Fig. 1.1
Henry T. Wycis holding Spiegel–Wycis stereotactic frame with Horsley-Clarke frame on the table. From Textbook of Stereotactic and Functional Neurosurgery. Lozano, Andres M.; Gildenberg, Philip L.; Tasker, Ronald R. (Eds.) 2nd ed. 2009; used with permission
Concurrent radiobiological innovations played an equally important role as stereotactic ones in the development of SRS. Ionizing radiation was used for therapeutic purposes soon after it was discovered; three months after Wilhelm Konrad Roentgen published his report in 1895, X-rays were used to treat skin and breast cancers [3, 9]. The discovery of radioactivity by Becquerel in 1896, and of radium by the Curies soon after, opened the door for the medical use of radioisotopes. X-rays were used to treat patients with pituitary tumors as early as 1906, and radium brachytherapy was applied to treat similar conditions at about the same time [10]. Harvey Cushing, the father of American neurosurgery, had extensive experience with both X-ray and brachytherapy treatments, although he remained skeptical of the utility of either [11]. Dosimetry was poorly understood, and treatments were not standardized. Nonetheless, some neurosurgeons continued to explore the uses of ionizing radiation, and new methods continued to be developed through the 1950s.
Emergence of Radiosurgical Practice
Lars Leksell conducted his earliest work with SRS while serving as head of the neurosurgery department at the University of Lund [12]. His preliminary work with a 250 kVp X-ray unit attached to a single beam port resulted in the successful management of pain control in trigeminal neuralgia patients (Fig. 1.2) [2, 5]. A ceaseless innovator, Leksell noticed that if he used higher energy radiation, depth dose and beam definition could be improved, especially in smaller treatment fields [12]. He then began working with Borje Larsson, a radiobiologist at the Uppsala University cyclotron unit, to investigate the use of converging proton beams for neurosurgery (Fig. 1.3). When these beams proved too complicated and difficult to use in a hospital setting, Larsson and Leksell began collaborating with Kurt Liden, a medical physicist at Lund.
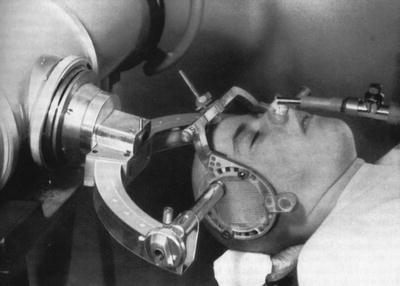
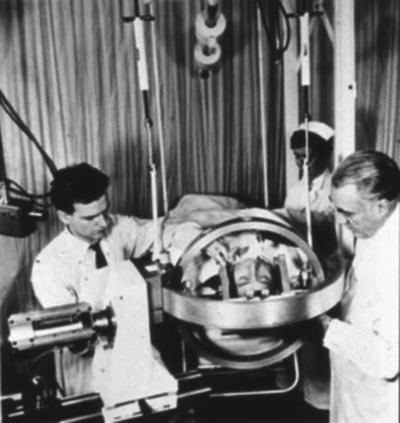
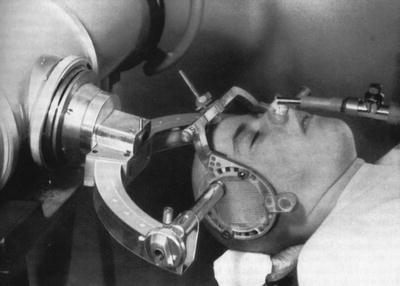
Fig. 1.2
First stereotactic instrument for radiosurgery using 280 kV X-rays, early 1950s. From Textbook of Stereotactic and Functional Neurosurgery. Lozano, Andres M.; Gildenberg, Philip L.; Tasker, Ronald R. (Eds.) 2nd ed. 2009; used with permission
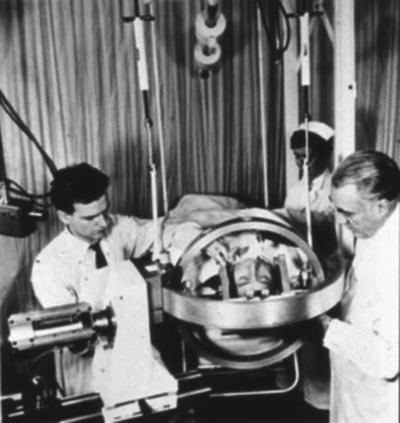
Fig. 1.3
Lars Leksell and his physicist colleague, Borje Larsson, preparing a patient for SRS with a particle beam accelerator in 1958. (Photo courtesy of L. Dade Lundsford, MD)
After experimenting with particle beams and linear accelerators, Leksell and his colleagues ultimately designed the gamma knife (GK), containing 179 cobalt sources in a hemispheric array (Fig. 1.4). The first unit was operational in 1968; Leksell’s first patient was immobilized using a molded plaster headpiece and treated for a craniopharyngioma [4]. The potential of the GK to treat neoplasms and vascular anomalies, as opposed to functional targets such as lesioning for control of pain and movement disorders, was recognized by Leksell early on. In the precomputed tomography (CT) era these treatments were mostly limited to patients with arteriovenous malformations (AVMs) [13] and acoustic neuromas, which could be imaged either on angiography or by polytomography, respectively [3].
