bentiromide
|
Clinical
|
Cancer induction
|
Diabetes mellitus, steatorrhea
|
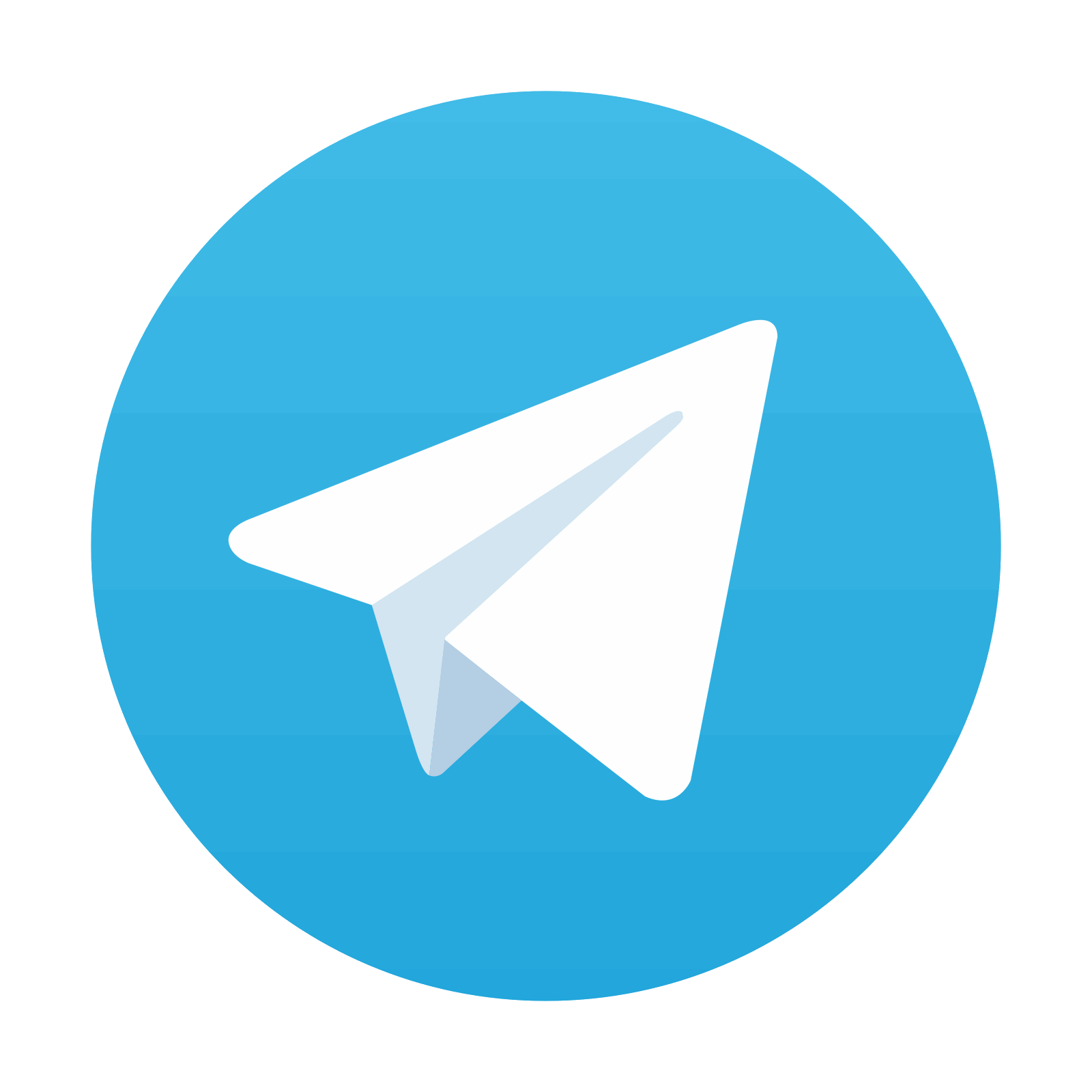
Stay updated, free articles. Join our Telegram channel

Full access? Get Clinical Tree
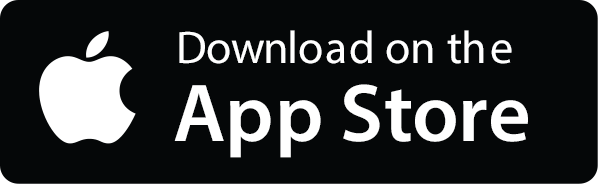
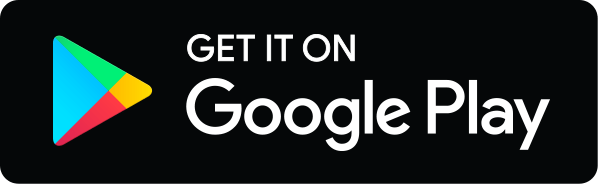