Fig. 8.1
(a, b) Six ossification centers of the distal humerus. C capitulum, R radial head, M medial epicondyle, O olecranon, T trochlea, L lateral epicondyle
Box 8.1: Typical Order of Ossification at the Elbow
C—capitellum |
R—radial head |
I—internal, or medial, epicondyle |
T—trochlea |
O—olecranon |
E—external, or lateral, epicondyle |
Bone maturation is affected by genetic and racial variability as well as environmental factors and pathological processes that can affect bone growth and maturation [1, 2]. There is no significant difference in timing or ossification pattern between the right and left elbow [3]. However, the timing of appearance of the ossification centers varies widely (Table 8.1). All except the capitellum appear and fuse at least 1 year earlier in girls than in boys [1]. The capitellum ossifies at about 3 months in girls and 6 months in boys. The approximate age of ossification for the five other centers in girls is 5 years for the radial head, 5 years for the medial epicondyle, 8–9 years for the trochlea, 8 years for the olecranon, and 9 years for the lateral epicondyle. In boys, the radial head ossification center appears at approximately 5–6 years, medial epicondyle at 7 years, trochlea at 9 years, olecranon at 10 years, and lateral epicondyle at 11 years. In general, the centers that ossify after the radial head appear about 2 years later in boys than in girls [1, 3–5].
Table 8.1
Reported ranges of appearance and fusion of ossification centers about the elbow
Girls—age of appearance | Girls—age of fusion | Boys—age of appearance | Boys—age of fusion | |
---|---|---|---|---|
Capitellum | 0.33–1 | 9.4–15 | 0.08–1 | 12.4–17.5 |
Radial head | 1.6–6.8 | 10.2–15 | 2.9–8.5 | 12.4–17.1 |
Medial epicondyle | 1.3–7.1 | 11.5–15.3 | 3.5–9.7 | 13–16.8 |
Trochlea | 5.7–11.1 | 9.2–13 | 7–12.5 | 11.6–15.2 |
Olecranon | 6–11 | 10.8–14.8 | 7.6–12.2 | 12.4–16.5 |
Lateral epicondyle | 7.3–11.6 | 9.5–13.3 | 8–14.4 | 12.1–15.9 |
The range of age of fusion of the elbow ossification centers is listed in Table 8.1. In general, girls fuse earlier than boys.
2 Distal Humeral Fractures
2.1 Supracondylar Fractures (Box 8.2)
Box 8.2: Supracondylar Fractures
Most common pediatric elbow fracture, age 5–9 |
Usually extension injury → posterior displacement of capitellum |
Normally anterior humeral line should intersect posterior or middle one third capitellum |
Disrupted hourglass or figure eight |
Supracondylar fractures are the most common pediatric elbow fracture. The peak incidence is between 5 and 9 years of age, and boys are affected twice as often as girls [6]. The left elbow is affected more often than the right. These fractures usually result from a fall such as from a chair, crib, or playground equipment, and most are caused by hyperextension when the child falls on the outstretched extremity [7].
Supracondylar fractures are categorized according to mechanism of injury, which is determined by assessing the angulation of the distal fragment. The vast majority (95 %) result from extension injury. Only 5 % result from flexion, and these occur predominantly in older children. With the extension type of fracture, the capitellum is posteriorly displaced. Many systems have been developed for classifying these fractures. The Gartland system employs three categories, based on the degree of displacement [8]:
Type 1: Nondisplaced or minimally displaced fracture (hairline fracture) (Fig. 8.2).
Fig. 8.2
Gartland type I minimally displaced supracondylar fracture. (a, b) There is subtle lucency, most obvious at the lateral supracondylar region (arrow). Alignment is normal
Type 2: Partially displaced (posterior cortical hinge is intact (in extension-type fractures), fragments are nearly aligned, and some bony contact is present) (Fig. 8.3).
Fig. 8.3
Gartland Type II partially displaced supracondylar fracture. (a, b) The fracture line is more apparent at the lateral condyle. There is mild posterior displacement of the capitellum, but some bony contact is maintained
Type 3: Completely displaced (no posterior cortical hinge (in extension-type fractures); fracture fragments are widely separated) (Fig. 8.4).
Fig. 8.4
Gartland Type III completely displaced supracondylar fracture. (a, b) The fracture is complete, and the distal fragment displaced by at least one bone width posteriorly. There is no contact between the fragments
Numerous newer classification systems have been developed, but they offer neither more reproducibility nor better interobserver validity.
Compartment syndrome may develop at the flexor compartment if there is direct laceration or interruption, spasm, or intimal tear of the brachial artery [6] or even in the absence of these complications. The median and radial nerves are at risk for injury in an extension-type fracture, while the ulnar nerve is more vulnerable in a flexion-type fracture. These injuries are more likely with a Gartland Type 3 displaced fracture.
The degree of displacement dictates the type of treatment. Type 1 Gartland extension fractures are immobilized without manipulation. Type 2 and Type 3 fractures require operative treatment. Closed reduction is attempted, stabilized by at least two crossed stainless steel Kirschner wires (K-wires). The conservative approach is less successful with the uncommon flexion fractures, as they are less stable.
Imaging
High-quality radiographic technique is essential for successful detection of subtle elbow fractures in children. The minimum standard examination for evaluating the injured elbow consists of a frontal view of the straight elbow and a lateral view of the flexed elbow [7]. Careful assessment of the soft tissues around the elbow joint can provide helpful clues about the presence and location of a fracture.
The vast majority of elbow fractures are accompanied by fluid within the elbow joint. An elbow effusion is easily detected on a well-positioned lateral view due to displacement of the anterior and posterior fat pads of the distal humerus. In the normal elbow, the anterior fat pad is partially visible extending from the coronoid fossa, but the posterior fat pad is hidden within the deeper olecranon fossa (Fig. 8.5). As fluid accumulates within the joint space, it displaces these fat pads upward and outward [7, 9, 10]. In the absence of a positive fat pad, significant intra-articular injury is unlikely but not impossible, as a capsular tear may allow fluid to leak into the surrounding soft tissues, preventing distension of the joint capsule (Fig. 8.6). A visible fat pad sign without a demonstrable fracture often (but does not absolutely [11]) indicates an occult fracture in the setting of trauma.
