Jonathan J. James, A. Robin M. Wilson, Andrew J. Evans
The Breast
Breast cancer is the most common malignant tumour in the UK with over 48,000 diagnoses annually—80% of cases are in women over the age of 50. It accounts for over 12,000 deaths per annum. Imaging is essential for the early detection and accurate diagnosis of breast cancer. Population screening with mammography aims to reduce mortality by detecting the disease at an earlier stage, before it has spread beyond the breast. Mammography and ultrasound are the first-line imaging investigations in women with breast symptoms. Magnetic resonance imaging (MRI) is established as an adjunctive diagnostic tool because of its high sensitivity for invasive breast cancer. Percutaneous image-guided breast biopsy is used for the pathological assessment of breast lesions. The combination of imaging, clinical examination and needle biopsy—known as ‘triple assessment’—is the expected standard for breast diagnosis.
Methods of Examination
Mammography
Mammography remains one of the principal imaging modalities for diagnosis, although its use is rarely indicated in women under the age of 35. The main indications for mammography are:
• follow-up of patients with previously treated breast cancer; and
• guidance for biopsy, or localisation of lesions not visible on ultrasound.
Mammography places stringent demands on equipment and image quality. The breast is composed predominantly of fatty tissue and has a relatively narrow range of inherent densities. Consequently, special X-ray tubes are required to produce the low-energy radiation necessary to achieve high tissue contrast, enabling the demonstration of small changes in breast density. High spatial resolution is required to identify tiny structures within the breast, such as microcalcifications measuring in the order of 100 µm; and short exposure times are necessary to limit movement unsharpness. Where the breasts are thicker or are composed of denser glandular tissue, higher energy radiation is required, although radiation dose must be kept to a minimum.
X-ray tubes produce a spectrum of radiation energies, which are determined by the target and filter combination and the peak kilovoltage (kVp). A molybdenum target is used because it produces a low-energy spectrum with peaks of 17.5 and 19.6 keV, providing high contrast. A tungsten target is less desirable because it produces higher energies (Fig. 69-1). The spectrum is refined further by adding a filter to reduce the proportion of radiation above and below the desired range. Commercially available target/filter combinations include molybdenum/molybdenum, molybdenum/rhodium, rhodium/rhodium, tungsten/molybdenum and tungsten/rhodium. Molybdenum/molybdenum is the most frequently used combination.
To achieve the required spatial resolution, mammography tubes must have an extremely small focal spot, 0.3 mm for routine mammography. For magnification mammography a smaller focal spot of 0.1 mm is required. Tube current should be as high as possible in order to keep exposure times short. Movement unsharpness may occur when exposure times exceed 1 second. Grids are used routinely for all mammographic studies. These reduce scattered radiation and so increase contrast, especially in the dense or thick breast. Modern mammography machines have a facility for automatic selection of target/filter combination, kVp and tube current according to the breast density and the thickness of the compressed breast. In addition, automatic exposure control devices detect the amount of radiation striking the detector and terminate the exposure at a preset level.
Standard Projections
There are two standard mammographic projections: a mediolateral oblique (MLO) view and a craniocaudal (CC) view (Fig. 69-2). Correct positioning is crucial to avoid missing lesions situated at the margins of the breast. The MLO view is taken with the X-ray beam directed from superomedial to inferolateral, usually at an angle of 30–60°, with compression applied obliquely across the chest wall, perpendicular to the long axis of the pectoralis major muscle (Fig. 69-3A). The MLO projection is the only projection in which all the breast tissue can be demonstrated on a single image. A well-positioned MLO view should demonstrate the inframammary angle, the nipple in profile, and the nipple positioned at the level of the lower border of the pectoralis major, with the muscle across the posterior border of the film at an angle of 25°–30° to the vertical (Fig. 69-2A).
