Abstract
Sonodynamic therapy (SDT) is a novel non-invasive treatment method that combines low-intensity ultrasound and sonosensitizers. Compared with photodynamic therapy, SDT has the advantages of deeper tissue penetration, higher accuracy and fewer adverse reactions. Sonosensitizers are essential for the efficacy of SDT. Sonosensitizers have the advantages of clear structure, easy monitoring, evaluation of drug metabolism and clinical transformation, etc. Notably, biochemical techniques can be used in the field of sonosensitizers and SDT to overcome inherent barriers and achieve sustainable innovation. This article first summarizes the molecular mechanism of SDT, focusing on organic sonosensitizers, inorganic nano-sonosensitizers and multi-functional drug delivery systems with targeting, penetration and imaging functions after a series of modifications. This review provides ideas and references for the design of sonosensitizers and SDT and promotes their future transformation into clinical applications.
Introduction
Cancer is currently one of the most challenging problems in medicine, which seriously threatens human health [ ]. Traditional treatment methods include surgical resection, radiotherapy and chemotherapy, but there are contraindications, drug resistance and long-term adverse reactions that affect the quality of life of patients [ ]. Therefore, researchers have focused on developing novel “minimally invasive” or even “non-invasive” treatment strategies.
Some non-invasive therapies, such as photodynamic therapy (PDT) and sonodynamic therapy (SDT), are gradually gaining attention. Among them, PDT uses photosensitizers to generate reactive oxygen species (ROS) or photothermal conversion reagents to generate heat to kill cancer cells. It has the advantages of minor toxic side effects, no drug resistance and high selectivity and has been used in clinical cancer treatment [ , ]. However, due to the limited penetration ability of light in biological tissues, phototherapy is more suitable for treating superficial tissue tumors and its therapeutic effect on deep tumors is limited [ ]. SDT, derived from PDT, has the advantages of good tissue penetration, less damage to surrounding normal tissues and low skin sensitivity, so it has been widely considered by researchers [ ]. Under ultrasound irradiation, sonosensitizers can transfer energy to the surrounding oxygen or water molecules to generate ROS to kill cells [ ].
In general, the killing ability of SDT in tumor cells is mainly related to three factors: the frequency and power of ultrasound, the oxygen content in the tumor area, and the type of sonosensitizer. Among them, the sonosensitizer is the most critical component [ ]. At present, the widely reported sonosensitizers are mainly organic sonosensitizers (such as porphyrins and phthalocyanine, etc.), inorganic sonosensitizers (such as titanium oxide, etc.) and a series of nano-sonosensitizers, including covalent organic frameworks, metal-organic frameworks, metal oxides and organic molecular materials. Among them, organic sonosensitizers (porphyrins and their derivatives) have good biocompatibility and easy modification. However, severe skin photosensitivity, low chemical/biological stability and poor tumor enrichment [ ]. Inorganic sonosensitizers have high stability, but low sonosensitizing efficiency and poor biocompatibility [ ] limit the effect of sonosensitive molecules in treating tumors. Therefore, constructing an ideal sonosensitizer is critical for effectively treating SDT. An ideal sonosensitizer should have good biocompatibility, high bioavailability and precise tumor targeting [ ]. Researchers have designed various nano-medicine delivery systems based on proteins, organic polymers and inorganic materials [ , ]. The nano-medicine delivery system has the advantages of improving the solubility of the sonosensitizer, prolonging the circulation time of the encapsulated sonosensitizer in vivo , and reducing the toxic side effects to improve its therapeutic effect, which provides a broader application scenario for SDT [ ].