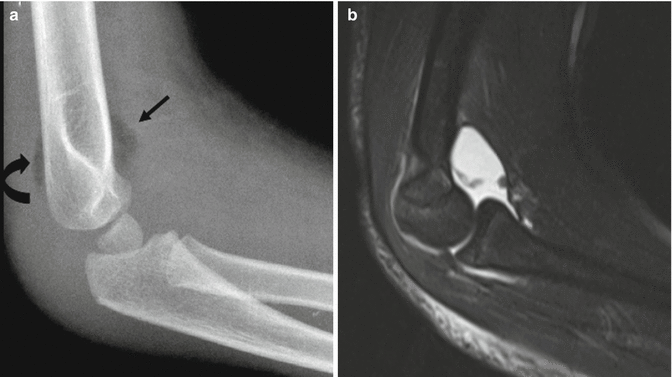
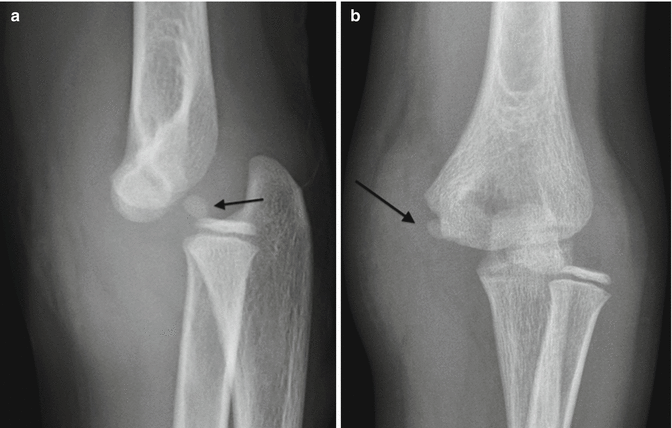
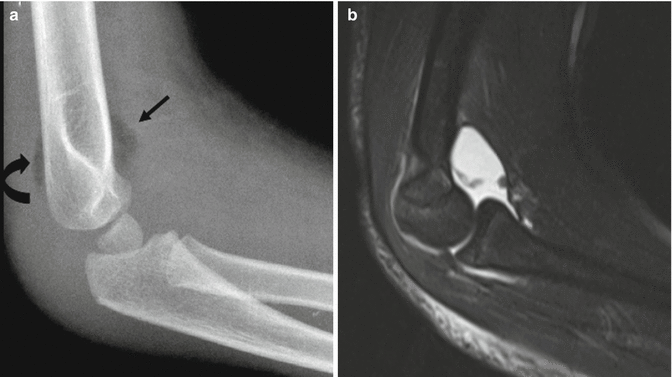
Fig. 8.5
Joint effusion. (a) The displaced anterior (arrow) and posterior (curved arrow) fat pads appear as lucent triangles extending from the bone margins. (b) T2-weighted (T2-W) fat-suppressed (FS) MRI shows hyperintense joint fluid elevating the soft tissues anterior to the joint
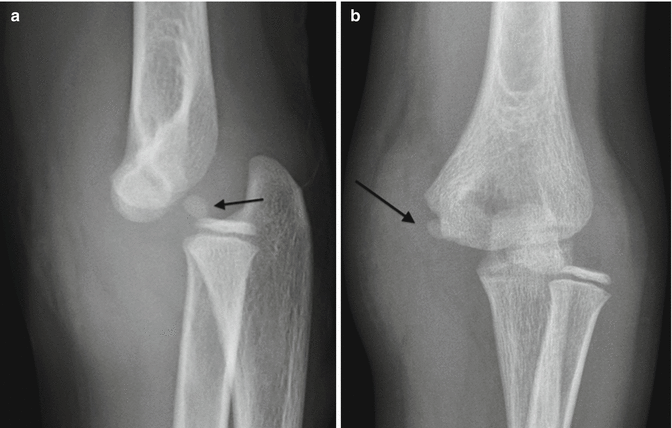
Fig. 8.6
Absence of a joint effusion despite severe injury. (a, b) The medial epicondyle is fractured and displaced (arrow), and the olecranon and radial head are also dislocated. Despite this severe trauma, there is no joint effusion. Increased density in the soft tissues probably results from extracapsular joint fluid due to capsular tear (Courtesy of Rebecca Stein-Wexler)
The anterior humeral line helps identify slight capitellar displacement, which in turn suggests a subtle distal humeral fracture may be present. This line is drawn along the anterior cortex of the humeral diaphysis on a well-positioned lateral view [7] and should intersect the posterior or middle third of the capitellum. If it crosses the capitellum more anteriorly, a supracondylar fracture is probably present (Fig. 8.7). The hourglass (or figure eight) sign is also useful (Fig. 8.8). Normal overlapping contours create an intact hourglass on the lateral view. In the presence of a supracondylar fracture, the hourglass may appear broken. However, in the absence of severe force, a supracondylar buckle or plastic bending fracture may occur, and these signs may be normal [7].
