Representative effective dose valve (mSv)
Representative effective dose valve (mSv)
Chest X-ray PA and lateral
0.1
0.05–0.24
Diagnostic invasive coronary angiography
7
2–16
Coronary CT angiography
64-slice MDCT, retrospective gating
12
9–19
64-slice MDCT, reduced tube voltage
6
3–8
64-slice MDCT, prospective triggering
3
2–4
264 or 320 MD row CT
4
2–8
The purpose of this manuscript is to outline the specific anatomic considerations for TAVR, to describe the aortic valvar complex and its components, and to outline how these anatomic structures are influenced by the aortic THV selected.
Fatigue Testing and Long-Term Durability
Long-term durability is a critical design component for aortic THVs. The Food and Drug Administration (FDA) Draft Guidance for Industry and FDA standards for Heart Valves published in 2010 provides an overview of the preclinical requirements for durability testing of prosthetic heart valves, including aortic THVs [6]. THV regulatory submissions are required to contain a “risk analysis” for the aortic THVs in which the probability and degree of harm associated with a device due to frame or valve malfunction is estimated and an appropriate plan is outlined to mitigate these risks [6]. Required testing includes material property testing (e.g., stainless steel, cobalt chromium, nitinol), biologic safety, hemodynamic and structural performance, device durability, component fatigue testing, and device-specific testing [6].
A required component to the aortic THV and THV component durability testing is accelerated wear testing (AWT), described in ISO 5840:2005 documents [6]. AWT is performed for 600 million cycles in rigid valves and their components and 200 million cycles in flexible valves [6]. Pulsatile flow and physiologic loading conditions are tested using a variety of physiologic parameters, including radial, linear, and axial compression, bending, torsion, shear stress and fluid drag, among other physiologic stresses (Fig. 8.1, Box 8.1). Aortic THV and the load-bearing, nonbiological components of flexible replacement heart valves (e.g., stent wire) are individually fatigue tested to 600 million cycles [6]. Animal studies are also recommended to assure long-term biologic stability and human post-mortem studies may be useful to understand the tissue interactions of THVs once implanted [6].

Fig. 8.1
Physical stress properties with THV. Radial compression (a) describes loading applied in a net uniform circumferential detection perpendicular to the centroidal axis of the frame resulting in a reduction of the cross-sectional diameter. Linear compression (b) applies to loading applied in a nonuniform manner predominantly from the side of the frame perpendicular to the centroidal axis resulting in a “flattened” elliptical cross-sectional profile. Bending (c) characterizes the behavior of the frame when loading is applied in a nonuniform manner resulting in a flexure (bending) of the frame that the centroidal axis post-loading is arced versus linear. Torsion (d) characterized the behavior of the frame when torque loading is applied perpendicular to the centroidal axis resulting in an angulated shear deformation of the frame that the centroidal axis is “twisted” versus linear. Axial compression (e) describes loading in a net uniform manner across the ends of the frame parallel with the centroidal axis resulting in shortening in the length of the frame. Shear stress (f) applies to directional loading in a non-normal manner predominantly from one side of the frame either parallel or tangential to the centroidal axis resulting in a canted structure about an unchanged linear centroidal axis. Valve closing loads (g) characterizes the behavior of the frame when blood pressure loading is applied across the closed valve during diastole resulting in local deformations of the frame in vicinity of the commissural attachment points. Fluid drag (h) is defined as the loading applied in a net axial direction imposed by wall shear stresses resulting from flow interactions with the inner surface of the valve (Courtesy of Medtronic, Inc)
The development of preclinical test conditions for aortic THVs is based on the estimation of the “anticipated clinical environment” (i.e., physiologic conditions) of the deformed and calcified aortic annulus in elderly patients. A description of these conditions is more challenging when the native aortic valve is not removed and remained in place [6]. MDCT reconstructions in patients treated aortic THVs have been useful in determining these boundary limits [7], but these studies are limited by their resolution to identify more subtle changes in aortoventricular compliance. A more precise characterization of aortic THVs in their physiology state may be provided by 3D TEE. Creation of an imaging database that included MDCT and TEE to determine boundary limits for the testing of aortic THVs is needed.
