The median anterior and central skull base forms an interface between the sinonasal and intracranial compartments. Due to the proximity of the intracranial structures, skull base involvement is a key assessment when evaluating the spread of sinonasal disease. This review describes the pertinent anatomy and the role of computed tomography and MR imaging in evaluating the median anterior and central skull base. The imaging appearances of pathologic processes that involve and traverse the skull base between the sinonasal and intracranial compartments are discussed and illustrated.
Key points
- •
Computed tomography (CT) helps assess the cortical bone changes of the anterior skull base (ASB) whereas MR imaging is superior for evaluating the bone marrow and foramina of the central skull base (CSB).
- •
The paranasal sinuses contribute to the median ASB and CSB, and important variant anatomy should be recorded to help prevent surgical complications.
- •
MR imaging is useful in delineating sinonasal malignancy by demonstrating direct extension through the ASB, perineural extension through the CSB and involvement of intracranial structures.
- •
Intrinsic bone lesions are most frequently benign in the ASB whereas primary or secondary malignant intrinsic bone lesions may also feature in the CSB.
- •
Endocranial tumors and contents may traverse the skull base into the sinonasal region. Cephalocele is an important consideration when imaging demonstrates a sinonasal mass with a skull base defect.
Introduction
The median ASB and CSB form an interface between the sinonasal and intracranial compartments. Because clinical and endoscopic assessment is limited, the cross-sectional imaging modalities of CT and MR imaging play a key role in addressing the origin, nature, and extent of disease within the ASB and CSB. Advances in endoscopic surgical approaches, image-guided surgery, and targeted radiotherapy have expanded therapeutic possibilities, and the radiologist is central within the multidisciplinary team when deciding on treatment planning.
This review briefly describes the appropriate CT and MR imaging techniques, and the pertinent anatomy of the median ASB and CSB relevant to the spread of disease and surgical planning. It then focuses on the imaging appearances of pathologic processes that involve and traverse the skull base between the sinonasal and intracranial compartments.
Techniques
CT and MR imaging are frequently complementary in characterizing and demonstrating the extent of pathologic processes within the median ASB and CSB.
CT is excellent at detecting cortical bone erosion or deficiency and hence is particularly useful for assessing the thin bony structures of the ASB or the foramina of the CSB. CT is also used to provide a bony roadmap for surgery. It may help differentiate pathologies by demonstrating calcific or ossific elements (eg, fibrous dysplasia [FD] and osteoma), adjacent bony sclerotic reactions (eg, meningioma, olfactory neuroblastoma, inverted papilloma, and chronic inflammation), bony destruction (eg, high-grade carcinoma or neuroendocrine tumor) or bony remodeling (eg, low-grade tumors, including sarcoma, lymphoma, melanoma, and olfactory neuroblastoma).
Multidetector CT is performed with a single axial volume acquired at submillimetric slice collimation, with generation of multiplanar 1-mm reformats (including a bone algorithm). If concurrent MR imaging is not available, then increased milliampere-seconds (>50 mAs), intravenous contrast, and 3-mm thick reconstructions help optimize the soft tissue appearances. If only bone detail is required, then cone-beam CT may also be used to evaluate the skull base at a lower radiation dose. CT angiography and CT cisternography are additional techniques that may be indicated for evaluation of skull base pathology.
MR imaging is superior for detecting infiltration of the bone marrow and perineural extension of disease, so it is valuable for imaging the CSB. MR imaging also provides superior mapping of the intrasinus extent of lesions (and hence contact with the skull base) while better delineating any intracranial extension.
MR imaging requires a combination of T1W, T2W, and gadolinium-enhanced T1W images. Sagittal sequences supplement routine coronal and axial planes for the assessment of midline pathology. Imaging is generally performed with 3- to 4-mm slice thickness and no gap, with an optimal field of view of 16 to 18 cm. Occasionally, volumetric sequences are used for image-guided surgery and radiotherapy planning and (with 3-D heavily T2W sequences) to demonstrate a cerebrospinal fluid (CSF) leak or cephalocele. T1W sequences are particularly useful for assessing bone marrow abnormality, whereas gadolinium enhancement delineates pathologic meningeal and perineural extension. Higher-resolution (eg, 512 × 512 matrix) sequences may be used to evaluate the integrity of bone and periosteum at the ASB or to assess for perineural spread (PNS). Fat-suppressed sequences are often included for imaging the CSB. Failure of fat suppression at the interface of the skull base and the paranasal sinuses is a problem with sequences based on frequency selective pulses, but this may be overcome by using short tau inversion recovery (STIR) or 3-point Dixon techniques. Diffusion imaging of the paranasal sinuses and the skull base is feasible and can be optimized with parallel imaging, readout segmented techniques, multishot acquisition, and turbo spin-echo techniques; however, there is little data on the diagnostic impact of diffusion-weighted imaging (DWI) in the setting of skull base and sinonasal pathology.