Tumor sites have enhanced permeability and retention (EPR) effects due to abundant neovascularization and lymphatic damage [ ]. Nano-medicine delivery systems can be passively enriched in the tumor site based on this effect, but the clinical treatment effect is not improved [ , ]. Studies have found that the complex microenvironment at the tumor site makes the drug stay in the stroma near the tumor blood vessels after reaching the tumor site, and it is difficult to reach the deep area of the tumor, thus showing heterogeneous distribution at the tumor site and reducing the therapeutic effect. Some researchers have proposed to use biological materials, such as exosomes, bacteria, viruses and different mammalian cells (such as blood cells, sperm cells and macrophages) as natural biological vectors [ , ]. Biological drug delivery vectors can be used efficiently due to their long circulation time in vivo , deformable ability to penetrate biological barriers, avoidance of immune surveillance and strong chemotaxis [ , ].
In this review, we first review the mechanism of SDT, elaborating on the interaction between SDT and ROS. The design strategy and changing characteristics of the new nano-sonosensitizer are also discussed to provide a reference for improving the efficiency of SDT.
Mechanisms of SDT
Despite the apparent advantages of SDT, the underlying mechanisms remain elusive. It is generally believed that the main mechanisms of SDT include the ultrasonic cavitation effect, ROS effect and thermal effect ( Fig. 1 ). It has been widely recognized that ultrasonographic SDT cooperatively causes cell death through multiple mechanisms. However, the exact mechanism of SDT still needs further investigation. This part summarizes the possible mechanisms of SDT generation by sonosensitizers under ultrasound irradiation.
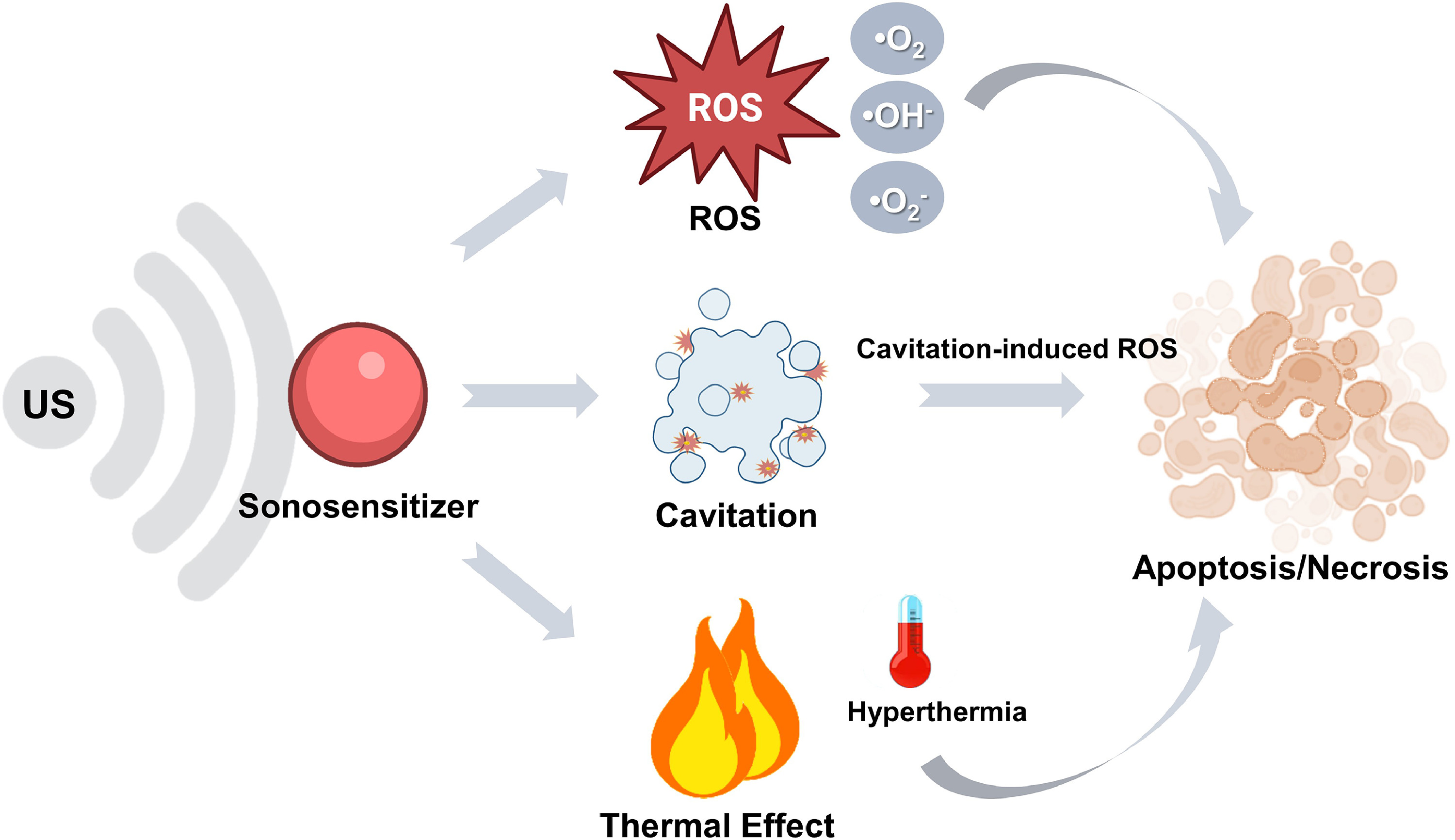
Ultrasound cavitation
Ultrasonic cavitation is closely related to sonodynamic phenomena. Ultrasonic cavitation is a classical ultrasonic non-thermal effect, which is the interaction between the sound field and the bubble and can be divided into inertial cavitation and non-inertial cavitation [ ]. During inertial cavitation, the cavitation volume steadily increases to the resonance volume, which triggers the explosion [ ]. Ultrasonic pressures at or above the threshold result in immediate microbubble rupture. The shock wave generated during rupture will form a steep rise in temperature and pressure in a small space, destroying the membrane structure and skeleton of the cell and thereby killing the cell [ , ]. Mechanical shear forces from non-inertial cavitation may also contribute to tumor cell death by disrupting the cell membrane (CM) [ ]. Studies have found that SDT has the potential to destroy the blood-tumor barrier and hinder the formation of the vascular lumen, resulting in the reduction of blood flow and energy supply, thereby inhibiting tumor growth [ ].
