Degenerative disease of the spine is a leading cause of back pain and radiculopathy, and is a frequent indication for spine MR imaging. Disc degeneration, disc protrusion/herniation, discarhtrosis, spinal canal stenosis, and facet joint arthrosis, as well as interspinous processes arthrosis, may require an MR imaging workup. This review presents the MR imaging patterns of these diseases and describes the benefit of the MR imaging in these indications compared with the other imaging modalities like plain radiographs or computed tomography scan.
Key points
- •
MR imaging has a key role for exploration of spine degenerative disease.
- •
Intervertebral disc fissures are optimally depicted on T2-weighted imaging.
- •
Spine MR imaging should systematically be performed for cases of back pain associated with neurologic deficit.
- •
MR imaging is the best imaging modality for the exploration of spinal canal stenosis.
Introduction
Degenerative disease of the spine is a leading cause of back pain and radiculopathy, and a frequent indication for spine MR imaging. Disc degeneration, disc protrusion/herniation, discarhtrosis, spinal canal stenosis, facet joint arthrosis as well as interspinous processes arthrosis may require an MR imaging workup. This review presents the MR imaging patterns of these diseases and describes the benefits of the MR imaging in these indications compared with the other imaging modalities like plain radiographs or computed tomography (CT) scan.
Introduction
Degenerative disease of the spine is a leading cause of back pain and radiculopathy, and a frequent indication for spine MR imaging. Disc degeneration, disc protrusion/herniation, discarhtrosis, spinal canal stenosis, facet joint arthrosis as well as interspinous processes arthrosis may require an MR imaging workup. This review presents the MR imaging patterns of these diseases and describes the benefits of the MR imaging in these indications compared with the other imaging modalities like plain radiographs or computed tomography (CT) scan.
Indications for spine MR imaging/imaging protocols
In most cases, back pain does not require imaging if no neurologic deficit is observed on clinical examination. However, when pain lasts for more than 4 weeks despite analgesic medications, imaging of the spine is required. The first line imaging technique is the conventional spine radiograph or spine CT scan, except in case of neurologic deficit.
In some cases, spine MR imaging should, however, be performed:
- •
Low back pain complicated by radiculopathy (cruralgia, sciatica);
- •
Symptoms related to spinal canal stenosis;
- •
Radiculopathy with neurologic deficit; and
- •
Cauda equina syndrome.
Compared with conventional radiographs, MR imaging of the spine offers the possibility to directly visualize the intervertebral disc, as well as the nerve roots and the spinal cord.
Compared with spine CT scan, MR imaging of the spine has the advantage of being a nonirradiant examination. Additionally, it helps to see the spinal cord clearly and may help to distinguish recurrent disc protrusion versus fibrosis in a postoperative condition or to detect an inflammatory lesion or tumor disease. The major limitation of this imaging modality is that the acquisition may be long and may be hampered by motion artifacts, especially in patients who are in a good deal of pain. CT of the spine presents some other advantages compared with MR imaging, like better visualization of osteophytes, intradiscal gas (vacuum disc), and calcifications.
It is accepted commonly that a MR imaging protocol of the spine should contain at least 2 orthogonal plans. The surface coils are the most commonly used. The most widely used protocol for exploration of degenerative disease of the spine includes: sagittal T1- and T2-weighted images (WI) and sagittal (or coronal) short tau inversion recovery (STIR)-WI. The latter sequence helps in the characterization of edema of the medullary bone. Axial T2-WI acquisitions are systematically performed on the 3 to 4 last lumbar disc levels to depict potential foraminal or extraforminal disc herniation. Axial T2-WI also helps to pinpoint the precise relationship between the disc bulging/herniation and the surrounding nervous structures (nerve roots and spinal cord). For cervical spine exploration, a 3-dimensional gradient echo sequence provides a slice thickness of less than 1 mm, helping to see more precisely the intervertebral foramina and the nerve roots. CUBE T2 acquisition may also be valuable because it allows for multiplanar reconstructions, offering a better visualization of nerve roots compression. Finally, contrast media injection should only be performed postoperatively to distinguish recurrent herniation from fibrosis or to help in distinguishing a disc-free fragment from a nerve root tumor. The whole spinal cord should be explored by the MR imaging acquisition when neurologic symptoms related to spinal cord compression are observed on clinical evaluation. Indeed, compressive disc herniation may be multifocal.