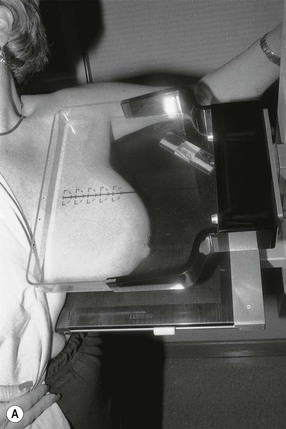
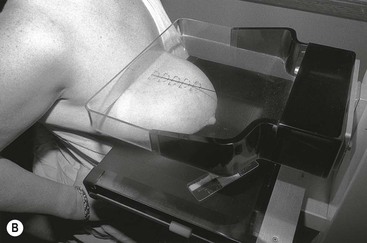
For the CC view, the X-ray beam travels from superior to inferior. Positioning is achieved by pulling the breast up and forward away from the chest wall, with compression applied from above (Fig. 69-3B). A well-positioned CC view should demonstrate the nipple in profile. It should demonstrate virtually all of the medial tissue and most of the lateral tissue except the axillary tail of the breast. The pectoralis major is demonstrated at the centre of a CC film in approximately 30% of individuals and the depth of breast tissue demonstrated should be within 1 cm of the distance from the nipple to the pectoralis major on the MLO projection (Fig. 69-2B).
Additional Projections
Supplementary views may be taken to solve specific diagnostic problems.1,2 For example, the CC view can be rotated to visualise either more of the lateral or medial aspect of the breast, compared to the standard CC projection. Localised compression or ‘paddle views’ can be performed. This involves the application of more vigorous compression to a localised area using a compression paddle (Fig. 69-4). These views are used to distinguish real lesions from superimposition of normal tissues and to define the margins of a mass. A true lateral view may be used to provide a third imaging plane in order to distinguish superimposition of normal structures from real lesions or to increase the accuracy of wire localisations of non-palpable lesions. The true lateral view is performed with the mammography unit turned through 90° and a mediolateral or lateromedial X-ray beam.
Magnification views are most frequently performed to examine areas of microcalcifications within the breast, to characterise them and to establish their extent. Magnification views are typically performed in the craniocaudal and lateral projections. The magnified lateral view will demonstrate ‘teacups’ typical of benign microcalcifications, described later in the chapter. Mammographic technique may need to be modified in women with breast implants. Silicon and saline implants are radio-opaque and may obscure much of the breast tissue. Consequently, mammography is of limited diagnostic value in some women. The Eklund technique can be employed to displace the implant posteriorly, behind the compression plate, maximising the volume of breast tissue that is compressed and imaged.3 Mammography-induced implant rupture has not been reported to date.
Breast Compression
Compression of the breast is essential for good mammography, for the following reasons:
• It reduces geometric unsharpness by bringing the object closer to the film.
• It improves contrast by reducing scatter.
• It diminishes movement unsharpness by permitting shorter exposure times and immobilising the breast.
• It separates superimposed breast tissues so that lesions are better seen.
Radiation Dose
Mammography uses ionising radiation to image the breast. The risks of ionising radiation are well known and any exposure needs to be justified, with doses kept as low as possible. The radiation dose for a standard two-view examination of both breasts is approximately 4.5 mGy.4 The average effective dose of radiation from mammography is equivalent to 61 days of average natural background radiation.5
Dose is more of an issue in a population screening programme, where women who may never develop breast cancer are being exposed to radiation. It has been estimated that the risk of inducing a breast cancer in women screened in the United Kingdom National Health Service Breast Screening Programme (NHSBSP) is 1 in 100,000 per mGy. A risk–benefit calculation has established that the benefits of screening far outweigh the risk of inducing a cancer, with the ratio of lives saved to lives lost calculated as approximately 100 : 1.4
Digital mammography systems have the potential to reduce patient dose without loss of image quality.