Under ultrasound irradiation, the gas in the liquid oscillates during compression and decompression cycles, thereby generating microfluidics and radiation forces. This oscillation increases drug penetration and diffusion by interfering with adjacent cell surfaces and membranes [ ]. When bubbles burst rapidly due to exposure to ultrasound, energy is released and high temperatures and pressures are generated, which lead to cell necrosis. Strong shock waves are also generated by the collapse of cavitation microbubbles, thereby causing mechanical damage and cell destruction [ , ]. Bubbles burst rapidly as a result of exposure to air, releasing energy. This results in high temperature and high pressure, leading to necrosis of the target cells. In addition, the intense fragmentation of microbubbles also drives the entry of extracellular sonosensitizers into the cell and generates sonodynamic activity, thereby accelerating the death of the target cells. Therefore, ultrasound-induced cavitation is one of the essential mechanisms for killing tumor cells. In addition to the inhibitory and destructive effects of cavitation on tumor growth, it is also a significant cause of ROS production during SDT.
ROS generation
ROS are toxic by-products of aerobic respiration and include singlet oxygen (•O 2 ), hydroxyl radical (•OH − ) and superoxide anion (•O 2 − ) [ ]. Like PDT, the ROS mechanism in SDT disrupts the REDOX balance of tumor cells, leading to cell death. Under US irradiation, the sonosensitizer absorbs energy from the ground state to the high-energy. Then, the high energy state returns to the low energy state, and a large amount of energy is released, accompanied by the generation of ROS, which can effectively damage intra-cellular DNA and protein, promote intra-cellular lipid peroxidation, affect intra-cellular signaling pathways, and lead to the activation of apoptotic protein caspase-3 [ ], Apoptosis of target cells was induced to inhibit tumor growth [ ]. In addition, sonosensitizers-mediated therapy can cause a decrease in mitochondrial membrane potential and changes in cytoskeletal morphology. There have been many attempts to treat diseases using SDT to produce ROS. In addition, the cavitation effect, pyrolysis and electroluminescence (sonoluminescence, SL) are also considered ultrasound exposure and sensibilize causes of ROS [ , ].