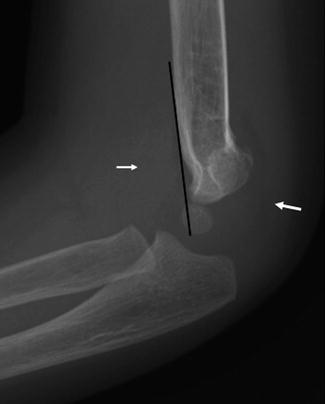
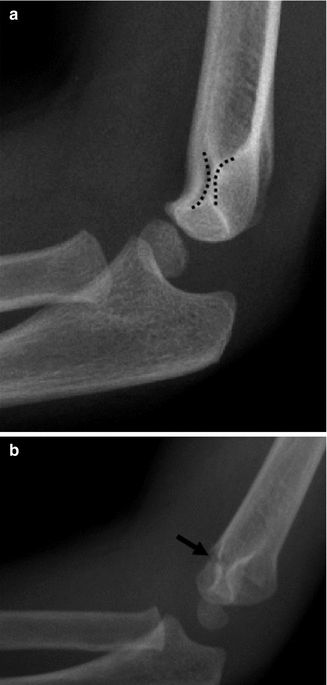
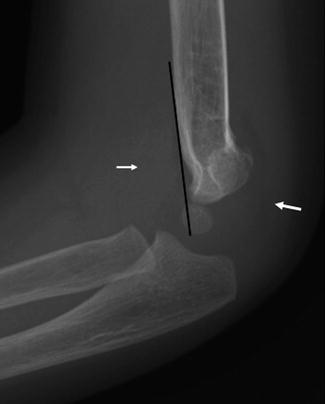
Fig. 8.7
Anterior humeral line with mildly displaced supracondylar fracture. The anterior humeral line intersects the anterior third of the capitellum, rather than the middle or posterior third. In addition, there is a large joint effusion, elevating both the posterior and anterior fat pads (arrows)
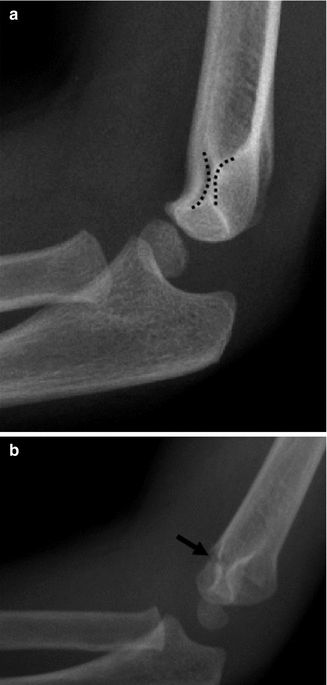
Fig. 8.8
Supracondylar fracture with broken hourglass sign (or figure eight). (a) Normal contralateral elbow for comparison shows intact hourglass. (b) Interrupted hourglass indicates supracondylar fracture, which in this case is also angulated (arrow)
Ultrasound (US) is very sensitive for detecting joint effusions, which are best depicted by examining the olecranon fossa in the sagittal plane from a posterior approach (Fig. 8.9). Under normal conditions, only a small amount of fluid can be identified between the fat pad and the humerus. US can sometimes detect a fracture as an interruption of the cortical bone surface, and displaced fragments may be evident. Doppler US noninvasively assesses the integrity of the brachial artery.
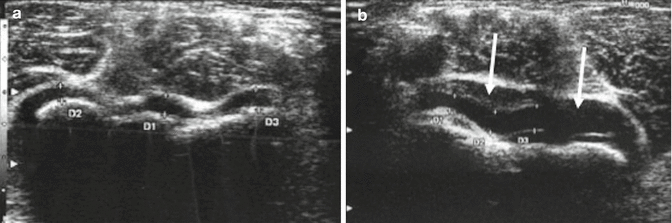
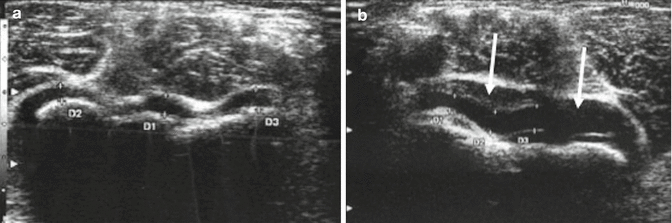
Fig. 8.9
Joint effusion demonstrated at ultrasound (US). (a) Transverse view of normal olecranon bursa for comparison showing minimal joint fluid. (b) Anechoic joint effusion (arrows) anterior to the surface of the humerus (D1-D3 label the measurements of the joint fluid)
Advanced imaging is generally not necessary in the diagnosis and treatment of supracondylar humerus fractures. However, computed tomography (CT) may be used to determine the position of displaced fragments in complex dislocation patterns.
2.2 Lateral Condylar Fracture (Box 8.3)
Box 8.3: Lateral Condylar Fractures
Second most common pediatric elbow fracture (20 %), age 4–10 | |
Varus force to extended elbow in supination | |
Salter II or IV: unstable if extends through trochlear groove | |
Traction from forearm extensors | |
Very subtle in young children as fracture predominantly involves cartilage | |
Small bone flake | |
Faint linear lucency between lateral condyle and trochlea | |
Sequelae | Nonunion and cubitus varus deformity |
Avascular necrosis of trochlea or capitellum |
Lateral condylar fractures are the second most common pediatric elbow fracture, accounting for 20 % of elbow fractures in children. They occur most often between ages 4 and 10 [12]. As with supracondylar fractures, the typical mechanism is a fall on an outstretched arm. This injury is caused by a varus force applied to an extended elbow while the forearm is supinated [13]. These fractures are usually Salter Harris Type II or IV: the fracture involves the metaphysis, physis, and the often incompletely ossified trochlea. Fractures of the lateral condyle are unstable and tend to become displaced even when immobilized because of traction from forearm extensors.
Several classification systems assess lateral condylar fractures. The amount of displacement can guide treatment (0–2 mm indicates casting, 2–4 mm indicates closed reduction and pinning, and >4 mm indicates open reduction and internal fixation). The Milch system determines stability by assessing whether the distal fracture extends to the trochlea. Type I fractures traverse the capitellar ossification center, passing lateral to the trochlear groove. They do not disrupt the trochlea, and therefore the elbow is stable. Type II fractures, which are most common, extend medial to the capitellum, sometimes passing through the trochlear groove, and pass through the physis and lateral trochlea, potentially allowing the ulna to subluxate [13]. Although commonly used in the USA, the Milch classification shows poor interobserver and only moderate intraobserver agreement [14] and in the majority of cases correlates poorly with intraoperative findings [15]. The Rutherford classification is also used to evaluate the stability of lateral condylar fractures, assessing whether the fracture line extends to the articular surface and whether the fragment is displaced [16].
The Weiss classification system, based on the amount of fracture fragment displacement and the presence of articular congruence, can direct surgery and predict likelihood of complications [17]. According to this system, a Type I fracture is displaced less than 2 mm. Type II and Type III fractures are displaced by 2 mm or more. The articular cartilage is intact with Type II fractures, whereas with Type III fractures, it is not. Both Types II and III require surgery, but surgical complications are more likely with Type III factures.
Nonunion is unfortunately relatively common with lateral condylar fractures. Residual deformity is common as well, most often cubitus varus due to progressive overgrowth of the lateral condyle [18] (the normal carrying angle is 15° of valgus). Occasionally, cubitus valgus (Fig. 8.10) due to lateral condylar growth arrest or delay and continued growth of the medial condyle occurs. In addition, in children, the vascular supply of the trochlea is vulnerable to injury [19], and resultant avascular necrosis may result in a fishtail deformity. Avascular necrosis of the capitellum may cause growth disturbance and deformity of the capitellum and radial head. Displaced lateral condylar fractures can result in both physeal and articular incongruity [20].
