Tumour
Hormone
Organ
Gastrinoma
Gastrin
Pancreas, duodenum
Insulinoma
Insulin
Pancreas
Glucagonoma
Glucagon
Pancreas
VIPoma
Vasoactive intestinal peptide
Pancreas
Carcinoid
Serotonin, substance P
Small intestine/prox. colon, lung
Medullary thyroid cancer
Calcitonin
Thyroid
Phaeochromocytoma
Norepinephrine
Adrenals
Somatostatinoma
Somatostatin
Pancreas
Depending on the cell physiology, different approaches can be used to enable tumour-specific molecular imaging. For tumours of sympatho-adrenal lineage (phaeochromocytoma, neuroblastoma), the catecholamine pathway is one possible approach to visualise pathologic cell distribution. Metaiodobenzylguanidine (mIBG) labelled with 123I or 131I is an adrenaline analogue, which gets stored in intracellular vesicles, but not further metabolised [7] (more on this subject in Chap. 6).
Another characteristic feature of neuroendocrine tumour cells is the frequent overexpression of somatostatin receptors on the cell surface [8, 9]. Of the five known main classes of somatostatin receptors, the subtype sstr2 is predominant in most NENs [8, 9]. Several subtypes can be expressed depending on differentiation, type and anatomic site of primary [8, 9]. Yet there is vast variation in sstr expression even between tumours of the same type.
5.4 Somatostatin
Somatostatin is a small regulatory peptide hormone, which is distributed in the central and peripheral nervous system, endocrine glands, immune system and gastrointestinal tract. Two active forms of somatostatin are known, one consisting of 14 amino acids and the other of 28 amino acids.
Generally somatostatin is an inhibitory hormone which inhibits the release of several secondary hormones. The actions of somatostatin are mainly located to the pituitary where the secretion of growth hormone and TSH is inhibited as well as in the gastrointestinal system, where the release of numerous of different gastrointestinal and pancreatic hormones is suppressed. Additionally somatostatin modulates the gastrointestinal motility, suppresses the exocrine action of pancreas as well as inducing apoptosis.
5.4.1 Somatostatin Receptors
The biologic actions of somatostatin are mediated through one of the six somatostatin receptor subtypes known to date (sstr 1, 2A, 2B, 3, 4, 5) [3, 10, 11]. These receptors are all G-protein coupled and activate a variety of different intracellular pathways such as inhibition of adenyl cyclase, stimulation of phosphotyrosine phosphatase (PTP) and modulation of mitogen-activated protein kinase (MAPK) [11–13].
After binding of an activating ligand on the receptor, the hormone-receptor complex gets internalised in the cell and directed to endosomes. The somatostatin receptor will then get recycled back to the cell membrane or degraded. The degradation of somatostatin receptors can subsequently lead to sstr downregulation on the cell surface [9–13].
Somatostatin receptors are physiologically expressed in a number of normal human tissues and organs, such as pituitary, brain, gastrointestinal tract, pancreas, kidney, immune cells, blood vessels and peripheral nervous system.
In addition several neoplasias (NET and non-NET) express somatostatin receptors [9, 10]; a selection of tumours is shown in Table 5.2. Clinically subtype sstr2 is of most importance as somatostatin and somatostatin analogues bind to sstr2 with nanomolar affinity [9, 10] and sstr2 is the subtype which is most frequently overexpressed by NENs [9, 10]. The other subtypes vary in overexpression in NENs as well as in their affinity for synthetic somatostatin derivatives [14].