Box 8.1 Physiologic Forces on TAVR Valves (see Fig. 8.1)
-
Radial
-
Linear
-
Axial compression
-
Bending, torsion
-
Valve closing loads
-
Fluid drag
General Properties of Aortic Transcatheter Heart Valves (THV)
Aortic THVs have been designed to displace the native aortic valve and replace it with a bioprosthetic valve that expands and conforms to the native aortic valvar complex. This is typically performed using either a balloon-expandable or self-expanding frame or polymeric sleeve. Aortic THVs vary by the access site, sheath size, composition of the supportive frame and valve leaflets, length of the frame and valve leaflets, length and thickness of the skirt used to prevent perivalvular regurgitation (PVR), and retrievability and repositionability. Design components of contemporary aortic transfemoral THVs are found in Table 8.2, and anatomic requirements for these valves are found in Table 8.3. Each valve interacts with the underlying aortic valvar complex in unique ways due to their unique design components.
Table 8.2
General design characterization of aortic transfemoral transcatheter heart valves
Manufacturer |
OD sheath (Fr) |
Frame |
Deployment |
Sizes |
Valve material |
Skirt material |
Retrievable | |
---|---|---|---|---|---|---|---|---|
SAPIEN |
||||||||
Ascendra |
Edwards |
22
24 |
Stainless Steel |
Balloon expandable |
23, 26 |
Bovine pericardium |
Bovine pericardium |
No |
XT |
Edwards |
18 |
Cobalt Chromium |
Balloon expandable |
23, 26 |
Bovine pericardium |
Bovine pericardium |
No |
CoreValve |
Medtronic |
18 |
Nitinol |
Self-expanding |
23, 26, 29, 31 |
Porcine pericardium |
Porcine pericardium |
Partially |
Portico |
St. Jude |
18 |
Nitinol |
Self-expanding |
23 mm |
Bovine pericardium |
Porcine pericardium |
Complete |
Sadra Lotus |
Boston Scientific |
18 |
Nitinol |
Self-expanding |
23 mm, 27 mm |
Bovine pericardium |
Bovine pericardium |
Complete |
Direct Flow |
Direct Flow Medical |
18/22 |
Saline injection |
23 mm |
Bovine pericardium |
Polymer Nylon sleeve |
Partially |
Table 8.3
Aortic valvar requirements for aortic transfemoral transcatheter valves
Aortic annulus |
Annular eccentricitya |
SOV width (mm) |
Coronary height (mm) |
SOV height (mm) |
Tubular aorta (mm) |
AV angle (°) | |||
---|---|---|---|---|---|---|---|---|---|
Diameter (mm) |
Area (mm2) |
Perimeter (mm) | |||||||
SAPIEN Ascendra |
|||||||||
23 mm |
18–23 |
254.5–415.2 |
56.5–72.3 |
– |
– |
– |
– |
– |
Any |
26 mm |
23–25 |
415.2–490.9 |
72.3–78.5 |
– |
– |
– |
– |
– |
Any |
CoreValve |
|||||||||
23 mm |
18–20 |
254.5–314.2 |
56.5–62.8 |
– |
≥25 |
– |
≥15 |
≤34 |
<70 |
26 mm |
20–23 |
314.2–415.2 |
62.8–72.3 |
– |
≥27 |
– |
≥15 |
≤40 |
< 70 |
29 mm |
23–27 |
415.5–572.6 |
72.3–84.8 |
– |
≥29 |
– |
≥15 |
≤43 |
<70 |
31 mm |
26–29 |
530.9–660.5 |
81.6–91.1 |
– |
≥29 |
– |
≥15 |
≤43 |
<70 |
Sadra Lotus |
|||||||||
23 mm |
18–23 |
254.5–415.2 |
56.5–72.3 |
– |
– |
>14 (>11) |
– |
– |
Any |
27 mm |
23–27 |
415.2–572.6 |
72.3–84.8 |
– |
– |
>14 (>11) |
– |
– |
Any |
Portico |
|||||||||
23 mm |
19–21 |
283.4–346.2 |
59.7–65.9 |
≥0.7 |
≥27 |
NA |
≥15 mm |
≤36 (≤38) |
<30 (<45) |
Direct Flow |
|||||||||
23 mm |
19–23 |
283.4–415.2 |
59.7–72.3 |
– |
– |
– |
– |
– |
Any |
Edwards SAPIEN Balloon–Expandable THV
The SAPIEN Valve (Edwards Lifesciences, Irvine, CA) is a trileaflet bioprosthesis made of bovine pericardium mounted on a balloon-expandable stainless steel stent. The distal half of the stent contains a fabric cuff that limits stent expansion and decreases perivalvular insufficiency. The valve is currently available as a 23 mm valve whose frame height is 14.5 mm and a 26 mm valve whose frame height is 16 mm (Fig. 8.2a). The newer generation SAPIEN XT has modified scallop-shaped leaflets that provide superior hemodynamic properties and coaptation that are mounted on a cobalt-chromium alloy stent with thinner struts, giving it a lower profile. Both valves are available in 23 and 26 mm sizes. In contrast to the RetroFlex 3 transcatheter delivery system that requires introduction of a 22 or 24 Fr sheath, respectively, the NovaFlex delivery system has reduced the sheath size to 18 Fr for the 23 mm XT valve and 19 Fr for the 26 mm valve.