Thermal effects
Another mechanism by which ultrasound-mediated therapy can destroy tumor tissue is by increasing the tissue temperature to destroy the target tissue [ , ]. The absorption and transformation of ultrasonic mechanical energy lead to thermal energy generation when the ultrasound propagates in the tissue. If the low-energy ultrasound focuses on the lesion, the target tissue is heated to a higher temperature, leading to irreversible thermal damage [ ].
Application of different materials sonosensitizers in SDT
Sonosensitizers are indispensable tools in the SDT process. It converts acoustic energy into chemical energy through its unique chemical structure to produce ROS for SDT. Therefore, the performance of the sonosensitizer can significantly affect the efficiency of SDT. The construction of an ideal sonosensitizer with high ROS generation capacity is a critical factor for efficient SDT treatment. The widely reported sonosensitizers are mainly organic sonosensitizers (porphyrins and their derivatives) and inorganic sonosensitizers (titanium oxide, etc.). Organic sonosensitizers have the advantages of good biocompatibility and easy modification. But severe skin photosensitivity, low chemical/biological stability and poor tumor enrichment. Inorganic sonosensitizers have high stability, but their low sonosensitizers efficiency and poor biocompatibility limit the therapeutic effect of sonosensitive molecules to inhibit and kill tumors.
Some researchers have designed a variety of nano-biological drug delivery systems based on proteins, organic polymers and inorganic materials, which have the advantages of improving the solubility of sonosensitizers, extending the circulation time of encapsulated sonosensitizers in vivo , and reducing toxic side effects to improve their therapeutic effect, providing broader application scenarios for SDT ( Fig. 2 ). This article reviews the application of organic small-molecule sonosensitizers and inorganic materials as sonosensitizers and briefly introduces the application of inorganic-organic biological materials as carriers of sonosensitizers.
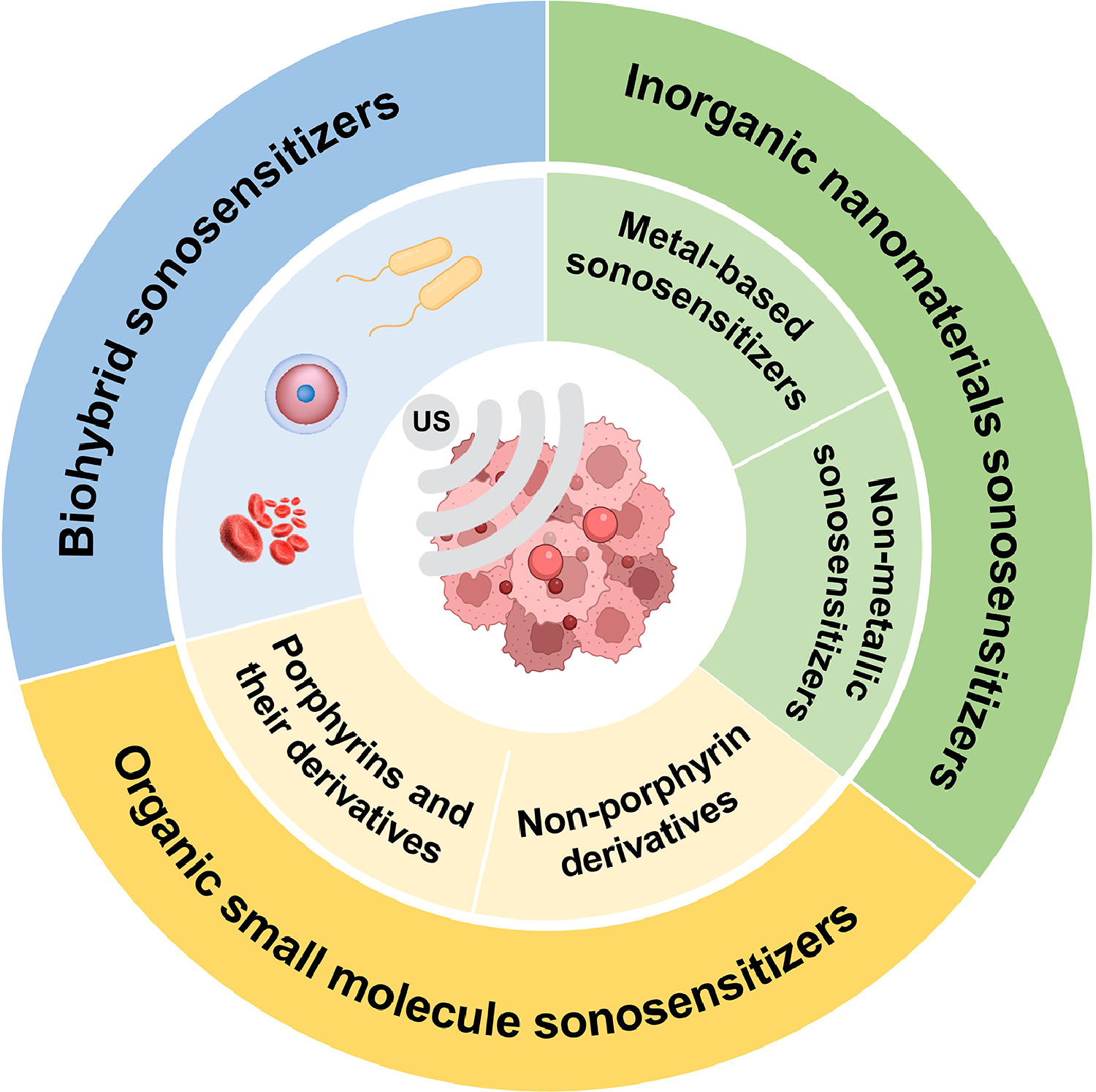
Organic small molecule sonosensitizers