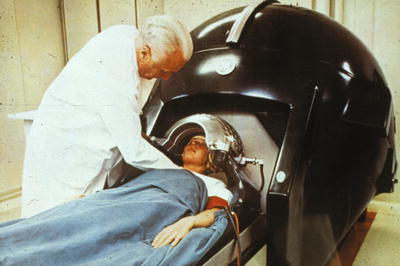
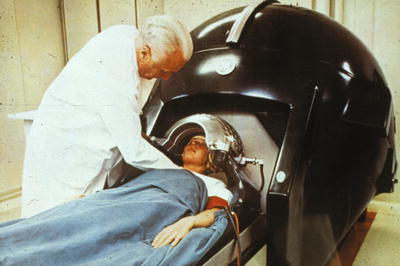
Fig. 1.4
Lars Leksell treating the first case of acoustic neurinoma with first version of gamma knife, 1968. From Textbook of Stereotactic and Functional Neurosurgery. Lozano, Andres M.; Gildenberg, Philip L.; Tasker, Ronald R. (Eds.) 2nd ed. 2009; used with permission
At the same time, work was continuing elsewhere with focused heavy particle irradiation. John Lawrence, whose brother Ernest won the 1939 Nobel Prize for his invention of the cyclotron, began experimenting with charged particle radiation in 1954. He treated patients with pituitary and other intracranial disorders (Fig. 1.5) [14, 15] using proton and helium ion beams. He initiated the use of the Bragg peak principle according to which proton beams deposit their energy at a distinct point, with minimal exit dose. In practice, heavy particle beams must be carefully shaped and spread in order to treat patients with intracranial lesions.
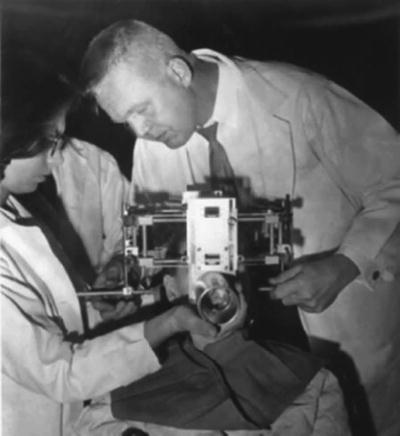
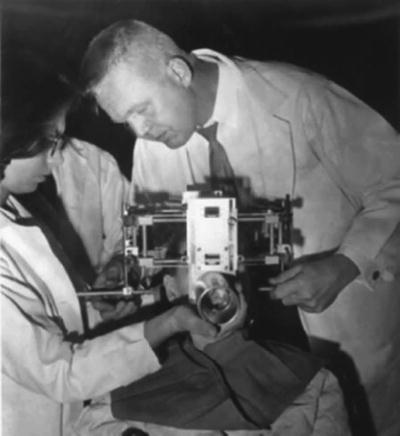
Fig. 1.5
Raymond Kjellberg with a frame for proton beam therapy of a patient with an AVM. (Photo courtesy of Richard Wilson, Mallinckrodt Research Professor of Physics, Harvard University)
After visiting Stockholm in 1959, Raymond Kjellberg, a neurosurgeon at the Massachusetts General Hospital, began the use of Bragg peak proton beam treatments in the USA [16]. He amassed a large series of patients with arteriovenous malformations and pituitary tumors. Similar efforts were carried out in California with helium ions [17]. For decades, the expense of building and maintaining a cyclotron has limited the use of heavy particle SRS to a few centers. In the last few years, new technology has decreased the size and cost of proton beam irradiation devices, and the number of such facilities has grown.