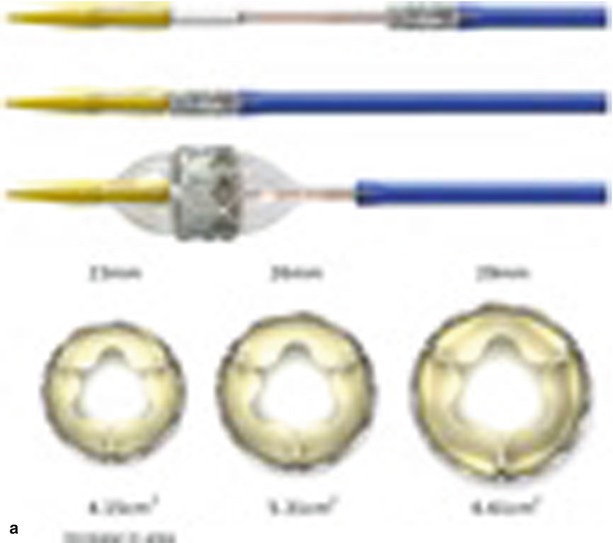
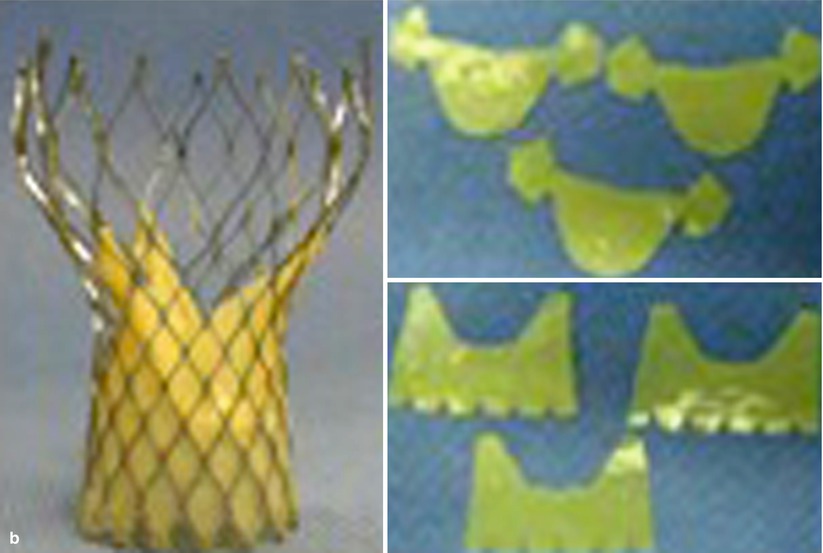
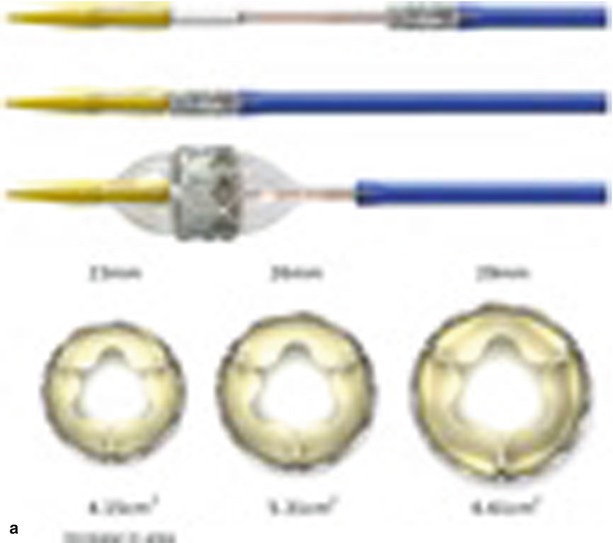
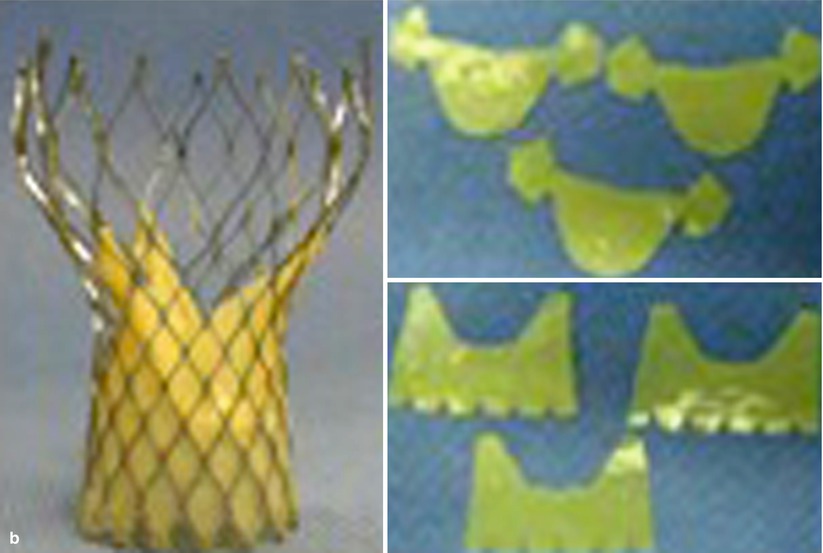
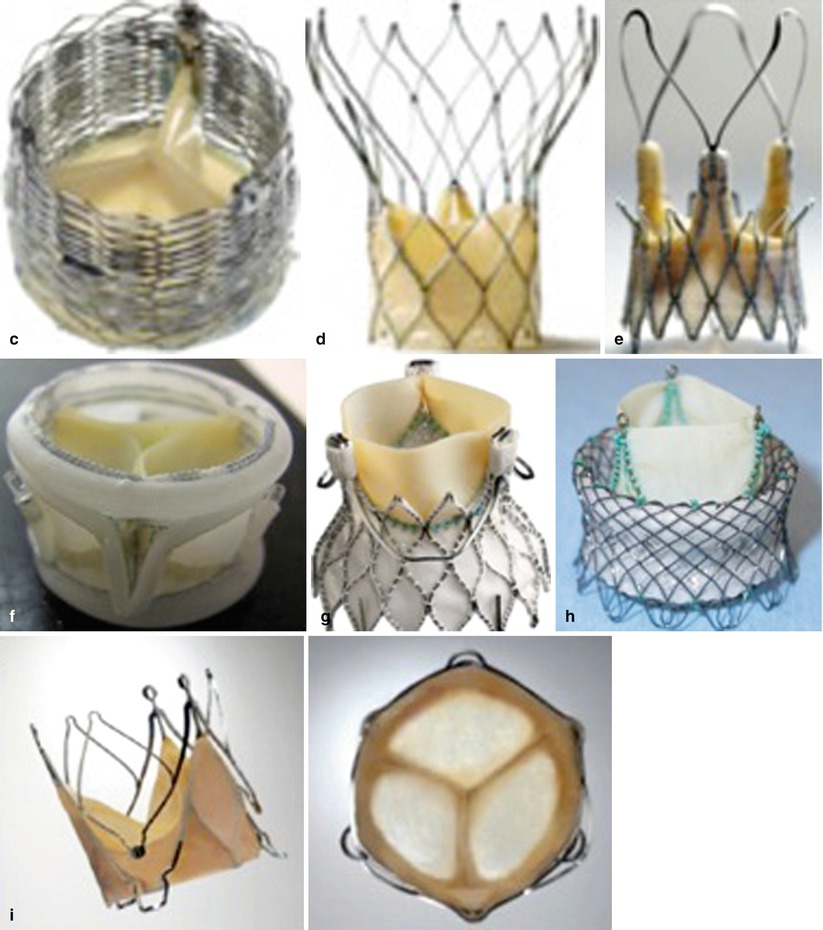
Fig. 8.2
Aortic transcatheter heart valves. Edwards SAPIEN (a), Medtronic CoreValve (left panel) and six porcine pericardial components (right panel) (b), SADRA Lotus (c), St. Jude Portico (d), Direct Flow Medical (e), Symetis ACURATE (f), Medtronic Engager (g), Heart Leaflet Technology (h), and JenaValve (i)
CoreValve Revalving System
The CoreValve Revalving System (Medtronic, Inc., Maple Grove, MN) is comprised of three components that include a self-expanding nitinol support frame with cells configured in a diamond cell design that anchors a trileaflet porcine pericardial tissue valve, an 18 Fr delivery catheter, and a disposable loading system. CoreValve frames are currently available in 23, 26, 29, and 31 mm sizes for an aortic diameter range of 18–20, 20–23, 24–27, and 26–29 mm, respectively (Fig. 8.2b). The nitinol frame that is approximately 50 mm in length was specifically designed for optimal functionality, stability, and durability (Fig. 8.2b) and has three levels of radial and hoop strength. The frame inflow exerts high radial expansive force to secure the frame within the aortic annular location. The strength of this portion of the frame prevents annular recoil allows the frame to partially conform to the noncircular shape of the aortic annulus. In addition, it prevents frame migration and minimizes the occurrence of perivalvular leaks. The “constrained” center portion of the frame has high hoop strength that resists size and shape