The Detector
Traditionally, the mammographic image has been recorded on film, but this has been superseded by digital technology. Manufacturers have developed a number of different approaches to producing a digital mammogram. The first type of digital system developed for mammography used photostimulable phosphor computed radiography (CR). This uses an imaging plate coated with a phosphor to replace the traditional screen/film mammography cassette. The imaging plate, stored in a conventional-looking cassette, is exposed in the usual fashion in a conventional (analog) mammography machine. A latent image is stored in the phosphor after exposure. The imaging plate is scanned by a laser beam in a plate reader and light is emitted in proportion to the absorbed X-rays. The emitted light is then detected by a photomultiplier system and the resulting electrical signal is digitised to produce the image.
More recently, full-field flat-panel detectors have been developed. One type of detector consists of a phosphor layer coated onto a light-sensitive thin-film transistor (TFT) array composed of amorphous silicon. Charge from the TFT array produced in response to the light emission from the phosphor is measured and digitised. The above digital systems require multiple conversion steps in the acquisition of the image: X-ray energy is converted into light energy, which is then converted into electrical energy. Multiple conversion steps are inefficient and have the potential to degrade image quality. Systems that avoid these conversion losses are described as being more ‘direct’. Some manufacturers use amorphous selenium or silicon dioxide in the detector, allowing the energy of the X-ray photon to be directly converted into electrical energy.
Digital Mammography in Clinical Practice
There are clear logistical advantages to digital mammography, including the potential to improve patient throughput, as traditional screen/film mammography is labour intensive, with time taken in handling cassettes, loading/unloading film and processing. Digital mammography equipment interfaces directly with picture archiving and communication systems (PACS), leading to further increased efficiencies associated with image storage and display, with soft-copy reporting from high-resolution (5 megapixel) monitors.
It is important to establish whether an improvement in image quality can translate into an improvement in cancer detection. A powerful test of the potential of digital mammography to improve cancer detection rates is in a screening setting. Several early studies found that digital mammography was at least equivalent to screen/film mammography in terms of cancer detection rates.6,7 The larger Oslo II study, which randomised over 25,000 women to either conventional or digital mammography, showed an increase in cancer detection in the women undergoing a digital mammogram, but this did not quite reach statistical significance (p = 0.053).8
To detect significant differences between the two techniques, the population size needs to be large, as the cancer detection rate in a screening population is around 6 per 1000 women screened. The North American Digital Mammographic Imaging Screening Trial (DMIST) enrolled 49,500 women.9 This study found that overall the diagnostic accuracy of digital and conventional mammography was similar. However, there were some groups of women where digital mammography outperformed screen/film mammography, showing significantly improved diagnostic accuracy. These were women under the age of 50, those with dense breast parenchyma and women who were pre- or perimenopausal. Encouragingly, it is in these groups of women that conventional screen/film mammography had shown reduced sensitivity for detecting breast cancer.