Intraoperative image guidance is frequently required to aid surgical approaches to the skull base. A tracking system (eg, optical or electromagnetic) simultaneously references a sensor on a surgical instrument with the patient and a preoperative or intraoperative imaging data set (using CT or MR imaging) ( Fig. 1 ).
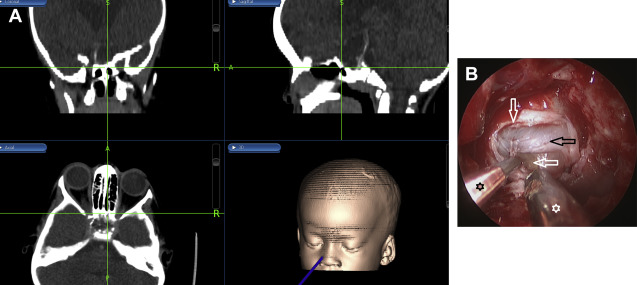
Anatomy
The paranasal sinuses contribute to the ASB and CSB in the midline and paramidline. Thus, the median ASB comprises the posterior wall of the frontal sinus, the roof of the nasoethmoid region, and the planum sphenoidale, whereas more posteriorly, the median CSB houses the sphenoid sinus. A coronal projection through the chiasmatic sulcus, tuberculum sella, and anterior clinoids separates the median ASB from the CSB ( Fig. 2 ). The wide range of variant anatomy within this part of the skull base should be recognized to distinguish it from pathologic processes and to prevent complications of sinonasal surgical approaches ( Table 1 ).
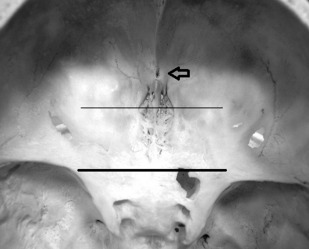
Median ASB | |
Angulated or low fovea ethmoidalis | A steeper sagittal skull base angle and a lower position of the fovea ethmoidalis relative to the orbit is associated with an increased incidence of surgical damage. |
Deep cribriform plate or asymmetric lateral lamellae (see Fig. 5 ) | A deep cribriform plate relative to the fovea ethmoidalis (hence, longer lateral lamella as graded by the Keros classification) or an asymmetric configuration increases the risk of penetration at surgery. |
Anterior ethmoid artery dehiscence (see Fig. 5 ) | It should be noted whether the anterior ethmoid artery lies immediately adjacent to the skull base before it enters the cribriform plate or whether it is suspended by a mesentery (as is present in 30% of cases) where it may be vulnerable to injury. |
Median CSB | |
Extent of sphenoid sinus pneumatization | Presellar or conchal type pneumatizations are less favorable for transsphenoidal surgical approaches to the pituitary fossa. |
Variations in sphenoid sinus septae (see Fig. 10 ) | There are challenges to sphenoid sinus surgery posed by the considerable variation in the configuration of intersphenoid sinus septations. An asymmetric septation inserting on the (possibly dehiscent) carotid canal should be communicated to the surgeon. |
Dehiscent bone overlying the optic nerve or carotid artery (see Fig. 10 ) | The optic nerves indent or course through the sphenoid sinus in 24% of cases. Dehiscence of the optic nerves is also often associated with a pneumatized anterior clinoid. Bulging and dehiscence of the ICA is seen in 4% of individuals and this should also be documented to prevent surgical complications. |
Onodi (sphenoethmoidal) cell (see Fig. 10 ) | This posterior ethmoid air cell extends superior to the sphenoid sinus. The surgeon may anticipate that the posterior wall of this air cell will access the sphenoid sinus; however, it will potentially breach the optic nerve canal. |
Protrusion and dehiscence of the vidian and maxillary nerves (see Fig. 10 ) | Extension of the sphenoid sinus between the vidian canal and foramen rotundum occurs in 40% of sinuses, with nerve protrusion and dehiscence having been associated with iatrogenic injury. |
Median Anterior Skull Base: Applied Anatomy
The median ASB is formed by the paired orbital plates of the frontal bone, the cribriform plate of ethmoid, and the lesser wing of sphenoid (see Fig. 2 ). Unlike the lateral ASB, which forms the roofs of the orbits, the median ASB it is directly related to the sinonasal region.
The orbital plate of frontal bone contributes to the ipsilateral posterior wall of frontal sinus and the fovea ethmoidalis (or roof of ethmoid). It generally consists of resistant compact bone, although there are multiple diploic valveless veins that traverse its walls with the potential to transmit infection. A large frontal sinus (pneumosinus) should be distinguished from abnormal expansion that encroaches on other structures, which is termed a pneumosinus dilatans (with a normal thickness wall) or a pneumocele (with thinned or deficient wall) ( Fig. 3 ). The fovea ethmoidalis slopes inferiorly at an angle of 15° as it extends posteriorly to the planum sphenoidale.