Table 5.2
Neoplasias potentially expressing somatostatin receptors
Gastrinoma |
Glucagonoma |
Paraganglioma |
SCLC |
Carcinoid tumours |
Merkel cell carcinoma |
Neuroblastoma |
Phaeochromocytoma |
Medullary thyroid carcinoma |
Pituitary tumours |
Breast cancer |
Prostate cancer |
Lymphoma (NHL and Hodgkin’s) |
Colon cancer |
Ovarian cancer |
Melanoma |
Sarcoma |
Renal cell carcinoma |
Astrocytoma |
5.5 Classification
The classification of NENs has been confusing and to date no general consensus has been agreed upon. Several different approaches have been attempted in order to classify the heterogeneous NENs to ensure optimal prognostic stratification and treatment schemes. Yet no universal classification for all NET sites is available. Because NENs predominantly arise in the gastroenteropancreatic or bronchopulmonary system, the following segment will deal mainly with classification of NETs from these organ systems.
In general NENs get classified by histology, grading, proliferation, anatomic site of origin, hormone secretion and/or extent of disease. Because many of the characteristics of the NETs are organ/site specific, location of the primary site can be a relevant stone in the therapy scheme. Therefore a classification by site of origin can be helpful in the clinical handling. The NETs are commonly divided into foregut, midgut and hindgut tumours based upon their embryonic segments and similar biological behaviour. NETs located in the small intestine and proximal colon (midgut) are often associated with typical carcinoid symptoms caused by serotonin hypersecretion, whereas NETs in the distal colon and rectum (hindgut) are usually non-functional [2, 15, 16]. Pancreatic and bronchial NETs (foregut) can be associated with hormone hypersecretion, but can be non-functional as well [2, 15, 16].
NETs can be histologically distinguished in well-differentiated NETs, poorly differentiated NECs and mixed adeno-neuroendrocrine carcinomas [17, 18]. The well-differentiated NETs are divided into low-grade and intermediate NETs dependent on the cell morphology, mitotic rate and/or Ki67 index of “hot spots” (Table 5.3). The Ki-67 index plays an important role in the NET classification, due to the good correlation of Ki-67 index and survival [19–22]. Additionally the Ki-67 index has proven to be an independent prognostic factor for survival, regardless of disease extent [23–27]. However, no significant difference in outcome between G1 and G2 carcinoids has been noted. The more aggressive poorly differentiated NECs are distinguished from the NETs using Ki-67 index/mitotic rate as well as showing a different pathological appearance. Additionally the NECs show a more rapid course with shorter survival and are generally treated with a platin-based chemotherapy scheme (Table 5.4) [28].
Table 5.3
Grading system for neuroendocrine neoplasms
Grade | Gastrointestinal tract and pancreas [18] |
---|---|
Low (G1) | <2 mitoses/10 HPF and Ki-67 index ≤2 % |
Intermediate (G2) | 2–20 mitoses/10 HPF or Ki-67 index 3–20 % |
High (G3) | >20 mitoses/10 HPF or Ki-67 index >20 % |
Table 5.4
ENETs stratification proposal for GEP-NETs
Prognosis | Differentiation | Grade | Stage | Potential therapy |
---|---|---|---|---|
Localised tumour | ||||
Very low riskof metastasis | Well differentiated | G1 | T1 | Endoscopic resection |
Low risk | Well differentiated | G1 | T2 | Surgery |
Intermediate risk | Well differentiated | G2 | T1 | Surgery |
High risk | Well differentiated | G1/G2 | T2 | Surgery |
High risk | Poorly differentiated | G3 | T1–T3 | Surgery, additional treatment |
Nodal metastases | ||||
Slow growth | Well differentiated | G1 | T1–T3, N1 | Surgery |
Intermediategrowth | Well differentiated | G2 | T1–T3, N1 | Surgery, additional treatment |
Fast growth | Poorly differentiated | G3 | T1–T3, N1 | Surgery, additional treatment |
Nodal anddistant metastases | ||||
Slow growth | Well differentiated | G1 | Any T, N1, M1 | Surgery, additional treatment |
Intermediategrowth | Well differentiated | G2 | Any T, N1, M1 | Surgery, additional treatment |
Fast growth | Poorly differentiated | G3 | Any T, N1, M1 | Chemotherapy |
The World Health Organisation guidelines provide a classification to the extent of the disease using the tumour-node-metastasis (TNM) staging. Due to the vast biological diversity of NETs, the extent of the disease alone does not provide an adequate risk stratification or survival data. Many NETs have already metastasised by the time of first diagnosis, making a stratification based solely on the TNM system inadequate. For example, NETs of unknown primary are by definition stage IV in the TNM system and the histological grade may prove the only prognostic parameter.