deformation, which is critical as this portion of the frame contains the “supra-annular” valve leaflets. The frame is concave at this location to avoid the coronary ostium with the native valve and allows coronary cannulation after the implantation. The outflow portion of the frame exerts low radial forces and serves to orient the frame to the aorta parallel to flow through the valve. The porcine pericardium was selected due to its lower profile (compared with bovine pericardium) and durability, and the three leaflet elements are constructed with long commissures to enhance durability.
Portico Self-ExpandingTHV
The Portico THV (St. Jude Medical, St. Paul, MN) consists of a delivery catheter that contains a soft tapered nose cone located on an 18 Fr capsule that contains a trileaflet bovine pericardial valve that is attached to the frame using three retention tabs [8] (Fig. 8.2c). A porcine pericardial sealing cuff is used to minimize perivalvular leak. The Portico valve is sized according to the nominal external stent diameter at the valvular level and is available in a 23 mm size [8]. A desirable feature is the potential to be deployed to the point of functionality while allowing for controlled recapture, followed either by repositioning and redeployment or by removal [8].
Sadra LOTUS Self-Expanding
The Lotus Valve System (Sadra Medical, Inc., Los Gatos, California) is a trileaflet bovine pericardial valve that is attached to a self-expanding nitinol mesh by three supporting posts (Fig. 8.2d). There is an adaptive seal at the lower end of the frame that is designed to conform to the aortic annulus and prevent perivalvular leak. The Lotus valve is