Some organic small molecules can be excited to produce ROS at therapeutic ultrasound frequencies ranging from 1 to 3 MHz. The most commonly reported small molecule sonosensitizers are porphyrin derivatives, such as protoporphyrin Ⅸ, ATX-70, chlorin e6 (Ce6) and hematoporphyrin (HP). Other non-porphyrin natural small molecules, such as rose bengal (RB), curcumin (CUR), chlorophyll, artemisinin and 5-aminolevulinic acid, have also been demonstrated to possess sonodynamic effects. The organic small molecule sonosensitizers materials and their application are summarized in Table 1 .
Category | Sonosensitizers | Therapy | Biological model | Refs. |
---|---|---|---|---|
Porphyrins and their derivatives | HMME | SDT | Endometrial cancer cells | 39 |
PpIX | SDT | Hepatoma cell line H22 | 40 | |
ZnPcs | SDT | HepG2 cells | 41 | |
Non-porphyrin derivatives | Rose red B | SDT | Sarcoma cells | 42 |
Eosin B | SDT | Sarcoma cells | 44 | |
CUR | SPDT | HepG2 Cells | 48 |
Porphyrins and their derivatives
Porphyrins and their derivatives are the most widely studied organic sonosensitizers with excellent sonosensitizers efficiency and catalytic properties. Porphyrins and their derivatives have no anti-tumor activity but show sonodynamic activity when subjected to ultrasound. For example, HP, HP methyl ether, ATX-70, PpIX, etc [ ]. Porphyrins, as first-generation photosensitizers, are widely used in PDT, and they are also the first generation of sonosensitizers [ ]. In 1990, Umemura et al. confirmed that ultrasound combined with HP could enhance the killing effect on cells through in vivo and in vitro experiments. They proposed the anti-tumor mechanism of HP activated by ultrasound to produce ROS for the first time [ ]. HP monomethyl ether (HMME) is a second-generation HP-related sensitizer of two porphyrins. Sun et al. investigated the anti-tumor effect of HMME-mediated SDT on endometrial cancer cells. SDT promotes ROS production, decreases MMP, and increases intra-cellular Ca 2+ concentration. This induces apoptosis in endometrial cancer cells [ ]. PpIX, a protoporphyrin derivative, is an essential representative of carboxy-modified porphyrin. Wang et al. examined the effect of PpIX on hepatoma cell line H22 in the presence of low-intensity ultrasound. The results confirmed that SDT using PpIX combined with ultrasound successfully induced apoptosis of H22 cells, and a series of events occurred in the mitochondria during the death process, including the release of mitochondrial protein cytochrome c, the redistribution of Bcl-2 family proteins Bid and Bax, and the reduction of mitochondrial membrane potential [ ].