Disc degeneration
The intervertebral discs are located between the endplates of adjacent vertebral bodies; they have a cushion shape and allow the mobility of one vertebral body over another. They are cartilaginous structures; their composition is divided into the nucleus pulposus in the central aspect of the disc and the annulus fibrosus at the peripheral aspect. The annulus fibrosus and the nucleus pulposus are both made of collagen (type II for the nucleus pulposus and type I for the annulus fibrosus) and proteoglycans. The nucleus pulposus is made of more than 80% of water and has a gelatinous texture. The annulus fibrosus consists of concentric rings or lamellae, with fibers in the outer lamellae continuing into the longitudinal ligaments and vertebral bodies. The collagen lamellae are denser at the peripheral aspect of the annulus. The Sharpey fibers are dense collagen fibers located on outer aspect of the annulus that attach the intervertebral disc to the adjacent endplates. The intervertebral disc is also attached ventrally and dorsally to the anterior and posterior longitudinal ligaments (PLL), respectively.
Disc changes may be related to aging or to degeneration if repeated, clinically significant mechanical constraints are exerted on the intervertebral disc. With aging, disc cells biological changes are observed, mainly cell type alteration within the nucleus pulposus, cell death, and alteration of cell phenotype compromising their ability to synthetize normal matrix components. Additionally, increased inflammatory response and enhanced catabolic metabolism leading to breakdown of the extracellular matrix are observed in the degenerated disc.
The term “disc degeneration” include the following pathomechanisms and imaging patterns: desiccation, disc space narrowing, disc bulging, disc herniation, disc tears or fissures, intradiscal gas, disc calcification, inflammatory/sclerotic changes of the vertebral endplates, and marginal osteophytes.
Progressive loss of disc water is called “desiccation” and appears on T2-WI imaging as a more or less marked reduction of the normal hyperintense signal within the intervertebral disc. This decrease in signal intensity on T2-WI is thus an early surrogate marker of intervertebral disc degeneration.
In 2001, Pfirrmann and colleagues proposed a 5-level grading scale for the evaluation of disc degeneration on T2 SE-WI ( Box 1 ).
- •
Grade I: disc is homogeneous with bright hyperintense white signal intensity and normal disc height
- •
Grade II: disc is inhomogeneous, but keeping the hyperintense white signal. Nucleus and annulus are clearly differentiated and a horizontal gray band could be present at the central aspect of the disc; eight is normal
- •
Grade III: disc is inhomogeneous with an intermittent gray signal intensity. Distinction between nucleus and annulus is unclear; disc height is normal or slightly decreased
- •
Grade IV: disc is inhomogeneous with a hypointense dark gray signal intensity. There is no more distinction between the nucleus and annulus ; disc height is slightly or moderately decreased
- •
Grade V: disc is inhomogeneous with a hypointense black signal intensity. There is no more distinction between the nucleus and annulus ; the disc space is collapsed
Before the advent of MR imaging, the reference imaging modality to visualize spinal disc degeneration was the discography, which consists of the puncture of the intervertebral disc with a needle under fluoroscopic guidance and subsequent injection of contrast material. In the mid 1980s, MR imaging has been shown to be a valuable noninvasive imaging technique to depict disc degeneration, especially for early signs of degeneration like intradiscal fissures. Yu and colleagues have described 3 patterns of intradiscal fissures related to spinal disc degeneration on T2-WI ( Fig. 1 ).