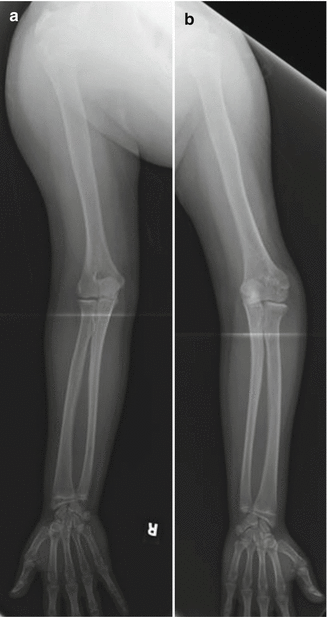
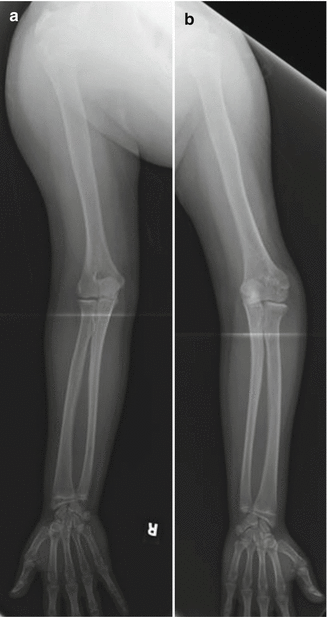
Fig. 8.10
Cubitus varus deformity. Frontal views of both arms with the forearm in supination show a normal right elbow (a). On the left (b), there is varus angulation at the elbow due to residual deformity after remote supracondylar fracture (Courtesy of Sandra L. Wootton-Gorges)
Nondisplaced lateral condylar fractures with intact articular cartilage can be treated by immobilization and close follow-up. Displaced fractures should be stabilized with two divergent K-wires or screw fixation of the fracture through the metaphyseal fragment [12] and often require open reduction. Unlike most pediatric fractures, these heal slowly.
Imaging
Lateral condylar fractures can vary from a subtle cortical condylar avulsion to total lateral condylar avulsion and rotation. Subtle fractures may manifest only as a faint linear lucency between the lateral condyle and the trochlea (Fig. 8.11) or as a lateral metaphyseal flake with possible disruption of the radiocapitellar line. Especially in young children, the extent of the fracture may be grossly underestimated, as only a tiny flake may be evident, since the trochlear extent of the fracture traverses cartilage and is thus radiographically occult. Occasionally, only a cortical fragment of the capitellum is avulsed, which can be very difficult to detect [7]. Mild displacement may be evident, facilitating diagnosis.
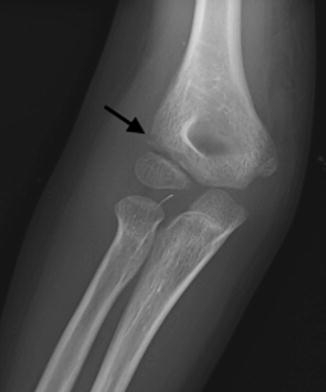
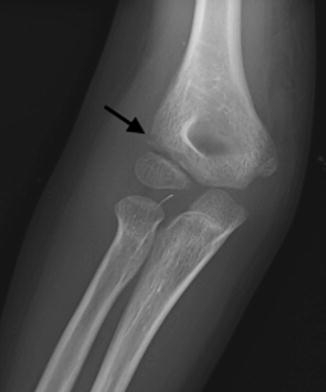
Fig. 8.11
Subtle lateral condylar fracture in an 8-year-old boy who fell on his outstretched arm. Subtle lucency proximal to the capitellum (arrow) may not extend to the trochlea. This fracture did not require fixation and is probably Milch Type I
This fracture may be much easier to appreciate on a lateral radiograph, as a large component can be directed posteriorly (Fig. 8.12). If the fracture extends to the articular surface, the joint becomes unstable, and the fragment is more likely to rotate (Figs. 8.13 and 8.14). Less commonly, the lateral condylar fracture extends through the capitellum itself. Isolated avulsion of the lateral epicondyle is a relatively rare injury, more often encountered in older children.