However, aggregation of conventional sonosensitizers occurs under physiological conditions, resulting in poor water solubility and pharmacokinetic performance. Moreover, the accumulation of porphyrin molecules can affect the energy transfer between porphyrin and oxygen molecules, resulting in low ROS production, which needs to be solved urgently. Zinc phthalocyanine (ZnPcs) as photosensitizers exhibit high photodynamic ROS production due to their high conversion efficiency from singlet to triplet states. According to these unique properties, ZnPcs are promising sonosensitizers. Recently, artemisinin derivatives have been found to have sonodynamic activity. Zhao et al. [ ] designed a phthalocyanine artesunate conjugate (ZnPcT4A) that produces twice as much ROS as porphyrin IX. Biological studies have shown that ZnPcT4A has high anti-cancer activity and biological safety, which opens up a new way to develop efficient organic sonosensitizers.
Non-porphyrin derivatives
Some dye-based compounds have also been used in SDT, such as fluorescein, bamboo erythromycin B, eosin, erythropoietin, etc. RB B is a basic fluorescent dye that was once used as a food additive. Umemura et al. [ ] treated sarcoma cells with rose red B under ultrasound and found significant damage to sarcoma cells, deducing that rose red produced singlet oxygen under ultrasound. Zou et al. [ ] investigated the sonodynamic damage of BSA induced by fluorescein and its derivatives. The UV spectrum showed a noticeable hyperchromic effect, and the fluorescence spectrum showed quenching. This indicates that BSA is significantly damaged, disulfide bonds are broken, and tryptophan and tyrosine are exposed. Eosin B red powder is easily soluble in water, the solution has green fluorescence, and it is soluble in ethanol. Biostain and histology were used to stain epithelial cells, muscle fibers and nuclei. He et al. [ ] also demonstrated the sonodynamic activity of eosin B by the method described above, and an increase in the concentration of eosin B led to a greater degree of cell damage. Wang et al. [ ] The bacterial family unit formation and viability tests were used to observe the changes in Staphylococcus aureus after sonication and hypoxia-erythromycin B. The permeability and DNA fragmentation of the CM were also studied. The results showed that when the concentration of hypocretin was 500 μM and the ultrasonic irradiation time was 50 minutes, the S. aureus was significantly destroyed. At the same time, it was found that the DNA was not broken, and the integrity of the CM was lost. It was concluded that hypoxigenin caused the death of S. aureus by damaging the CM and increasing the membrane permeability under ultrasound.