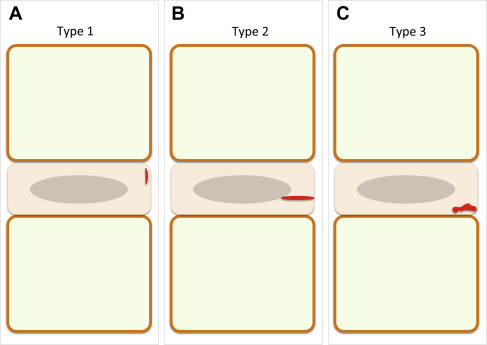
Annular fissure is a subtype of disc fissure located within the annulus fibrosus. It appears on T2-WI as a focal high intensity zone within the annulus (most commonly in its peripheral aspect). According to a recent recommendation for lumbar disc degeneration nomenclature, the term “fissure” should be used instead of “tear” because tear has a connotation of injury.
When disc degeneration is evolved, it may lead to disc bulging or even herniation when the disc loses its cohesion and its mechanical properties. Sometimes, major disc degeneration may appear as intradiscal gas (most frequently nitrogen) or may lead to disc calcification (appearing in both conditions as hypointense signals on T1-WI and T2-WI).
Disc herniation
Disc protrusion or extrusion consists of focal bulging of disc material (nucleus, annulus, and/or material from the endplate). Disc protrusion or extrusion has the same signal as the intervertebral disc. Sometimes, the protrusion or herniation may appear with a hyperintense signal on T2-WI. Peripheral enhancement may be seen after contrast media injection, especially in the lumbar region, and may be related to inflammatory processes, neovascularization, or both ( Fig. 2 ). This inflammation-related enhancement should be distinguished from the epidural venous plexus. This inflammatory process may help to resorb spontaneously the disc herniation.
The terminology for intervertebral disc bulging is very muddled. The most widely used nomenclature is as follows ( Fig. 3 ) :
- •
Disc bulging : circumferential (symmetric or asymmetric) disc enlargement.
- •
Disc herniation : disc protrusion or extrusion leading to a focal bulging, usually 3 mm or greater, beyond the vertebral margin.
- •
Disc protrusion : eccentric herniation with a broad-based connection with disc (the greatest distance, in any plane, between the edges of the disc material beyond the disc space is less than the distance between the edges of the base in the same plane).
- •
Disc extrusion : eccentric disc bulging with a narrowed connection to the disc.
- •
Free fragment : epidural fragment of disc inside the vertebral canal with no connection to the intervertebral disc. This pattern may also be called “ sequestration .” The free fragment is located more frequently at the anterior aspect of the spinal canal, but may also be observed at the lateral or even posterior aspect of the canal ( Fig. 4 ). A free fragment in the intradural compartment may be observed rarely, when the disc fragment has ruptured through the thecal sac.
Fig. 4
Lumbar MR imaging. Sagittal T2-weighted imaging (WI); ( A ) and T1-WI ( B ). Moderate L4-L5, and marked L5-S1 disc degeneration ( asterisks ) are seen. ( C ) Axial T2-WI at L5-S1 level. Posterior right L5-S1 disc bulging ( C , arrow heads) associated with a posterior free fragment (sequestration; white arrows ).
- •
Migration : disc herniation with upward or downward migration compared with the disc’s plane ( Fig. 5 ).
Fig. 5
Lumbar MR imaging. Sagittal T2 ( A ), coronal short tau inversion recovery-weighted imaging (WI); ( B ) and axial T2-WI ( C ) showing an L4-L5 upward migrated disc herniation ( arrows ).
- •
Contained disc herniation : the displaced part of the disc is covered by the outer fibers of the annulus fibrosus and/or by the PLL. This pattern of herniation is also called “subligamentous herniation.”
- •
Uncontained disc herniation : the herniated fragment is neither covered by the peripheral aspect of the annulus nor by the PLL.
- •
Transligamentous disc herniation : disc extrusion through the PLL.
- •
Transmembranous disc herniation : disc extrusion through the peridural membrane.
Most of the disc herniations are located at the lower lumbar spine (L4-L5 and L5-S1). Indeed, the 2 above-mentioned levels account for approximately 90% of disc herniations. On the cervical spine, disc degenerations are usually located at its inferior aspect (ie, C5-C6 and C6-C7 levels). It is noteworthy that thoracic spine disc herniations are rare (approximately 1% ) owing to specific anatomic factors like the low weight of the thoracic intervertebral disc. Disc fragment calcifications are more frequent in the thoracic region than in the cervical or lumbar spine ( Fig. 6 ).