Acceptance
When SRS was first invented, stereotactic localization relied on atlases, ventriculography, and angiograms. The advent of the computerized tomography (CT) scanner in the mid-1970s, and MRI some 10 years later, opened up the possibility of direct targeting of tumors and other “soft tissue” targets inside the skull. Image-guided stereotaxy became available for brain biopsy, craniotomy, tumor resection, and functional neurosurgery. Due to this, the 1980s saw the evolution of SRS from an esoteric technique, available at the original GK in Stockholm (and as fractionated treatments at the few heavy particle accelerators around the world), to an emerging technology of increasing utility.
In 1984, after several years of intense regulatory reviews and logistical battles, Dade Lunsford and colleagues completed the installation of the first American GK at the University of Pittsburgh [18]. This group was instrumental, via an ongoing series of peer-reviewed publications, in placing the technique and clinical indications for SRS on a sound scientific basis. Since then, several new models of GK units have become available. The most current high-end model, the GK Perfexion, enables automated collimator changes and improves access to intracranial targets.
As the potential horizons of SRS broadened, other investigators were able to adapt linear accelerators (LINACs) for SRS (Fig. 1.3) LINAC units create X-rays by accelerating electrons to almost the speed of light, then directing the electron beams to a heavy metal alloy. The resulting X-ray is collimated and focused onto a target. Before inventing the GK, Lars Leksell and his colleagues at the Karolinska Institute experimented with LINAC, but abandoned the system due to low photon energies and mechanical inaccuracy [4]. However, the ability to use CT imaging for lesion localization and beam targeting greatly increased the accuracy of LINACs. These systems were a cheaper alternative to GK or heavy particle accelerators [19] and were quickly adapted. Working independently, in Buenos Aires and Vicenza, Italy, respectively, Betti and Colombo reported the successful use of LINACs for SRS [20, 21] in 1982. Their systems allowed for the rotation of the LINAC gantry in a single plane.
At about the same time, Winston and Lutz described the use of a commercially available stereotactic frame for LINAC radiosurgery [22]. Following in their footsteps, Loeffler and Alexander demonstrated how a LINAC dedicated to SRS could be a practical alternative to a GK [23]. In the late 1980s Friedman and Bova elected not to install the second American GK unit, preferring to develop a new LINAC SRS system [24]. Other advantages of these LINAC systems, besides ubiquity and lower cost, included the availability of collimators in a much greater variety of diameters than provided with the GK. This allowed for the use of single isocenters when treating patients whose targets were over 18 mm in diameter, the width of the largest GK collimator. However, at around the same time several GKs were installed in several sites around the world.
As clinical experience increased, publications appeared, indications broadened, and vendors became increasingly interested, a debate emerged regarding the merits of the Gamma Knife versus LINAC-based SRS. By now, clinical and physics studies indicate that SRS can be delivered effectively and accurately with either method [19, 25]. Numerous reports demonstrating the efficacy of SRS with few if any short-term complications and lower costs led to the proliferation of GK and LINAC units around the world.
Fractionation, Extracranial Radiosurgery, and Other Advances
As the Gamma Knife and LINAC systems continued to improve treatment outcomes, it became clear that both technologies had promise for wider application. Rapid advances in neuroimaging, robotic technology, and beam targeting soon followed. John Adler, a neurosurgeon who trained at the Brigham and Women’s Hospital in Boston, spent a fellowship year with Lars Leksell in 1985 (Adler JR, personal communication). Excited by his exposure to the GK, Adler saw the potential of SRS being extended to other areas of the body. This required a method of delivering focused radiation without a stereotactic frame. Partnering with engineers at Stanford University and with private financial backing, the Cyberknife ultimately came into being in 1994 (Fig. 1.6).