Computer-Aided Detection
Computer-aided detection (CAD) is a software system that is designed to assist the film reader by placing prompts over areas of concern, to reduce observational oversights. CAD systems are highly sensitive for detecting cancers on screening mammograms. CAD will correctly prompt around 90% of all cancers, with 86–88% of all masses and 98% of microcalcifications correctly marked. Specificity is much more of a problem with a high rate of false-positive prompts. The number of false prompts will vary according to the level of sensitivity at which the system is set; typically, there are between two and four false prompts per standard set of mammogram images.10–12
The routine use of CAD remains controversial and there is no consensus in the literature as to whether CAD improves film reader performance; despite this, CAD is used in the interpretation of screening mammograms in around 75% of cases in the United States.13 Some prospective studies of the use of CAD in the screening setting have shown a significant improvement in a single film reader’s performance when CAD software is applied, whereas others have shown no effect on cancer detection rates.12,14 A large multicentred retrospective review of the use of CAD in the interpretation of screening mammography in the United States found its use associated with a decrease in the specificity of screening, with an increase in recall rates for only a very minimal improvement in sensitivity, largely the result of a non-significant increase in the detection of ductal carcinoma in situ.13
It is difficult to extrapolate the findings of these studies to the UK breast screening programme (NHSBSP), where virtually all films are double read by two readers. Double reading is known to increase cancer detection rates by 4–14% compared to single reading, and so the question is whether one reader using CAD could produce results equivalent to double reading and whether CAD is a more cost-effective solution to recruitment problems than training non-medically qualified film readers. The Computer-Aided Detection Evaluation Trial (CADET II) was a large prospective trial of over 30,000 women that reported a comparable cancer detection rate for single reading with CAD to double reading but with a small but significant increase in recall rate.15 A further sub-analysis has suggested that at the present time CAD is not a cost-effective alternative to double reading in the NHSBSP in view of the increase in recall rates.16
Digital Breast Tomosynthesis
One of the limitations of mammography is that it produces a two-dimensional (2D) radiographic view of a three-dimensional structure and, as a consequence, a cancer may not be detected due to overlapping normal glandular tissue obscuring the presence of a tumour. Lesions may be simulated by the superimposition of normal tissue, leading to unnecessary recalls following screening mammography. These factors result in a reduction in the sensitivity and specificity of mammography. Breast tomosynthesis is an emerging digital mammographic technique where thin slices through the breast are reconstructed from multiple low-dose projections acquired at different angles of the X-ray tube. The resulting thin sections can be scrolled through by the reporting radiologist, with the potential to alleviate the effects of tissue superimposition.
The role of tomosynthesis is still being defined, and there is debate surrounding its use as an adjunct or replacement for conventional 2D digital mammography. Its role in diagnosis and screening requires clarification. It has the potential to be an additional tool, for the work-up of screen-detected abnormalities replacing traditional ‘paddle’ views.17 When used as a screening tool, studies show an improvement in specificity, with a reduction in recall rates of up to 11%; improvements in sensitivity over conventional 2D mammography are not so clear.18
Ultrasound
The main indications for ultrasound are:
• characterisation of palpable mass lesions;
• assessment of abnormalities detected on a mammogram;
• primary technique for the assessment of breast problems in younger patients; and
Breast ultrasound requires high-quality, high-resolution grey-scale imaging, using linear probes with high frequencies typically between 7.5 and 15 MHz. Higher frequencies result in greater resolution, but as the frequency increases, the ability of the ultrasound beam to penetrate to deeper breast tissue decreases. Consequently, the frequency selected has to be appropriate for the size of the breast to be examined. Parameters such as harmonics and compounding are available on modern ultrasound machines and can be applied to enhance the displayed image. Their use is subject to operator preference. Techniques such as colour flow imaging (Doppler) and elastography may also have a role in lesion characterisation.