Functionality may have an impact on the prognosis, yet the biological behaviour of most functioning NETs get defined by grade and stage. The clinical symptoms caused by hypersecretion of hormones define the division in functioning or non-functioning NETs. The immunohistological staining is not a defining criterion, only the presence of typical symptoms. Yet a functional tumour may be treated differently than a non-functional; therefore the presence of hormonal production should be included in the tumour nomenclature.
5.6 Diagnostical Tracers (Somatostatin Receptor-Based Imaging)
Due to the short half-life of somatostatin in human serum of 2–3 min caused by rapid enzymatic degradation, new somatostatin analogues with longer half-lives were necessary. The development led to the cyclooctapeptide octreotide, which still is very much in clinical use today. The “cold” octreotide and lanreotide are used in the treatment of acromegaly as well as antiproliferative therapy and symptom control of NETs. There are short-acting as well as long-acting products available.
The cyclic peptide additionally served as lead structure for most of the later-developed somatostatin analogues such as tyrosine3-octretotide (TOC), tyrosine3-ocreotate (TATE) and naphthylalanine3-octreotide (NOC). Octreotide and octreotide derivatives show a general high affinity to sstr2 with varying affinity to the other somatostatin receptor subtypes [34].
The radiolabelled somatostatin analogues generally consist of a biological active part, usually octreotide, TOC or TATE coupled with a chelator complex (i.e. DTPA, DOTA, EDDA/HYNIC) and a gamma emitting radiometal. The internalisation of the radioactive ligand-receptor complex with intracellular entrapment of the radiometal is considered the mechanism enabling tumour imaging by good scintigraphic tumour-to-background ratio, especially in delayed images.
With the indium-111-labelled DTPA-octreotide (OctreoScan), the first commercially available tracer enabling visualisation of in vivo somatostatin receptor distribution came on the market and still is the gold standard within gamma scintigraphy. Later DOTA- and HYNIC/EDDA-conjugated analogues, new tracers, found their way into the clinical routine. 68Ga-DOTA-labelled derivatives prove the best imaging modality available, because of the superior qualities of PET/CT imaging. However, this modality is still restricted to only a few specialised centres and at time being no PET tracers are registered for clinical use, whereas three different tracers are registered for use in the generally widespread gamma scintigraphy.
5.6.1 [111In]-DTPA-D-Phe1-Octreotide
[111In]-DTPA-D-Phe1-octreotide (or [111In]-pentetreotide) has been commercially available over two decades and is the most frequently used somatostatin analogue in scintigraphy. Due to the relatively long half-life of 111In of 2.8 days, sequential multiple-day imaging can be performed. The cyclotron product 111In is a gamma-emitting radiometal with two medium-energy photo peaks (173 and 247 keV). Medium-energy collimator is used during imaging and data from both windows are added.
The recommended intravenously applied activity for adults undergoing a somatostatin receptor study is 185–222 MBq, resulting in an effective dose of around 12 mSv (0.054 mSv/MBq) [35]. For children the recommended activity is 5 MBq/kg [35, 36]. The amount of peptide injected is only around 10–20 μg; hence significant pharmacologic effects are not expected. Before administration, a control of the labelling yield according to the manufacturer’s instructions is recommended. The radiolabelled product should be applied within 6 h after preparation.
Common indications for somatostatin receptor imaging are staging of patients with NENs, detection and localisation of neuroendocrine tumour lesions, follow-up of patients with NENs and evaluation of patients planned to undergo peptide receptor radionuclide therapy. In Table 5.5 a selection of tumours (NETs, non-NETs) and non-malignant processes is shown.