Disc bulging or herniation may be responsible for nerve root compression either in the spinal canal (at its lateral aspect: the lateral recess; Fig. 7 A ), in the intervertebral foramen ( Fig. 7 B), or after the exit of the nerve root from the intervertebral foramen (ie, the area lateral to the facet joint). The risk of nerve root compression by disc herniation may be increased by a hypertrophy of the adjacent facet joint, particularly the superior articular process. Nerve root impingement may appear as a simple contact between the disc and the nerve root with loss of the periradicular cerebrospinal fluid (CSF) signal and/or disappearance of the anterior epidural fat’s hyperintense signal. In some cases, the nerve root may be pushed back by the herniated disc or even not visible anymore. Increasing size of the nerve root as well as nerve root enhancement (related to breakdown of the blood–nerve barrier and to changes in capillary permeability of the nerve root ) are radiological signs of nerve root suffering in case of compressive disc herniation. Rarely, an osteophyte may be responsible for nerve root compression. Disc herniation or bulging may also be responsible for spinal cord irritation or compression or for cauda equina syndrome in case of major disc herniation below the conus medullaris ( Fig. 8 ).
The pathomechanisms involved in radiculopathy may be a direct compression of the nerve root by the herniated disc and/or related to inflammatory reaction around the herniated disc, leading to an irritation of the nerve root.
Disc degeneration is associated in more than one-half of the cases with changes of the vertebral endplates adjacent to the diseased disc.
The intervertebral disc participates for the congruency of a true articulation between the endplates of 2 adjacent vertebral bodies. As for other joints of the body with arthrosis, degeneration of the cartilaginous endplates (fissures) leading to edematous reaction of the subchondral bone marrow may be observed in disc degenerative disease. In 1988, Modic and colleagues detailed the subchondral bone marrow changes on MR imaging acquisitions in discarthrosis, and have subdivided the signal changes into 3 patterns ( Figs. 9 and 10 ).
Modic Type 1
Modic type 1 changes includes an inflammatory reaction with bone marrow edema and vascular congestion. It appears as a hypointense signal on T1-WI and hyperintense signal on T2-WI and STIR-WI. Enhancement is observed commonly after gadolinium injection. Sometimes, Modic’s type 1 inflammatory changes may be so pronounced that they may mimic a spondylodiscitis. However, when increased disc signal intensity on T2-WI and/or paravertebral or epidural abscess are seen, the more likely diagnosis is an infectious spondylitis rather than Modic type 1 degeneration.
Modic Type 2
Modic type 2 inflammation entails subchondral fatty conversion of the bone marrow. These changes appear as a hyperintense signal on both T1-WI and T2-WI, and hypointense on STIR-WI. Type 2 changes may be observed as frequently as in 20% of the cases in patients greater than 50 years of age, and are usually located in the anterior one-third of the vertebral endplate.
Modic Type 3
Modic type 3 changes include sclerotic changes of the subchondral bone marrow. They appear as hypointense signal on T1-WI, T2-WI, and STIR-WI.
Evolution from Modic’s type 1 to type 3 usually takes a few years of time. Some congenital vertebral anomalies, like lumbosacral transitional vertebrae, may favor disc degeneration.