available in 23 and 27 mm sizes and is delivered via an 18 Fr Sheath. If the THV does not conform appropriately to the annulus due to undersizing or incomplete expansion, it can be recaptured and retrieved prior to final release. The radial expansive force of the valve renders the aortic annular more circular than ellipsoid after deployment.
Direct Flow Medical
The Direct Flow Medical THV (Direct Flow Medical, Inc., Santa Rosa, California) is designed as a trileaflet bovine pericardial tissue valve that is attached to an inflatable framework with a slightly tapered, conformable polyester fabric cuff [9] (Fig. 8.2e). The device system is comprised of a bovine, trileaflet pericardial tissue valve, a sheathed delivery and recovery system, and a Dacron fabric cuff that is inflated with saline-contrast to conform to the native annulus. Ventricular and aortic cuff rings are independently inflatable. After the saline-contrast cuff inflation ensures the position and sealing of the device with fluoroscopy, the saline-contrast mixture is exchanged for an inflation medium that solidifies and hardens to form a permanent support structure [10].
Symetis ACURATE TA
The Symetis ACURATE TA (Symetis, Ecublens Switzerland) is composed of a surgical-quality porcine tissue valve mounted on a self-expandable nitinol stent that is partially covered by a polyethylene terephthalate (PET) skirt (Fig. 8.2f) [11]. The delivery system has a flexible isodiametric shaft and ergonomic design allowing for a controlled, sheathless, atraumatic, and two-step implantation [11]. It is available primarily as a transapical device, but retrograde designs are currently under consideration.