Table 5.5
Sensitivity of somatostatin receptor imaging using [111In]-pentetreotide
Tumour | Sensitivity |
---|---|
Pituitary tumours | High (>75 % detection rate) |
Gastrinomas | High (>75 % detection rate) |
Carcinoids | High (>75 % detection rate) |
Non-functional P-NETs | High (>75 % detection rate) |
Functional P-NETs except insulinoma | High (>75 % detection rate) |
Paragangliomas | High (>75 % detection rate) |
SCLC | High (>75 % detection rate) |
Merkel cell carcinoma | High (>75 % detection rate) |
Meningiomas | High (>75 % detection rate) |
Sarcoidosis and granulomatous diseases | High (>75 % detection rate) |
Graves’ disease and ophthalmopathy | High (>75 % detection rate) |
Insulinomas | Intermediate (40–75 % detection rate) |
Medullary thyroid carcinoma | Intermediate (40–75 % detection rate) |
Differentiated thyroid carcinoma | Intermediate (40–75 % detection rate) |
Lymphoma (NHL, HL) | Intermediate (40–75 % detection rate) |
Phaeochromocytoma | Intermediate (40–75 % detection rate) |
[111In]-DTPA-D-Phe1-octreotide is registered for use in scintigraphic localisation of primary and to determine the extent of metastatic NET’s disease.
Patients undergoing somatostatin receptor imaging should generally be well hydrated before and at least 1 day after injection, to reduce radiation burden. If the patient does not suffer from diarrhoea, a mild laxative should be administered the day before and in the evening after injection. Long-acting somatostatin analogues should be withdrawn 5–6 weeks prior to injection, eventually switching to short-acting preparations until 1 day before the study in order to prevent receptor occupation which potentially leading to false negative findings. Naturally the usual precautions for nuclear medicine studies for pregnant and breastfeeding women apply. [111In]-pentetreotide should not be administered into lines for total parenteral nutrition, because of the possible formation of a complex glycosyl octreotide conjugate.
After injection [111In]-pentetreotide is rapidly cleared from the plasma: just 35 % of the injected radioactivity remains in the bloodstream after 10 min. Twenty-four hours after application only 1 % still remains in the blood. The tracer excretion is almost completely renal: 85 % of the administered activity is eliminated with urine within 24 h. Hepatobiliary and faecal excretion accounts for less than 2 % [35–37]. The biologic half-life of 111In-pentetreotide is 6 h.
The normal physiologic distribution pattern (Fig. 5.1) of [111In]-DTPA-octreotide features visualisation of the thyroid, spleen, liver, kidneys and occasionally the pituitary and adrenals as well as the urinary bladder, gall bladder and bowel. In 15 % of the female patients diffuse uptake in the breasts can be seen. The tracer uptake in the thyroid, spleen, adrenals and pituitary is somatostatin receptor mediated, whereas the kidney visualisation is caused by reabsorption of the radiotracer in the tubular cells. Normally bowel uptake is not seen on the early images (4 h after injection), but can occur on the delayed images. Therefore sequential images can be helpful to distinguish pathological and physiological tracer bowel uptake. A recommended protocol for acquisition parameters is shown in Table 5.6.