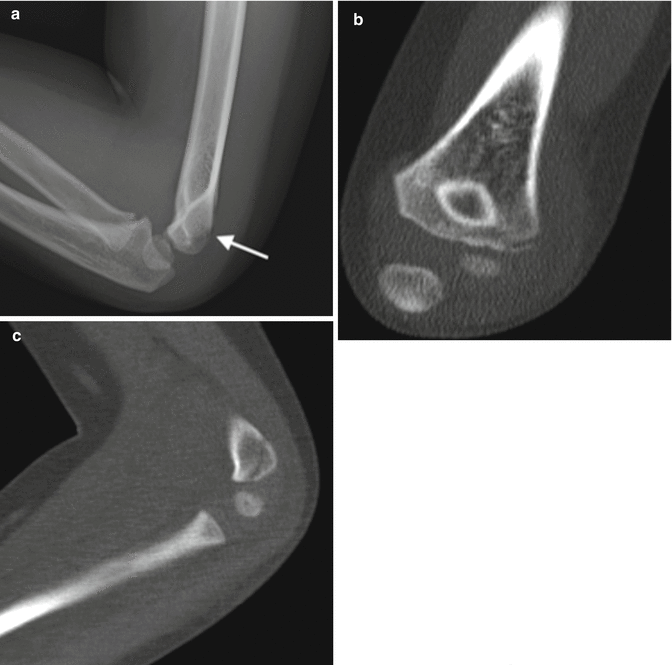
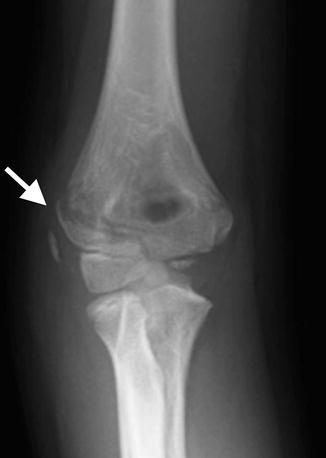
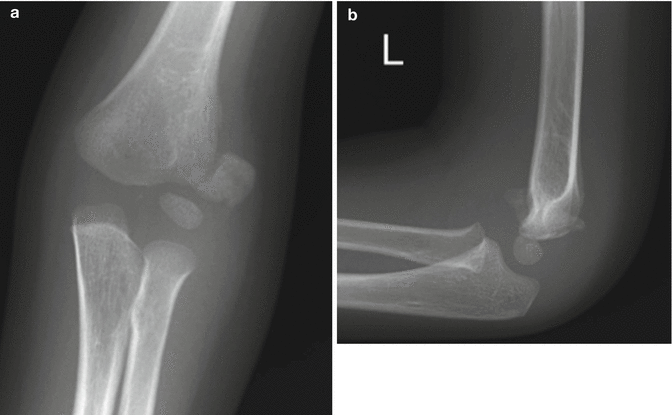
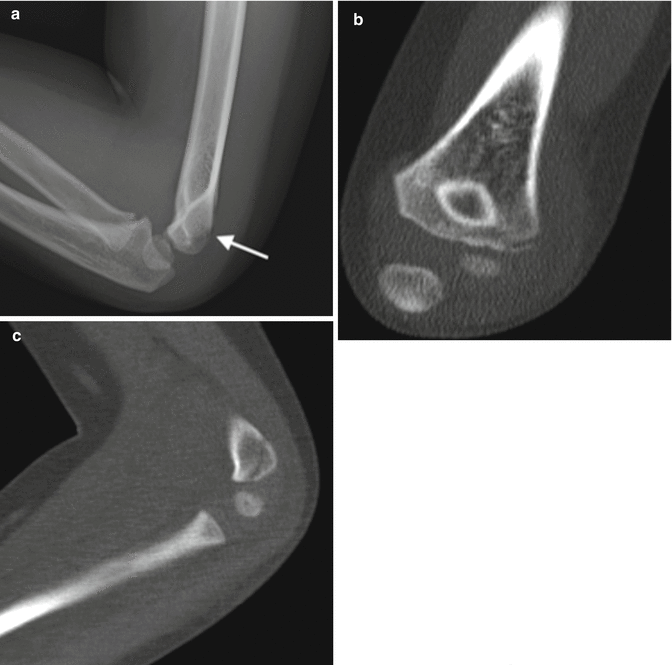
Fig. 8.12
Lateral condylar fracture seen on lateral radiograph. (a) Lateral view demonstrates subtle linear lucency (arrow) directed posteriorly. Coronal reformatted CT (b) shows lateral condylar location of fracture, which extends to the physis on the (c) sagittally reformatted CT (Courtesy of Rebecca Stein-Wexler)
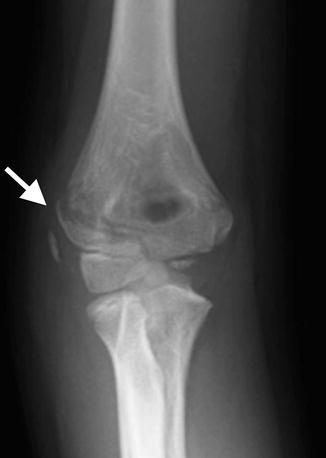
Fig. 8.13
Mildly displaced and rotated supracondylar fracture (arrow)
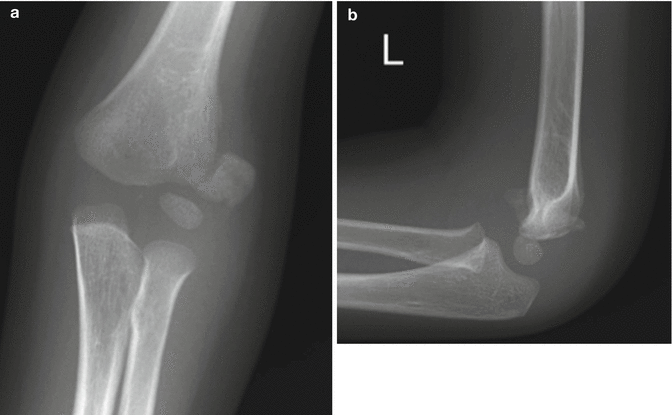
Fig. 8.14
Severely displaced and rotated lateral condylar fracture. (a, b) This is most likely a Salter Harris Type IV, with the fracture line crossing the physis and joint line
Because lateral condylar fractures can be difficult to identify, it is important to seek clues, such as lateral soft tissue swelling on the frontal radiograph and a positive fat pad on the lateral (discussed in detail in the section on supracondylar fractures, above). An oblique radiograph with the arm internally rotated best demonstrates the amount of displacement and rotation of the lateral condylar fragment [21]. A significant gap in the lateral cortex may indicate articular discontinuity. It may take 4–14 days for the fracture line to appear, and the metaphyseal fragment can be very small and therefore overlooked. Furthermore, the diagnosis may be extremely difficult before the capitellum is ossified.
US can help establish the diagnosis of lateral condylar fracture by detecting joint fluid as well as cortical irregularity or an actual step-off at the lateral condylar bony or cartilaginous surface. CT may demonstrate the position of the displaced fragments in complex dislocation patterns (Fig. 8.15). Magnetic resonance imaging (MRI) can evaluate concomitant soft tissue injuries such as injury to the lateral collateral ligament complex, the muscles of supination, and/or the forearm extensors that are attached to the lateral condyle.