Elastography is an ultrasound technique that can provide additional information based on tissue stiffness or hardness. The concept that malignant lesions feel firmer or stiffer than the surrounding breast tissue is well recognised from clinical palpation. There are two methods of producing an elastography image or elastogram: strain elastography, where the operator gently manually compresses the breast tissue, and shear wave elastography, where pulses are generated by the transducer producing transverse shear wave propagation through the breast tissue. The main advantage of shear wave elastography is that the technique is quantitative and highly reproducible. Information regarding stiffness can be displayed as a black and white or colour overlay onto the grey-scale image (Fig. 69-5). Features on the elastogram that can be measured include quantitative elasticity (stiffness) in kPa and size ratios relative to conventional grey-scale imaging. In general, breast cancers tend to be stiff, with benign lesions or normal tissue appearing softer (elasticity <80 kPa). Invasive breast cancers often produce areas of stiffness that are larger than the grey-scale abnormality, likely due to changes in the tumour-associated stroma. Elastography has the potential to improve the specificity of breast ultrasound for differentiating benign from malignant masses, reducing the number of benign biopsies. In a recent study, the use of shear wave elastography resulted in a significant improvement in the specificity of breast mass assessment from 61.1 to 78.5%.19
Ultrasound Technique
The patient is examined in the supine position with the ipsilateral arm placed behind the patient’s head. When imaging the outer portion of the breast it helps to turn the patient into a more oblique position. The aim is to flatten the breast tissue against the chest wall, reducing the thickness of breast tissue to be imaged. It is best to image the breast tissue in two planes perpendicular to each other. A transverse and a sagittal plane is a common combination, but some authors advocate examining the breast in a radial and anti-radial direction. The theory behind this method is that the ducts of the breast are positioned in a radial direction, running towards the nipple rather like the spokes of a bicycle wheel. Most breast cancers begin in the ducts and so tumours extending along the ductal system may be better visualised in this plane.20
Normal Anatomy
The breast lies on the chest wall on the deep pectoral fascia with the superficial pectoral fascia enveloping the breast. Suspensory ligaments—called Cooper’s ligaments—connect the two layers, providing a degree of support to the breast and giving the breast its shape (Fig. 69-6). Centrally, there is the nipple–areolar complex. Collecting ducts open onto the tip of the nipple. There are sebaceous glands within the nipple–areolar complex called Montgomery’s glands. Small raised nodular structures called Morgagni’s tubercles are distributed over the areola, representing the openings of the ducts of Montgomery’s glands onto the skin surface.21 Deep to the nipple–areolar complex, the breast is divided into 15–25 lobes, each consisting of a branching duct system leading from the collecting ducts to the terminal duct lobular units (TDLUs), the site of milk production in the lactating breast.
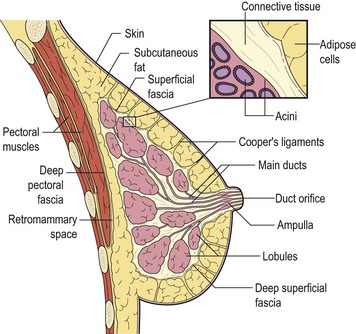
The number of TDLUs per lobe varies according to age, lactation, parity and hormonal status. At the end of reproductive life there is an increase in the amount of adipose tissue and, although the main duct system is preserved, there is considerable loss of lobular units. These changes in breast composition are manifested by changes in the breast density on mammography.
Younger women tend to have denser glandular breast tissue. In older women, the mammographic density tends to decrease, with replacement of the glandular tissue by fatty tissue. Classification systems have been developed to describe the density of breast tissue on mammography. One of the best known is the Wolfe classification:22
• Wolfe N1 refers to a breast containing a high proportion of fat;
• Wolfe P1 refers to a predominantly fatty breast with <25% visible glandular tissue;
• Wolfe P2 refers to a breast with >25% visible glandular tissue; and
Similarly, the American College of Radiology (ACR) breast imaging reporting and data system (BIRADS) lexicon defines four patterns of increasing density, where 1 is almost entirely fatty and 4 is extremely dense.23
Mammographic density is a risk factor for the development of breast cancer, with a dense background pattern associated with a higher than average risk of developing breast cancer and more aggressive tumour characteristics.24 The mechanism through which increased density contributes to breast cancer risk remains unclear. In addition, dense breast tissue may hide abnormalities in the breast, making cancer detection more difficult. The sensitivity of mammography for detecting breast cancer is directly related to the density of the breast tissue. In general, mammography is more sensitive at detecting breast cancer in older, postmenopausal women because the breast tends to be composed of greater amounts of fatty tissue.
Breast Pathology
Benign Mass Lesions
Cysts
Cysts are the most common cause of a discrete breast mass, although they are often multiple and bilateral. They are common between the ages of 20 and 50 years, with a peak incidence between 40 and 50 years. Simple cysts are not associated with an increased risk of malignancy and have no malignant potential.