Engager Valve
The Engager™ Aortic Valve bioprosthesis (Medtronic, Inc., Minneapolis, MN, USA) is a biological heart valve prosthesis composed of three leaflets, cut from tissue fixated bovine pericardium, sewn to a polyester sleeve, and mounted on a compressible and self-expanding nitinol frame (Fig. 8.2g) [12]. The stent assembly consists of a shaped main frame and a support frame, which are coupled together so as to form the commissural posts of the valve [12]. The bioprosthesis is available in 23 and 26 mm size with an inflated frame length of 25.5 and 27.5 mm, respectively [12]. These two sizes are designed to fit an effective aortic annulus MDCT between 21 and 26.5 mm, corresponding to a TTE annulus diameter between 19 and 26 mm [12].
JenaValve
The JenaValve™ AVR system (Jena Technologies, Munich, Germany) is designed as a trileaflet porcine root tissue valve. It is attached to a low-profile nitinol stent with flexible stent posts supporting the valve leaflets and positioning feelers for accurate placement of the prosthesis with regard to the native valve leaflets [13] (Fig. 8.2h). The valve prosthesis is available in 23, 25, and 27 mm sizes for implantation in native aortic annuli ranging from 21 to 27 mm in diameter. The transapical device is available using a sheathless 32 Fr delivery catheter [13].
Heart Leaflet Technologies
The Heart Leaflet Technologies (HLT) valve (Heart Leaflet Technologies, Inc., Maple Grove, Minnesota) is comprised of a trileaflet porcine pericardial tissue valve, a superelastic nitinol wire form that supports the valve structure, a superelastic nitinol mesh, and a braided polyester liner integrated within the support structure to prevent regurgitant flow around the valve (Fig. 8.2i).
Noninvasive Assessment of the Aortic Valvar Complex
Noninvasive characterization of the aortic valvar complex includes the aortic annulus, sinus of Valsalva (SOV), coronary arteries, tubular aorta, left ventricular outflow tract, and the aortomitral continuity which are all considered for aortic THV selection (Table 8.3). The central component of the complex is the aortic valve that is typically comprised of three semilunar leaflets that are anchored in the basal annular plane and extend to the tubular aorta (Fig. 8.3).
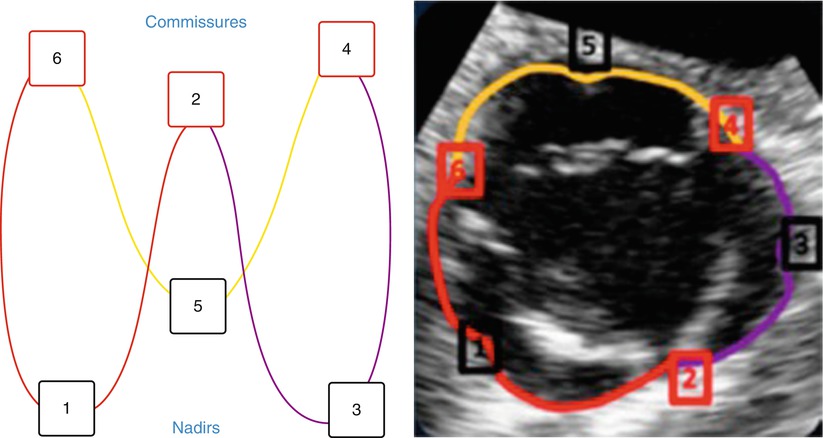
Fig. 8.3
The aortovalvular backbone. Annulus orientation as seen on two-dimensional aortic short axis view. Red – right coronary cusp annulus, purple – left coronary cusp annulus, yellow – noncoronary cusp annulus, 1 – 3 – 5 Nadirs, 2 – 4 – 6 commissures
Aortic Annulus
Proper sizing of the aortic annulus has become a central component of TAVR. The aortic annulus generally has an elliptical shape with anchor points at the nadir of each of three aortic leaflets. The caudal valvar insertion points extend into the left ventricular outflow tract forming a virtual ring that is referred to as the basal annular ring. The true anatomic ventricular arterial juncture is located approximately 3 mm superior to basal annular ring [14–16]. While earlier clinical studies used 2D TTE or TEE for annular sizing, more recent studies have demonstrated the value of MDCT for the precise determination of annular dimensions [17–19]. The most important principle of MDCT analysis is the alignment of the basal annular plane of the three sinuses. Maximal diameters, perpendicular diameters, average diameters, area, and perimeters have all been used to determine the appropriate annular diameter (Fig. 8.4).