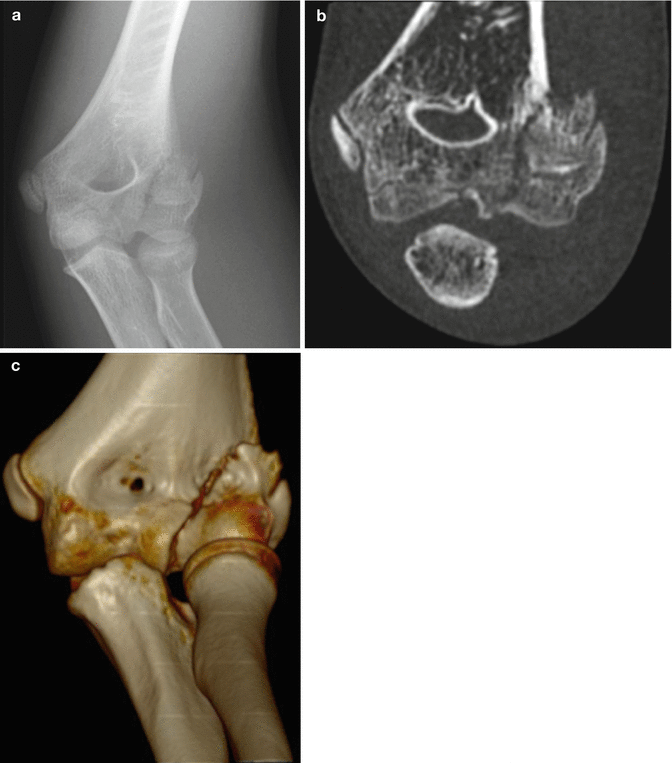
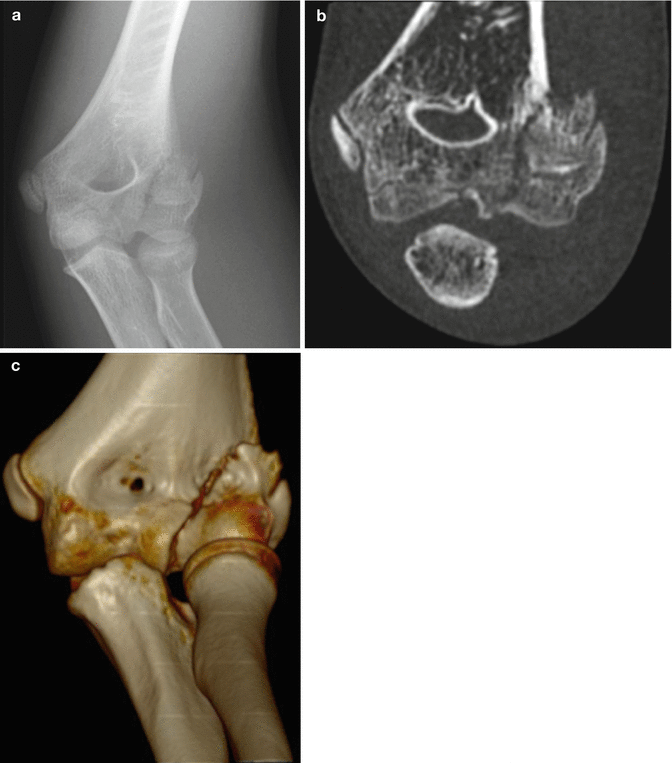
Fig. 8.15
Lateral condylar fracture. (a) The radiograph shows slight separation of the fragment at this Salter Harris IV fracture. Coronally reformatted CT (b) and 3D reconstruction (c) better define the extent of displacement
2.3 Medial Epicondylar Avulsion Fracture (Box 8.4)
Box 8.4: Medial Epicondylar Fractures
Less common, ages 8–15 |
Valgus strain causes ulnar collateral ligament and forearm flexors to pull on medial epicondyle |
50 % also have dislocated elbow at presentation |
Radius and ulna posteriorly displaced |
Fracture at cartilaginous physis (Salter I) |
Physis may appear wide |
Fragment often displaced, may become trapped in joint near trochlea |
May mimic normal trochlear center |
Apply CRITOE to avoid mistakes—I before T |
Medial epicondylar avulsion fractures are less common and occur in older children, usually between 8 and 15 years [7]. Fractures of the medial epicondyle are associated with dislocation of the elbow joint at presentation in half of cases [6]. Both the radius and the ulna are usually posteriorly displaced [13]. The mechanism of injury is valgus strain to the joint, which causes the ulnar collateral ligament to exert traction on the medial epicondyle. This avulsive force causes the medial epicondyle to fail. The bone tends to separate at the cartilaginous physis—the weakest link in the pediatric skeleton. Another avulsion injury consists of purely muscular avulsion secondary to contraction of the forearm flexors when the elbow is flexed. This mechanism may be responsible for medial epicondylar fractures associated with arm wrestling and overhead throwing [22].
A displaced bony fragment can injure the ulnar nerve, which passes through a groove in the posterior aspect of the medial epicondyle. Furthermore, traction on the medial epicondyle secondary to contraction of forearm flexor muscles and tension from the medial collateral ligament may cause the fractured epicondyle to dislocate. In severe cases, the dislocated epicondyle may be trapped in the elbow joint, limiting joint mobility.
Nondisplaced fractures may be treated nonoperatively [6]. Open reduction (with K-wires for younger children, a screw for older) is usually performed if displacement exceeds 15 mm. However, such issues as hand dominance and whether the patient participates actively in sports affect the decision of whether to operate.
Imaging
The sequence and timing of ossification of the trochlea and medial epicondyle are important in interpreting medial elbow fractures. If the medial epicondyle is fractured and displaced, it may become entrapped between the trochlea and the coronoid process of the ulna (Fig. 8.16). In this position, it may be confused with the normal trochlear ossification center if that center has not yet ossified. However, that would imply that the trochlea had ossified before the medial epicondyle, which would be unusual. Infrequently, variant ossification order confounds this issue. In that case, imaging the contralateral elbow may help.