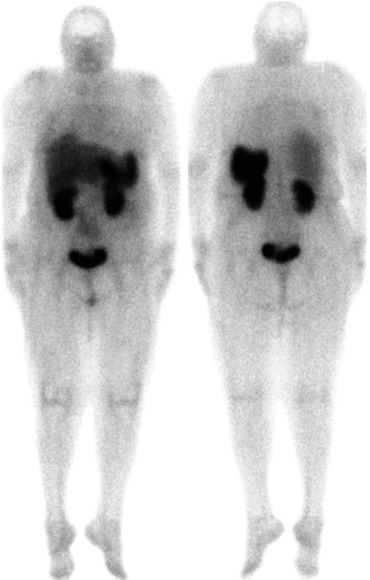
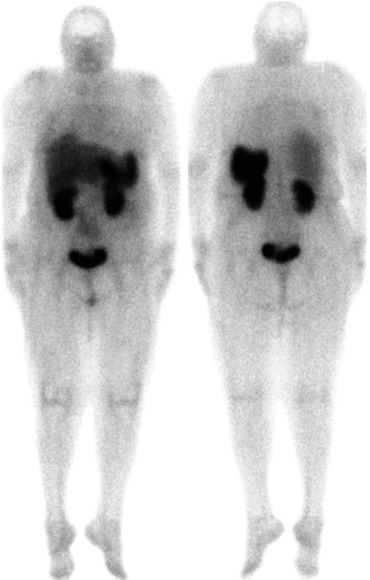
Fig. 5.1
Normal [111In]-pentetreotide whole body scan with physiologic sstr distribution
Table 5.6
Acquisition protocol for 111In-pentetreotide
Collimator | Medium energy, parallel hole |
---|---|
Energy window | 172 and 245 keV with 20 % windows summed in the acquisition frames |
Planar images (anterior and posterior) | 4 h p.i. (7 min), 24 h p.i (10 min), 48 h p.i. (15 min)per view |
128 × 128 or 256 × 256 matrix | |
Whole body (256 × 1,024 matrix) | Scanning speed: 4 h p.i., 10 cm/min; 24 h p.i., 7 cm/min; 48 h p.i., 5 cm/min |
SPECT or SPECT/CT | 4 h p.i.: 3° angular sampling, 20 s per projection; 24 h p.i.: 3°, 30 s, 48 h: 6°, 60 s |
128 × 128 acquisition matrix |
The tumour uptake kinetic is relatively slow, taking at least 4 h [36, 37]. Therefore images are acquired 4, 24 and 48 h after administration. The 4-h images are obtained to evaluate the abdomen before any activity in the gut appears. Planar whole body scans provide a good overview of the distribution of the radiopharmaceutical. Due to the lower tumour-to-background ratio compared to the later images, some tumour lesions may not be visible in the early images. Any relevant non-physiological uptake indicates the presence of lesions with overexpression of somatostatin receptors, which is usually suspicious for or concordant with