On mammography they are seen as well-defined, round or oval masses (Fig. 69-7A). Sometimes a characteristic halo is visible on mammography. Ultrasound also demonstrates well-defined margins, with an oval or round shape. There is an absence of internal echoes indicating the presence of fluid. The area of breast tissue behind a cyst appears bright on ultrasound (posterior enhancement) due to improved transmission of the ultrasound beam through the cyst fluid (Fig. 69-7B). When these features are present, a cyst can be diagnosed with certainty. Aspiration is easily performed under ultrasound guidance to alleviate symptoms or when there is diagnostic uncertainty. Cytology on cyst fluid is not routinely performed unless there are atypical imaging features or the aspirate is bloodstained.
Fibroadenomas and Related Conditions
Fibroadenomas are the most common cause of a benign solid mass in the breast. They present clinically as smooth, well-demarcated, mobile lumps. They are most frequently encountered in younger women with a peak incidence in the third decade. With the advent of screening, many previously asymptomatic lesions are detected.
On mammography, fibroadenomas are seen as well-defined, rounded or oval masses (Fig. 69-8A). Coarse calcifications may develop within fibroadenomas, particularly in older women (Fig. 69-9).
Ultrasound features have been described that are characteristic of benign masses.20 These include hyperechogenicity compared with fat, an oval or well-circumscribed lobulated or gently curving shape and the presence of a thin echogenic pseudocapsule. If these features are present with no features suggestive of malignancy, then a mass can be confidently classified as benign.
These features are demonstrated by fibroadenomas (Fig. 69-8B). Most fibroadenomas are isoechoic or mildly hypoechoic relative to fat, with an oval shape and lobulated contour. A thin echogenic pseudocapsule may be seen. Percutaneous biopsy may be avoided in women under the age of 25, where the risks of any mass being malignant are very small;20 however, in most cases, even though the mass appears benign, percutaneous biopsy is undertaken to confirm the diagnosis.
Fibroadenomas must be distinguished from well-circumscribed carcinomas; this is done by percutaneous biopsy. Phyllodes tumour can also have a similar appearance to fibroadenoma, leading to diagnostic difficulties (Fig. 69-10). The pathological characteristics can also be similar to those of large fibroadenomas. Most phyllodes tumours are benign, but some (less than 25%) are locally aggressive and may even metastasise.21 When a diagnosis of phyllodes tumour is made, surgical excision must be complete with clear margins to prevent the possibility of recurrence. Many larger fibroadenomas (over 3 cm) and those that show a rapid increase in size are excised in order to avoid missing a phyllodes tumour.
Papilloma
Papillomas are benign neoplasms, arising in a duct, either centrally or peripherally within the breast. Many papillomas secrete watery material, leading to a nipple discharge. As they are often friable and bleed easily, the discharge may be bloodstained.
On mammography, they may be seen as a well-defined mass, commonly in a retroareolar location (Fig. 69-11A). Sometimes the mass is associated with microcalcifications. On ultrasound, they typically appear as a filling defect within a dilated duct or cyst (Fig. 69-11B). On aspiration, any cyst fluid may be bloodstained. As it is impossible to differentiate papillomas from papillary carcinomas on imaging criteria, percutaneous biopsy is required.
Papillomas are associated with an increased risk of malignancy, particularly if they are multiple or occur in a more peripheral location within the breast. Consequently, excision of papillary lesions is desirable and may be therapeutic in cases of nipple discharge. In situations where percutaneous biopsy shows no evidence of cellular atypia, an alternative to surgical excision is piecemeal percutaneous excision using a vacuum-assisted biopsy device.
Lipoma
Lipomas are benign tumours composed of fat. They present clinically as soft, lobulated masses. Large lipomas may be visible on mammography as a radiolucent mass (Fig. 69-12A). On ultrasound their characteristic appearance is that of a well-defined lesion, hyperechoic compared with the adjacent fat (Fig. 69-12B).