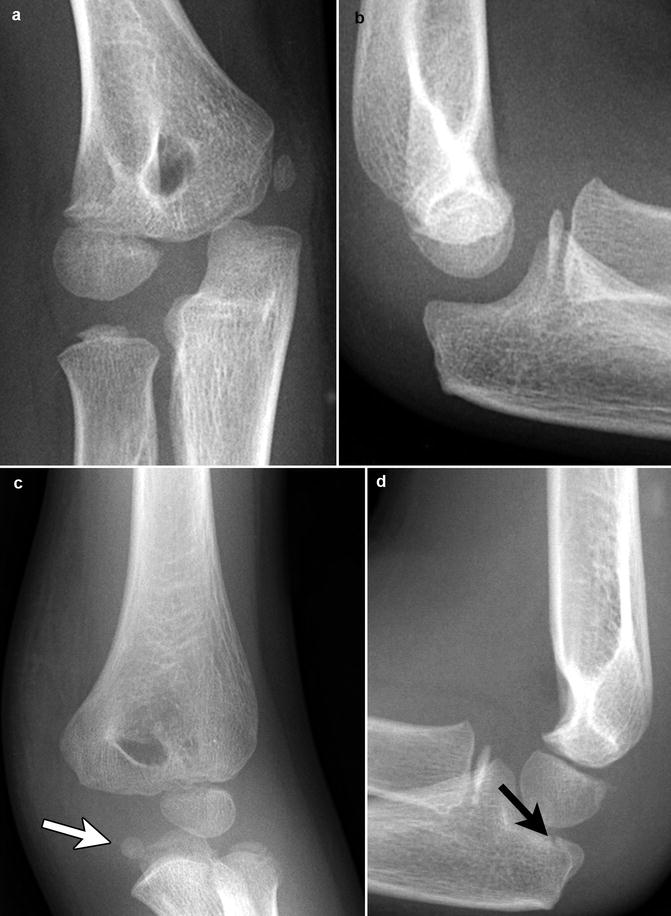
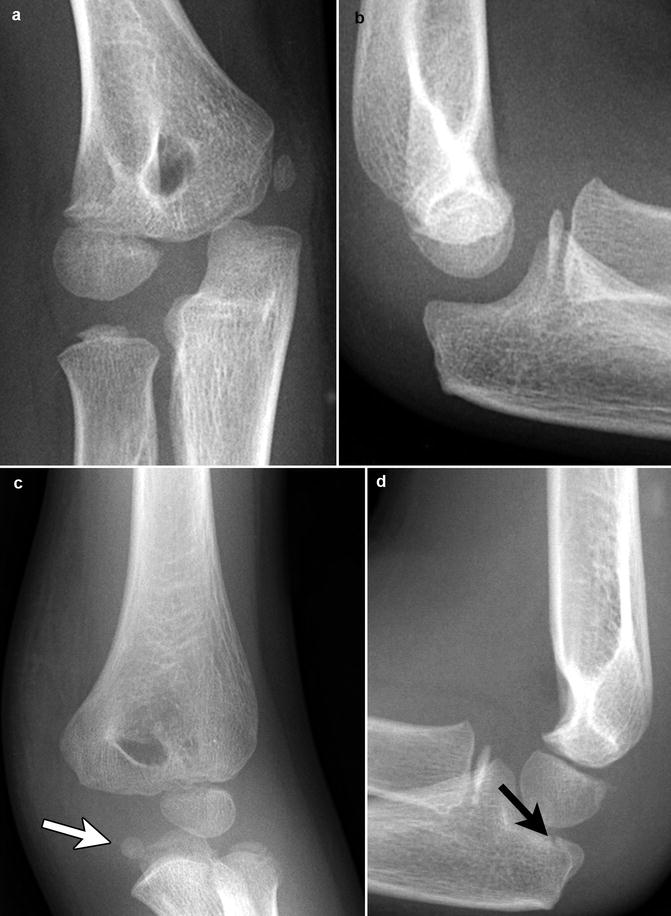
Fig. 8.16
Medial epicondylar fracture in a 6-year-old boy. (a, b) Normal right side for comparison. Note trochlear and olecranon apophyses have not yet ossified and normal position of the medial epicondyle. (c) The left medial epicondyle is displaced into the joint (white arrow). (d) There is also a nondisplaced olecranon fracture (black arrow) (Courtesy of Sandra L. Wootton-Gorges)
A lateral radiograph demonstrates a positive fat pad sign due to effusion, and a dislocated medial epicondyle may be apparent (see Fig. 8.6). On the frontal view, there is medial soft tissue swelling. Before ossification, radiographs can only suggest the possibility of fracture due to joint effusion or soft tissue swelling. If ossification has begun, the medial epicondylar physis may appear abnormally wide (Fig. 8.17).
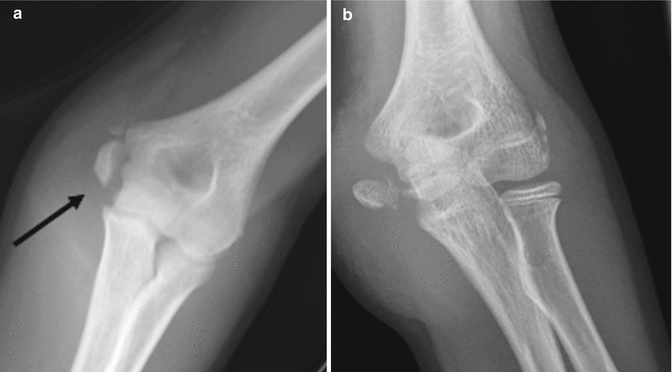
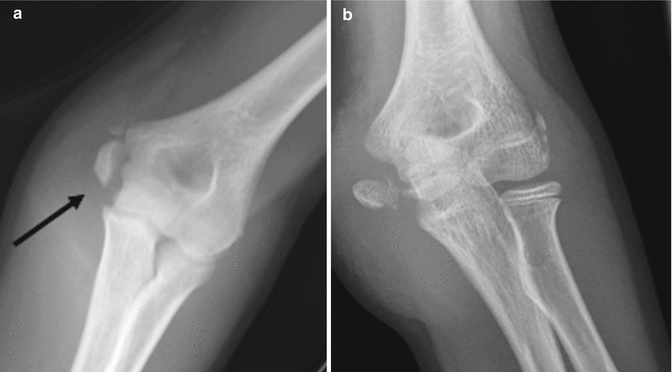
Fig. 8.17
(a) Medial epicondylar fracture in an 11-year-old girl. The physis at the medial epicondyle is abnormally wide (arrow), and the medial epicondyle slightly displaced. (b) Another child with a more displaced medial epicondylar fracture
US can assist with diagnosis prior to ossification of the medial epicondyle. MRI is usually not necessary but demonstrates physeal widening, bone contusion with edema on both sides of the physis, and possible dislocation of the medial epicondyle.
Differential Diagnosis
Fractures of the medial epicondyle may be mistaken for the very rare trochlear fracture. They may also be confused with medial condylar fractures that involve the medial column but are extra-articular. These two fracture patterns are treated differently and should not be mistaken. Elbow dislocation is very uncommon with medial condylar fractures but commonly encountered with fractures of the medial epicondyle.
2.4 T (or Y) Fracture
T- (or Y-) shaped fractures are intercondylar fractures with varying displacement between the condyles and the humerus. These fractures are uncommon in children and are generally caused by a blow to the elbow.
Imaging
Radiographs show that the intercondylar distance is increased, and there are displaced fracture fragments. These fractures require reconstruction of the articular surface and reattachment to the shaft [6]. CT or MRI helps with planning reconstruction.