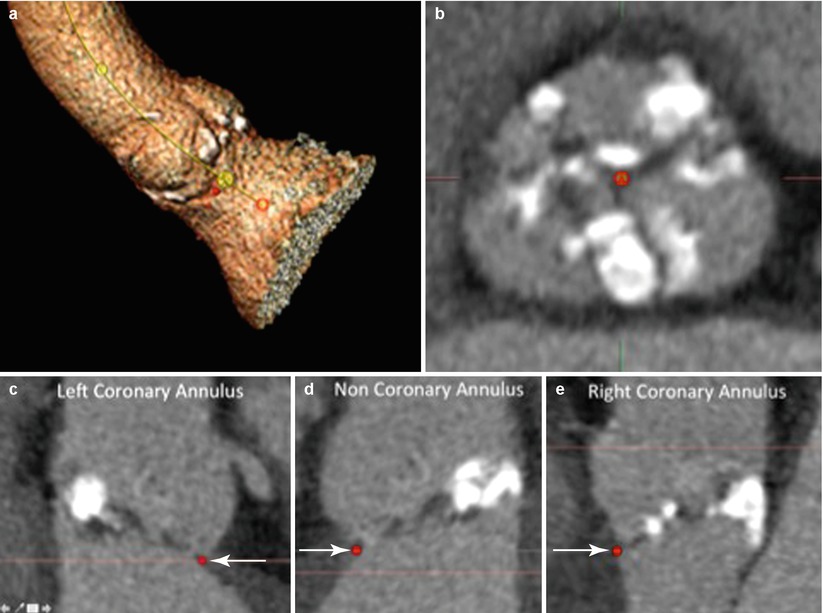
Fig. 8.4
Aortic annulus. Quantitative measure of the aortic annulus begins volume rendering of the aortovalvular complex and the creation of the aortoventricular centerline that will facilitate the creation of the double oblique (MPR) images for identification of the basal annular plane (a). The cross-sectional view of the double oblique (MPR) demonstrating the cross section of the sinus of Valsalva (b) allows identification of the basal annulus of the left coronary annulus (c, arrow), noncoronary annulus (d, arrow), and right coronary basal annulus (e, arrow). This then allows alignment of the basal annular plane of all three coronary sinuses (f). Measurements including the maximal diameter, perpendicular to the maximal diameter, area, and perimeter measurements (g)
A number of series have compared TTE, TEE, MDCT, and contrast angiography for the assessment of aortic annular dimensions [14–16, 20–25] (Table 8.3). In general, MDCT provides larger annular measurements than echocardiography. In a series of 45 consecutive patients with severe aortic stenosis referred for TAVR, correlations between TTE, TEE, and MDCT were good but the difference between MDCT and TTE (1.22 ± 1.3 mm) or TEE (1.52 ± 1.1 mm) was larger than the difference between TTE and TEE (0.6 ± 0.8 mm); p = 0.03 and p < 0.0001, respectively [23]. A decision based on MDCT measurements would have modified the TAVR strategy in a large number of patients (40–42 %) [23]. Cardiac MR (CMR) has also been used to estimate the annular dimensions [20]. In a series of 202 consecutive patients assessed by CMR, MDCT, and TTE for TAVR, close agreement was observed between CMR and MDCT in the dimensions of the aortic annulus and similarly for sinus of Valsalva, sinotubular junction, and ascending aortic measures [20]. Agreement between TTE-derived measures and either CMR or MDCT was less precise [20]. Intraobserver and interobserver variabilities were lowest with CMR [20].
The benefit of THV sizing based on MDCT and CMR (compared with TEE or TTE) has been shown in several studies [20]. In a series of 109 patients who underwent MDCT prior to balloon-expandable SAPIEN stent placement, appropriate sizing of the aortic annulus using mean diameters, area, and circumference correlated with the development of perivalvular regurgitation [26]. MDCT was repeated after TAVR to assess THV eccentricity (1 – (short diameter/long diameter)) and expansion (MDCT measured THV area/nominal THV area). Moderate or severe paravalvular aortic regurgitation was associated with TAVR undersizing [26]. The difference between THV size and MDCT annular size was predictive of PAR. The area under the curve (AUC) was 0.81 for the mean diameter, 0.80 for area, and 0.76 for circumference [26]. Annular eccentricity was not associated with PAR [26]. In this series, 35.3 and 45.1 % of aortic THVs were undersized relative to the MDCT mean diameter and area, respectively [26]. In another series, the presence and severity of AR after TAVR were associated with larger aortic valve annulus measurements by both CMR (p = 0.03) and MDCT (p = 0.04) but not TTE-derived measures (p = 0.10) [20]. In patients undergoing imaging assessment for TAVR, the presence and severity of AR after TAVR were associated with larger aortic annulus measurements by both CMR and MDCT (but not TTE) [20].