Hamartoma
Hamartomas are benign breast masses composed of lobular structures, stroma and adipose tissue—the components that make up normal breast tissue. They occur at any age. On imaging they may be indistinguishable from other benign masses, such as fibroadenomas. Sometimes large hamartomas are detected on screening mammograms and are impalpable (Fig. 69-13). On mammography they classically appear as large, well-circumscribed masses containing a mixture of dense and lucent areas, reflecting the different tissue components present. Diagnostic difficulty may be encountered because percutaneous biopsy specimens may be reported as normal breast tissue.
Invasive Carcinoma
Breast carcinomas originate in the epithelial cells that line the terminal duct lobular unit (TDLU). When malignant cells have extended across the basement membrane of the TDLU into the surrounding normal breast tissue, the carcinoma is invasive. Malignant cells contained by the basement membrane are termed non-invasive or in situ.
Classification of Invasive Breast Cancer
There is much confusion regarding the classification of breast cancer. Some tumours show distinct patterns of growth, allowing certain subtypes of breast cancer to be identified. Those with specific features are called invasive carcinoma of special type, while the remainder are considered to be of no special type (NST or ductal NST). Special-type tumours include lobular, medullary, tubular, tubular mixed, mucinous, cribriform and papillary. Different types of tumour have different clinical patterns of behaviour and prognosis. It should be understood that when a tumour is classified as of a special type this does not imply a specific cell of origin, but rather a recognisable morphological pattern.21,25