The killing ability of some anti-tumor drugs, such as cisplatin, daunorubicin and adriamycin, is significantly enhanced when combined with ultrasound. Cisplatin has cytotoxicity, inhibiting the DNA replication process of cancer cells and damaging their CM structures. It is clinically used in ovarian cancer, prostate cancer and so on. Irajira et al. [ ] A sonosensitive nanoplatform (ACA) consisting of alginate coloaded with cisplatin and gold nanoparticles (AuNPs) was constructed. When stimulated with ultrasound, cisplatin showed a solid ability to induce cancer cell death, demonstrating the anti-tumor effect of the ACA nanoplatform in combination with ultrasound. The thermal and mechanical effects of ultrasound can enhance the therapeutic effect of conventional chemotherapy methods. Doxorubicin (DOX), a commonly used anthracycline anti-biotic used to treat a variety of cancers, causes DNA damage, apoptosis, production of oxygen and hydroxyl radicals, and enhances cavitation effects. An et al. [ ] prepared a degradable hollow polydopamine nanoparticle (P@HP) by co-loading DOX and Ce6 and modified by mitochondrial triphenylphosphonium (TPP) to encapsulate platinum nanoparticles (Pt). Under ultrasound, the nanocomposite enriches the tumor site by enhancing the permeability and retention effect of solid tumors, followed by killing tumor cells by releasing DOX to inhibit cellular DNA replication.
Some natural products can produce sonosensitivity under ultrasound due to their unique properties. CUR, the main component of curcuma longa, has anti-tumor effects and low toxicity to normal cells. In addition, CUR can also induce tumor cell apoptosis as a sonosensitizer. To introduce more functions and prepare more efficient sonosensitizers, Zhu et al. [ ] prepared CUR-loaded poly ( l -lactic-co-glycolic acid) microbubble (CUR-PLGA-MB). They found that CUR-PLGA-MB had a more significant anti-tumor effect in PDT compared to SDT or PDT and that CUR-PLGA-MB was a promising sound/photosensitizer.