A number of parameters can be used to estimate the annular diameter (Table 8.4). In a series of 75 patients undergoing MDCT, the minimal and maximal annular diameter differed by 6.5 mm (5.7–7.2), p < 0.001] indicating an elliptical shape of the annulus in the majority of cases [19]. Estimation of the annular diameter using area and perimeter measurements seemed to be associated with better outcomes in these patients (Table 8.5) [19]. In another series of 136 patients, the discriminatory value of multiple CT annular measures for post-TAVR perivalvular aortic regurgitation was compared with 2D echocardiographic measures [27]. In receiver-operating characteristic models, cross-sectional MDCT parameters had the highest discriminatory value for post-TAVR perivalvular regurgitation [27]. The area under the curve was 0.82 for maximal cross-sectional diameter minus prosthesis size (p < 0.001) and 0.81 for circumference-derived cross-sectional diameter minus prosthesis size (p < 0.001) [27]. In contrast, traditional echocardiographic measures were nondiscriminatory in relation to post-TAVR PV aortic regurgitation [27]. In an analysis of 46 patients with MDCT, the aortic annulus plane was reconstructed at 10 % increments over the cardiac cycle [28]. In both subjects with normal and with calcified aortic valves, minimum diameter increased in systole (12.3 ± 7.3 % and 9.8 ± 3.4 %, respectively; p < 0.001), and ellipticity index decreased (12.7 ± 8.8 % and 10.3 ± 2.7 %, respectively; p < 0.001) [28]. The cross-sectional area (CSA) increased by 11.2 ± 5.4 % and 6.2 ± 4.8 %, respectively (p < 0.001) [28]. Perimeter increase was negligible in patients with calcified valves (0.56±0.85 %; p < 0.001) [28]. Relative percentage differences between maximum and minimum values were significantly smallest for perimeter compared with all other parameters [28]. The aortic annulus, generally elliptical, assumes a more round shape in systole, thus increasing CSA without substantial change in perimeter. Aortic annulus perimeter appears therefore ideally suited for accurate sizing in patients undergoing TAVR [28]. 3D TEE reconstructions of the aortic annulus may also provide better correlations with MDCT than 2D TTE or TEE alone (Fig. 8.5).
Table 8.4
Comparative studies of imaging modalities for the aortovalvular complex in patients with aortic stenosis
Author |
Number of patients |
Modalities |
Structures analyzed |
Findings |
---|---|---|---|---|
Altiok et al. [14] |
49 |
2D TTE
2D TEE
3D TEE
Contrast angiography
MDCT |
Annulus
Distance from annulus to left main |
Sagittal diameters determined by angiography, TTE, 2D TEE, 3D TEE, and MDCT were smaller than coronal diameters determined by angiography, 3D TEE, and MDCT |
Coronal and sagittal diameters determined by 3D TEE were in high agreement with corresponding measurements by MDCT (23.60 ± 1.89 vs 23.46 ± 2.07 mm and 22.19 ± 1.96 vs 22.27 ± 2.01 mm, respectively; mean ± SD) | ||||
There was a high correlation between MDCT and 3D TEE for the definition of coronal and sagittal aortic annulus diameters (r = 0.88, SEE = 0.89 mm and r = 0.77, SEE = 1.26 mm, respectively) | ||||
Correlation of 3D TEE (13.47±1.67 mm) and DSCT (13.64±1.82 mm) in the analysis of the distance between aortic annulus and left main coronary artery ostium was better (r = 0.54, SEE = 1.55 mm) than between angiography (14.85 ± 3.84 mm) and MDCT (r = 0.35, SEE = 1.77 mm) | ||||
Dashkevich et al. [15] |
33 |
2D TEE
MDCT
Hager Dilator |
Annulus |
Calculated average diameter by MDCT correlated significantly with intraoperative measurements (r = 0.923, p < 0.001) and with the size of implanted valve (r = 0.867, p < 0.001), while correlation of TEE and intraoperative measurements was weak (r = 0.523, p = 0.002)
![]() Stay updated, free articles. Join our Telegram channel![]() Full access? Get Clinical Tree![]() ![]() ![]() |