Histological grade has implications for tumour behaviour, imaging appearances and prognosis. The morphological features on which histological grade is based are tubule formation, nuclear pleomorphism and frequency of mitoses.25 Low-grade tumours that are well differentiated are less likely to metastasise.
Imaging Appearance of Invasive Breast Cancer
Mammography.
Carcinomas typically appear as ill-defined or spiculate masses on mammography (Figs. 69-14A, B). Lower-grade cancers tend to be seen as spiculate masses, due to the presence of an associated desmoplastic reaction in the adjacent stroma. Higher-grade tumours are usually seen as an ill-defined mass, but sometimes a rapidly growing tumour may appear relatively well defined, with similar appearances to a benign lesion such as a fibroadenoma (Fig. 69-14C).
Many breast cancers arise from areas of ductal carcinoma in situ (DCIS) and are associated with microcalcifications on mammography (Fig. 69-14A). This is particularly true for high-grade invasive ductal carcinomas that are often associated with high-grade DCIS.26
Special-type tumours can have particular mammographic characteristics:
• Lobular carcinomas can be difficult to perceive on a mammogram due to their tendency to diffusely infiltrate fatty tissue. Compared with ductal NST tumours, lobular cancers are more likely to be seen on only one mammographic view, are less likely to be associated with microcalcifications and are more often seen as an ill-defined mass or an area of asymmetrically dense breast tissue.27
• Tubular and cribriform cancers often present as architectural distortions or small spiculate masses.28
• Papillary, mucinous and medullary neoplasms may appear as new or enlarging multilobulated masses and may be well defined, simulating an apparently benign lesion.29,30
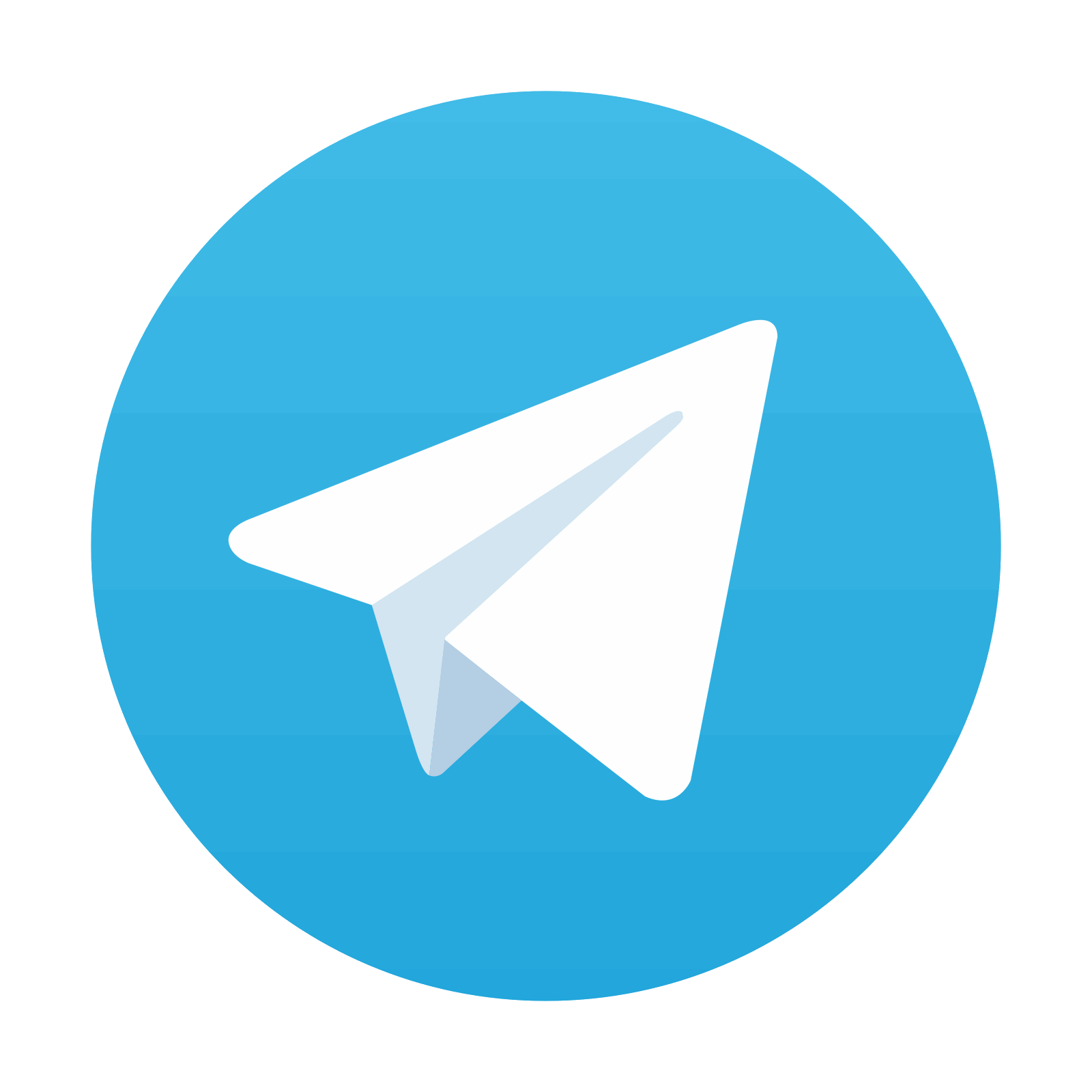
Stay updated, free articles. Join our Telegram channel

Full access? Get Clinical Tree
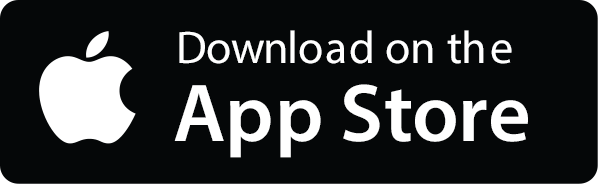
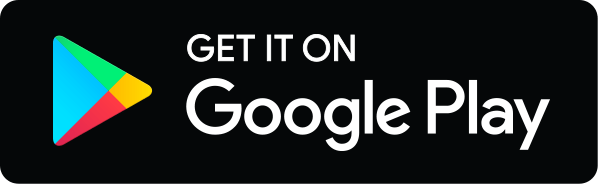