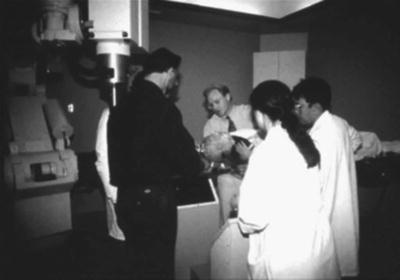
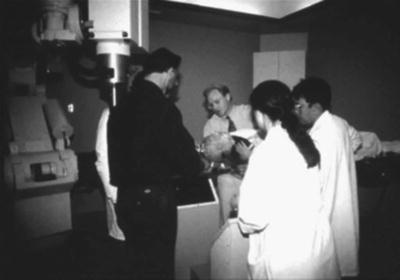
Fig. 1.6
The first Cyberknife treatment, 1994. (Photo courtesy of John R. Adler, MD)
The Cyberknife delivers SRS via an X-band LINAC with an output of 6 MV. It is nonetheless small enough to be mounted on an industrial robot, allowing for a theoretically infinite number of beams to be aimed at the target. For treatment of intracranial targets, the patient is immobilized using a customized, molded plastic mask system that offers a high degree of reproducibility between multiple fractions. Treatments are fashioned using an inverse planning method; to allow for practical computation times, the number of beam origins (“nodes”) and robot angles are limited. Various other LINAC-based systems have been developed to improve dose planning and delivery through beam shaping and intensity modulation. These systems include XKnife (Radionics), Novalis (BrainLAB), and Peacock (NOMOS Corporation). Single fraction SRS technologies have also been evolving; a rotating Gamma Knife system (RGS), invented in the 1990s, changes beam diameters during treatment without interchanging collimator helmets, which increases the system’s flexibility and decreases treatment time.
In addition, CT scanners are now being incorporated into LINAC-based systems. The Tomotherapy Hi-Art system, invented in the 1990s, provides integrated treatment planning, patient setup, and CT-guided treatment. The newest Novalis TX system includes an on-board imaging cone beam CT scan to improve visualization of soft tissue targets during treatment and an ExacTrac image-guidance system to adjust for minute patient movements. These developments allow for real-time updated imaging during treatment to ensure positioning accuracy. Perhaps more significantly, this improves intrafraction targeting accuracy as well. Peer-reviewed publications have demonstrated the acceptance of the Cyberknife and Novalis frameless systems [26–29]. These and other articles have fostered a useful debate regarding the concept of hypofractionation in SRS and indeed if such treatments are still “radiosurgical” [30, 31].
Extracranial SRS
With frameless LINAC-based SRS and SRT, it became possible to further step outside the bounds of traditional radiosurgery and treat targets outside the brain. The first radiosurgical moves out of the intracranial compartment were in the logical direction of the skull base and past that into the paranasal sinuses, using either GK [32, 33] or LINAC units [34]. Creative modifications of standard stereotactic systems, such as the vacuum pillow stabilizer described by Lax in 1994 [35], were designed to allow for treatment of targets throughout the body. Hamilton and colleagues described the first truly extracranial radiosurgical unit in 1995. This prototypical system relied on a skeletal fixation frame and was designed to provide spinal SRS [36]. The need to surgically place a clamp on a spinous process and to treat the patient in a prone position limited the appeal of this groundbreaking concept. Non-CNS targets were later treated using newer stereotactic technologies such as the Elekta Stereotactic Body frame. However, with the advent of new frameless techniques and improvements in beam targeting, such external devices for rigid fixation and localization are no longer necessary. Table 1.1 summarizes the historical landmarks in the development of SRS.