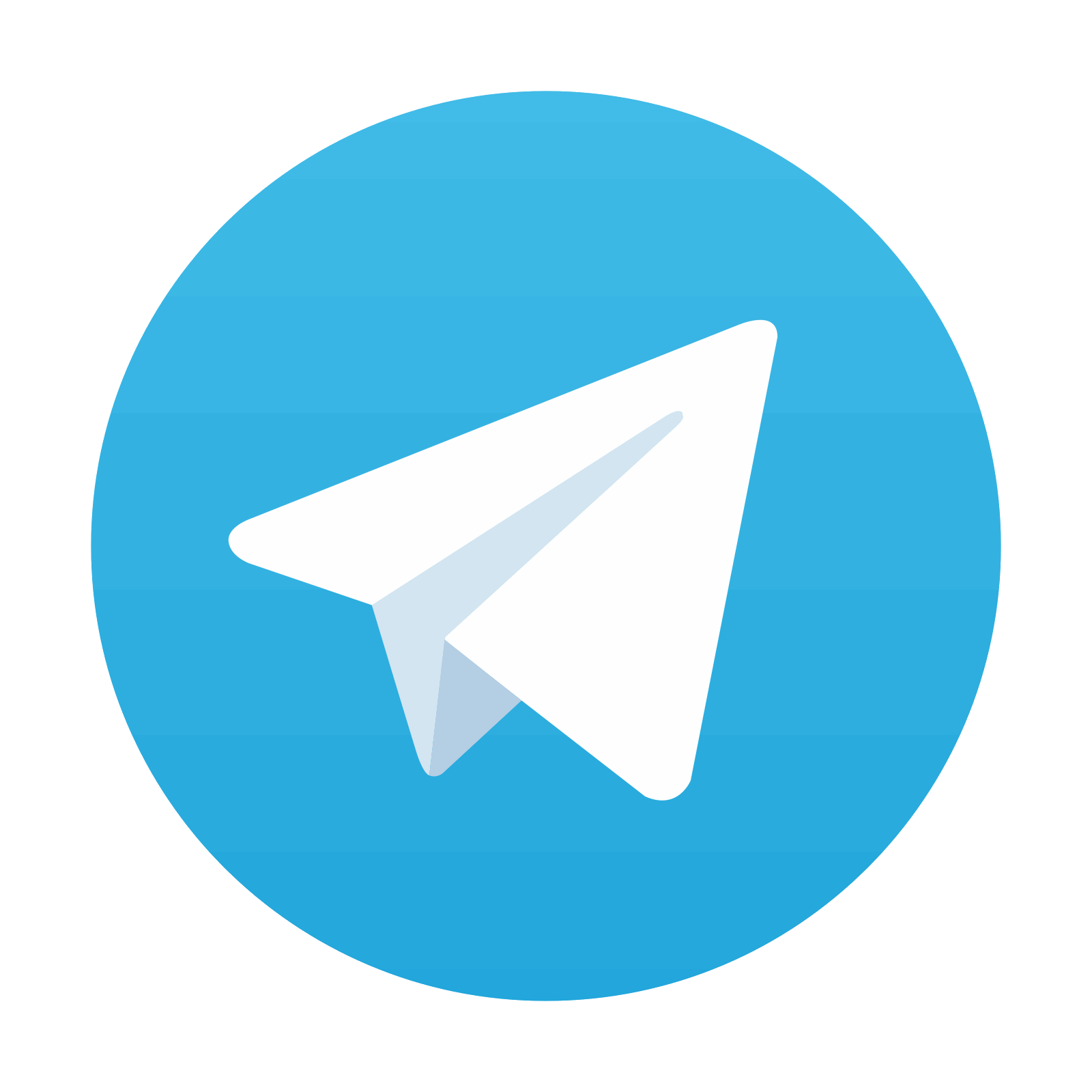
Stay updated, free articles. Join our Telegram channel

Full access? Get Clinical Tree
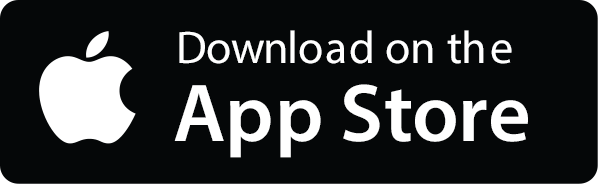
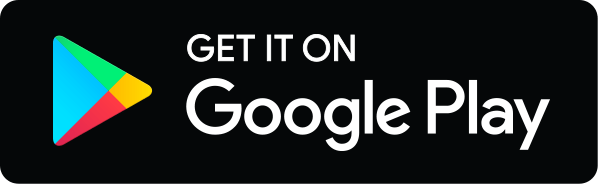