Although these sonosensitizers all have strong sonodynamic effects, most organic sonosensitizers have poor water solubility, unstable properties in the microenvironment and short blood circulation time, which cannot accumulate in large amounts at the tumor site to produce enhanced EPR effect [ ]. Therefore, finding new science and technology to effectively improve the defects of existing sonosensitizers and exploring new sonosensitizers are urgent problems to be solved in SDT.
Inorganic nanomaterials sonosensitizers
Inorganic sonosensitizers have higher stability and superior physicochemical properties than organic sonosensitizers and have been widely used in biomedical fields. Many inorganic materials can be excited by ultrasound to produce ROS. Most of these materials are semiconductor materials with unique energy band structures. In addition, inorganic materials, as nanocarriers, can load various components and more easily achieve a variety of functions. The inorganic nanomaterials sonosensitizers in recent years are summarized in Table 2 . Sonosensitizers can be classified into two types based on the main components present in the nanostructures: metallic and non-metallic types [ ].
Category | Sonosensitizers | Therapy | Biological model | Refs. |
---|---|---|---|---|
TiO 2 nanostructures-based sonosensitizers | HTiO 2 NP | SDT | SCC7 cells | 53 |
Manganese-based composite materials | MO@FHO | SDT | MDA‐MB‐231 cells | 58 |
MnCO 3 NPs | SDT | 4T1 cells | 60 | |
Other metal-based sonosensitizers | BMO NRs | SDT | HeLa cells | 61 |
Silicon-based sonosensitizers | Si-Pt NCs | SDT/CDT | 4T1 cells | 64 |
Carbon-based sonosensitizers | MnO x /TiO 2 -GR-PVP | SDT/PTT | 4T1 cells | 65 |
PHFs | SDT | Colon 26 tumors | 66 | |
C-dots MBs | SDT | TRAMP cells | 68 |
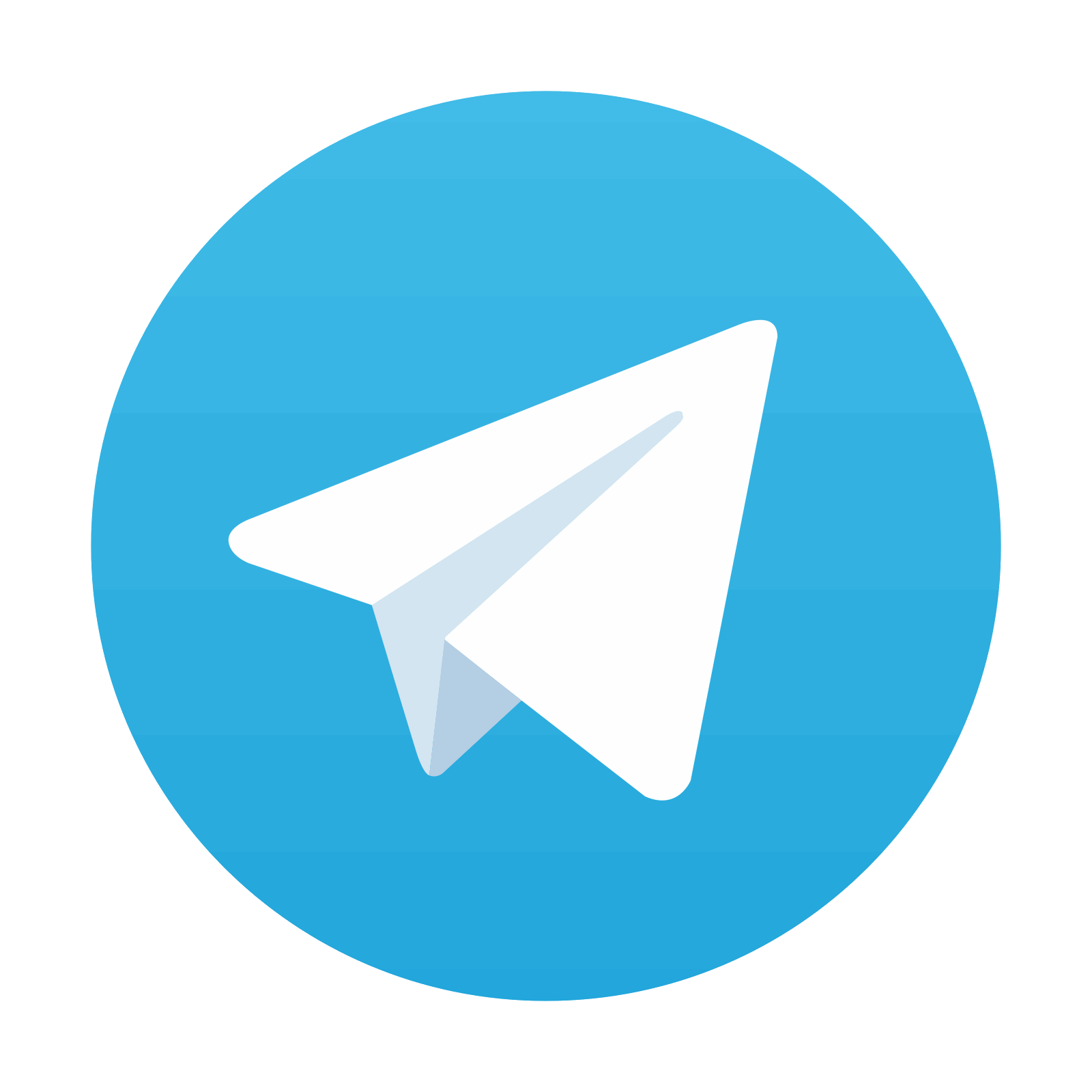
Stay updated, free articles. Join our Telegram channel

Full access? Get Clinical Tree
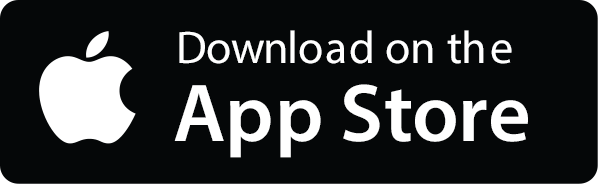
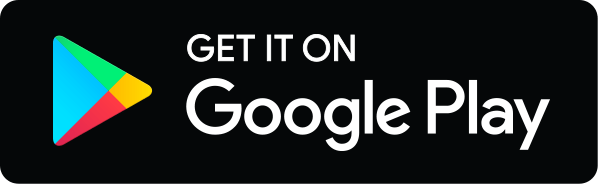