malignant disease. Yet increased focal or diffuse density of somatostatin receptors can be caused by a numerous of benign processes. In Tables 5.7 and 5.8, pitfalls and potential misinterpretation of positive and negative results are shown [36, 37]. Furthermore the antibiotic bleomycin may cause local pulmonary accumulation of [111In]-pentetreotide.
Table 5.7
Pitfalls and false positive results causing potential misinterpretation
Radiation pneumonitis | Bacterial pneumonia |
Accessory spleen | Cerebrovascular accident |
Scar tissue | Granulomatous disease |
Gallbladder uptake | Diffuse breast uptake |
Nodular goitre | Adrenal uptake |
Focal stool collection | Urine contamination |
Ventral hernia | Concomitant second tumour |
Common cold (nasal uptake) | Respiratory infections |
Table 5.8
False negative results causing potential misinterpretation
Presence of unlabelled somatostatin caused by octreotide therapy | Liver metastases may appear iso-intense to normal liver tissue |
Presence of unlabelled somatostatin caused by tumour secretion | Variable sstr2 expression of the tumour lesions |
Different sstr subtypes of the tumour lesions | Variable tumour differentiation |
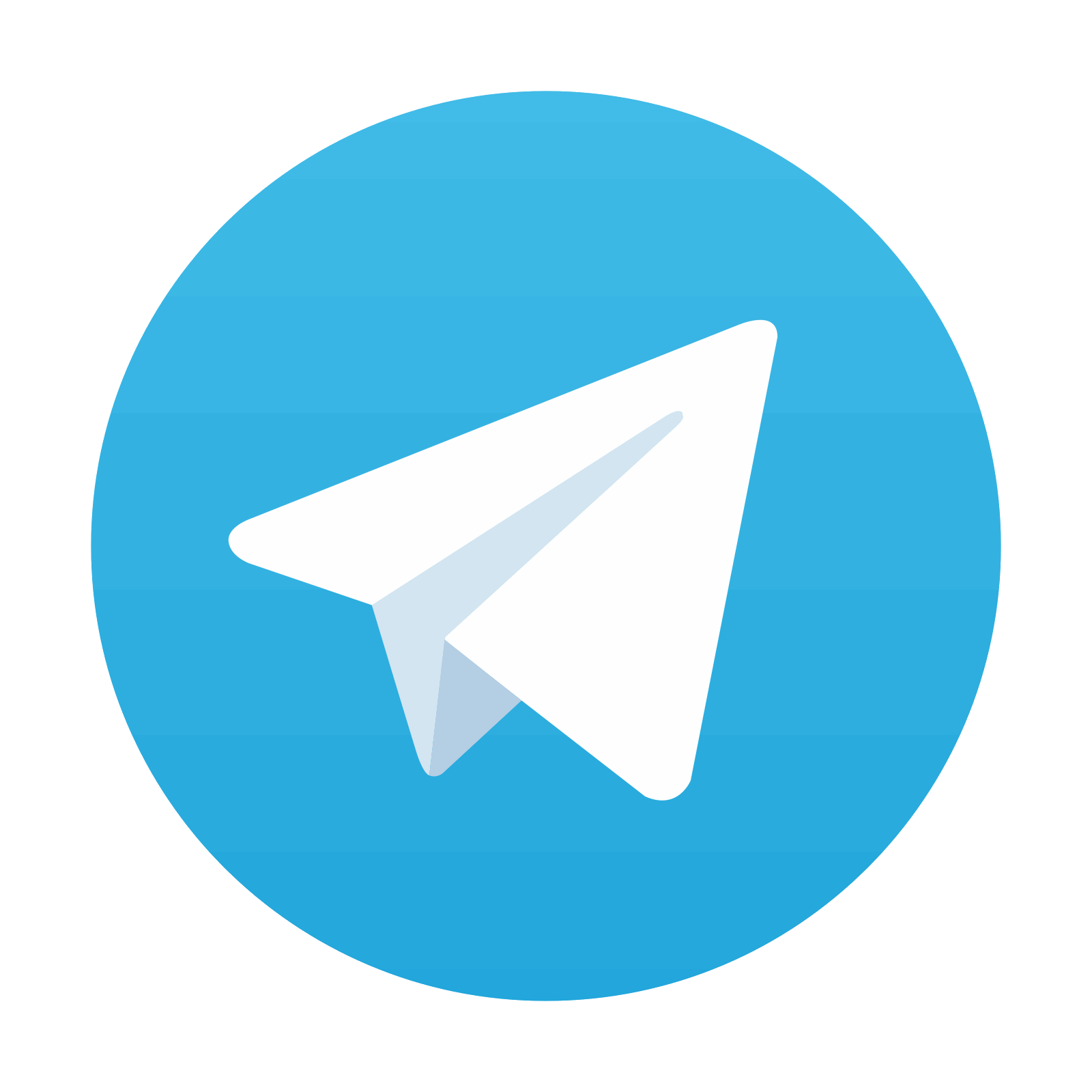
Stay updated, free articles. Join our Telegram channel

Full access? Get Clinical Tree
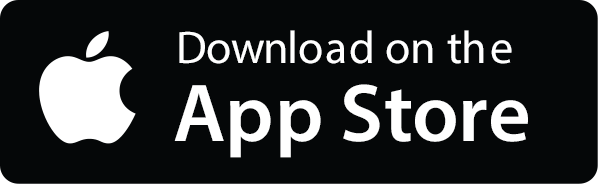
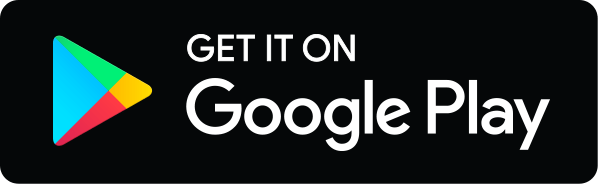