2.5 Complete Physeal Displacement
Complete physeal displacement is a specific type of supracondylar fracture that generally occurs before age 2 [6]. As the name suggests, it is characterized by a fracture line that traverses the physes of the medial epicondyle, trochlea, capitellum, and lateral epicondyle, with displacement of this cartilaginous mass. The non-ossified epiphyses are markedly displaced but difficult to recognize, since the earliest to ossify—the capitellum—may ossify as late as 12 months. Delayed diagnosis increases the likelihood of avascular necrosis of the distal humerus. It is therefore important to maintain a high index of suspicion for this type of fracture in the infant who presents with a swollen elbow after birth or trauma. Treatment involves either closed reduction with a cast or pinning [6].
Imaging
On a frontal radiograph, the proximal radius and ulna appear displaced medially relative to the distal humerus, although they maintain a normal relationship to each other (Fig. 8.18). The radius and ulna appear posteriorly displaced on the lateral view.
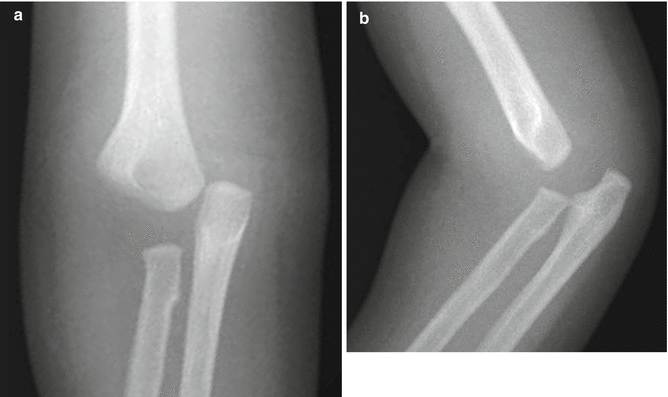
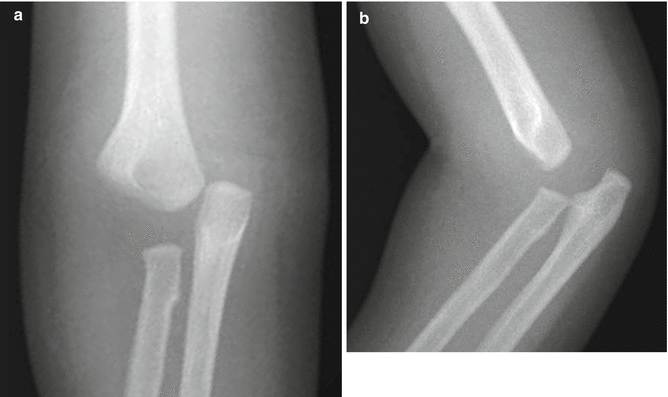
Fig. 8.18
Complete physeal displacement in an infant. (a, b) The radius and ulna maintain a normal relationship with each other but are displaced medially and posteriorly with respect to the distal humerus
Differential diagnosis includes elbow dislocation, but elbow dislocations in young children are exceedingly rare and generally show the proximal radius and ulna displaced posterolaterally instead of posteromedially, with disruption of the relationship between the proximal radius and the capitellum [23]. US or MRI successfully establish the diagnosis.
3 Fracture of the Radius and Ulna
3.1 Radial Neck Fractures
The radial neck is usually fractured during a fall on an outstretched arm. The compressive force causes the radius to impact upon the capitellum. Fractures in this area typically involve either the physis (Salter Harris I) or the metaphysis and physis (Salter Harris II) [6, 24]. Crepitus at the radial head and tenderness with gentle pronation and supination are common. In the acute setting, motion is usually limited by a painful hemarthrosis, rather than a true mechanical block. However, displaced fracture fragments sometimes prevent forearm rotation.
The relationship between the radial head and the annular ligament determines the functional outcome of this fracture. The extent of angulation helps direct treatment; the AO classification scheme can be employed to describe fragment position (Fig. 8.19).
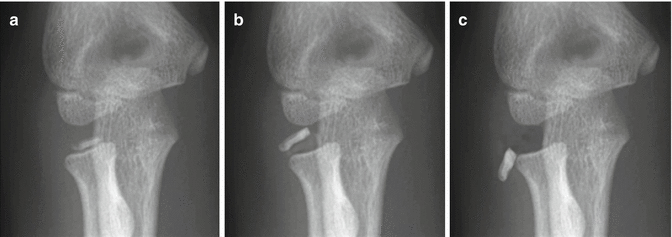
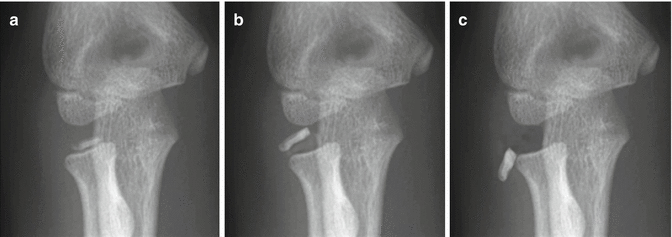
Fig. 8.19
AO classification of radial neck fractures. (a) With Type I, there is no angulation or displacement. (b) With Type II, the radial head is displaced less than 50 % of its diameter, and it appears angled. (c) Displacement more than 50 % (with angulation) constitutes Type III
In general, any translation should be corrected, whereas angulation up to 30° (some suggest 50°) may be treated conservatively [6], depending on the age of the child. Displaced fractures require surgical stabilization with K-wires or an intramedullary elastic nail.
Imaging
As with all elbow fractures, injury to this area must be assessed with an understanding of when ossification centers ossify. Before radial head ossification, radiographs cannot differentiate radial head dislocation from physeal fracture with displacement. Frontal and lateral radiographs of the elbow are generally supplemented with either a radiocapitellar view or a Greenspan view (with the beam angled 45° cephalad and the elbow in neutral position).
The disk-shaped epiphysis at the proximal end of the radius articulates with the capitellum and with the lesser sigmoid notch of the ulna. Intra-articular in location, the radial head should point toward the capitellum on all views (Fig. 8.20). With injury, there may be a fracture line, abnormal alignment, angulation of the radial head, or depression, comminution, or displacement of the radial head or neck (Fig. 8.21). The fat pads are almost always elevated, indicating joint effusion. Small bony intra-articular fragments are infrequently present [25, 26].