Table 1.1
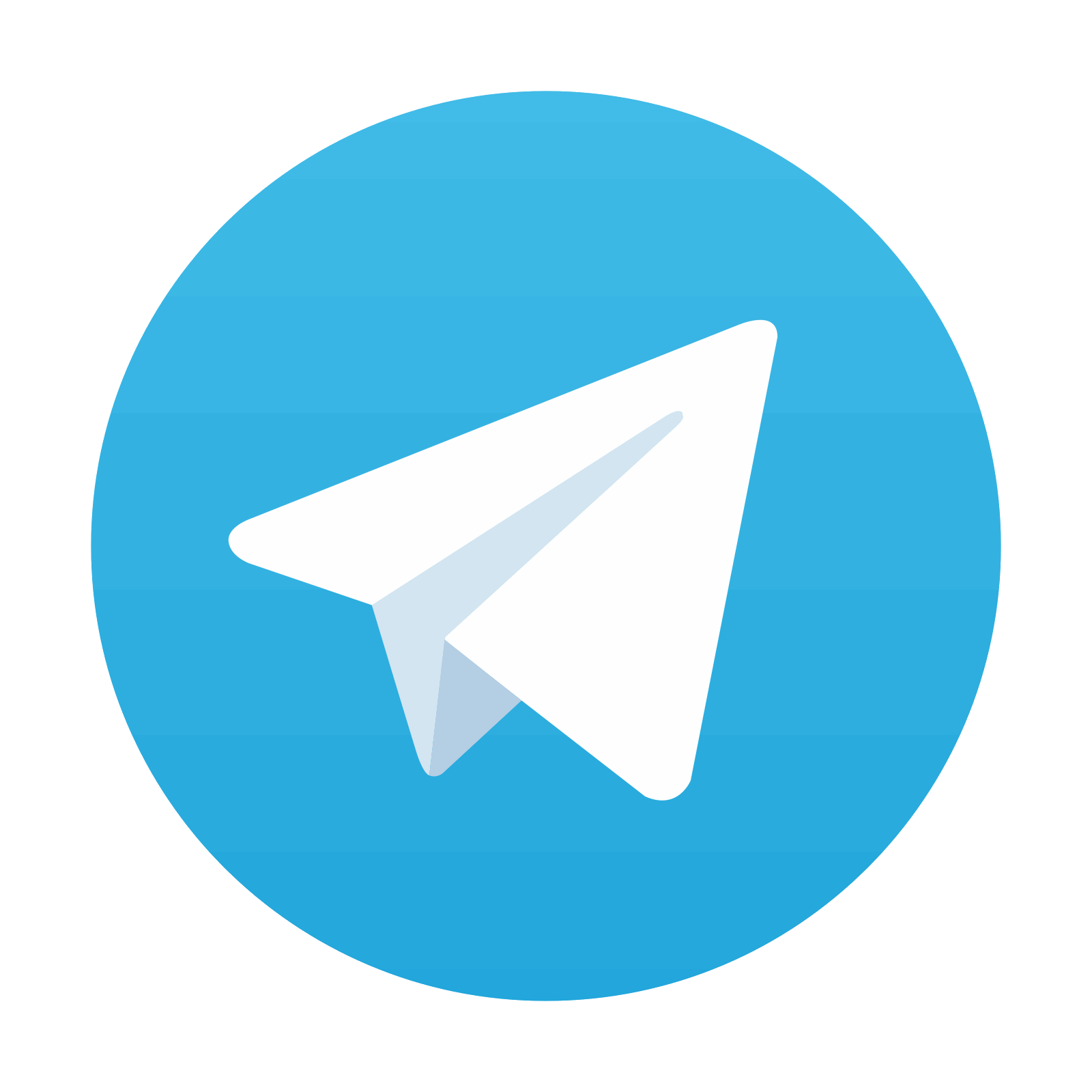
SRS landmarks
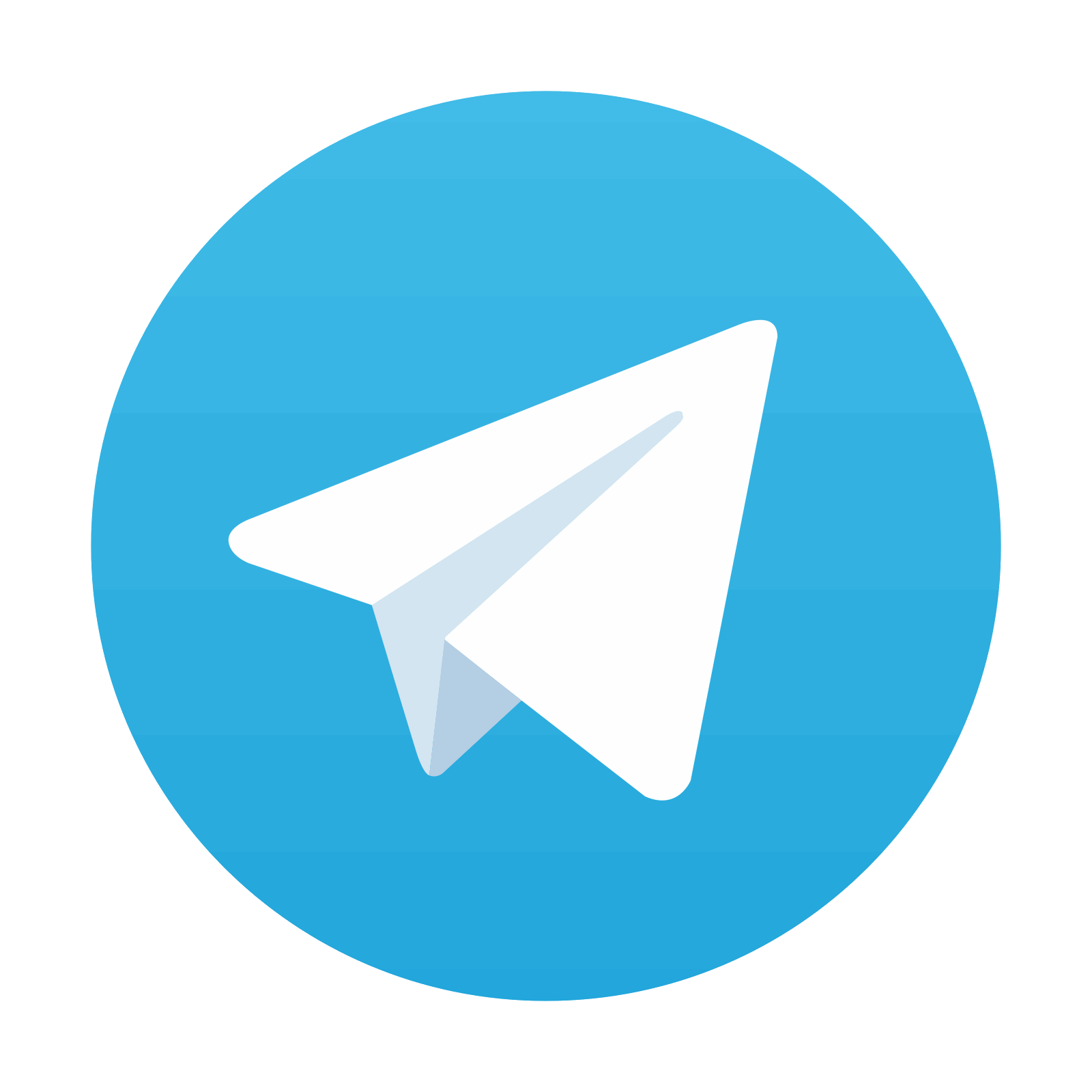
Stay updated, free articles. Join our Telegram channel

Full access? Get Clinical Tree
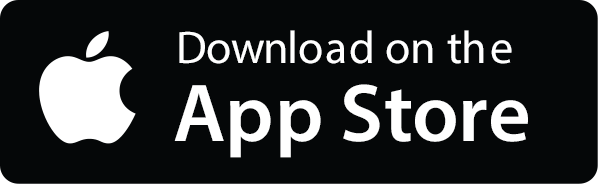
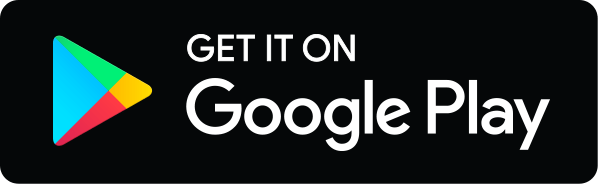