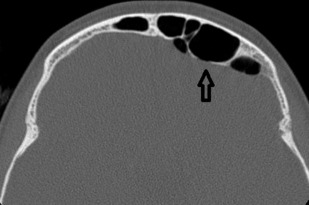
The fronto-cribriform suture represents the junction between the fovea ethmoidalis and the more medial cribriform plate (or roof of the nasal cavity). These structures converge at the vertically orientated lateral lamella of the cribriform plate ( Fig. 4 ). Sinonasal variant anatomy predisposes to surgical ASB injury. The Keros classification grades the length of the lateral lamella, with the longest (>7 mm or type III) present in 8% of individuals and most prone to surgical injury. The lateral lamella is attached to the vertical lamella of the middle turbinate and this may be damaged by turbinate manipulation (see Fig. 4 ; Fig. 5 ). The lateral lamella is also traversed by the anterior ethmoidal artery and this is a site of particular bony weakness (see Figs. 4 and 5 ). The cribriform plate itself is a delicate structure, which is susceptible to injury and erosion. It demonstrates multiple perforations through which pass the olfactory nerves. Small defects in the cribriform plate and lateral lamella are a normal finding on CT. The olfactory nerves form a potential pathway for tumor spread as they extend superiorly to the olfactory bulbs (see Fig. 4 ). The bulbs sit on the cribriform plate and beneath the frontal lobes with decreased bulb volume noted in patients with congenital or acquired olfactory dysfunction (see Fig. 4 ). The dura is tightly adherent to the bone of the cribriform plate and ethmoid roof, so it is often disrupted by adjacent fractures and is also prone to tumor invasion.
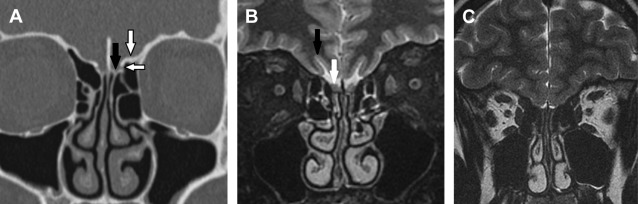
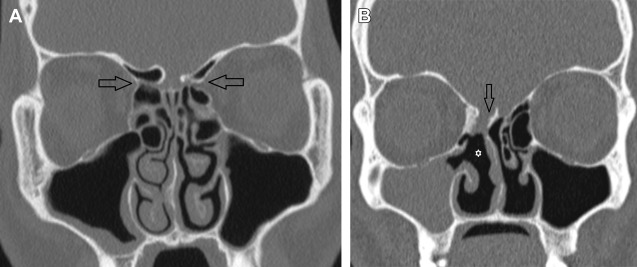
The midline portion of the ASB is largely unossified at birth, with variable rates of subsequent ossification ( Fig. 6 ). It may be judged to be complete on CT at between 14 months and 4 years. The crista galli is the triangular process of the ethmoid on the superior surface of the cribriform plate, which attaches the dura of the anterior falx (see Fig. 2 ). T1W high signal from the yellow marrow of the crista galli should not be misinterpreted as pathology, such as a dermoid, on MR imaging. The foramen caecum is located just anterior to the cribriform plate and crista galli at the frontoethmoid suture (see Fig. 2 ). It corresponds to the site of a temporary dural diverticulum, which extends to the nasal bridge in embryogenesis and hence it is the origin of various ASB developmental anomalies. The foramen caecum is usually 3 mm or less in size in children and 2 mm in adults. It is usually blind ended; however, it may transmit an emissary vein. A wide foramen caecum (see Fig. 6 ) and bifid crista galli may, but does not necessarily indicate that a midline developmental lesion extends intracranially.
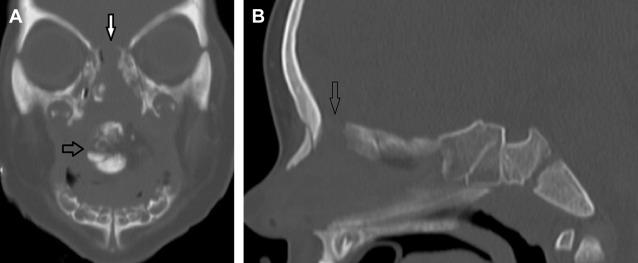
Median Central Skull Base: Applied Anatomy
The sphenoid sinus is contained within the body of the sphenoid bone, which is the principle median structure of the CSB. It variably occupies the midline sagittal compartment of the CSB, which is formed by the body of sphenoid, including the upper clivus (basisphenoid), and is bordered laterally by the petro-occipital fissures ( Fig. 7 ). The sphenoid largely consists of medullary bone, which makes it a focus for hematogenous spread of pathology. The MR imaging signal of the basisphenoid marrow varies considerably. Although more homogenously increased T1W signal yellow marrow is seen with age, 30% to 60% of healthy adults demonstrate heterogenous signal in the basisphenoid marrow. A more focal benign variation in the imaging appearances of the bone adjacent to the sphenoid sinus is seen with arrested pneumatization ( Fig. 8 ). It is proposed that this is a consequence of failed sinus formation, despite preparation for pneumatization by earlier marrow conversion. Foci of T1W high signal (fatty) and T1W low-signal/T2W high-signal (microcystic) change are seen on MR imaging, with characteristic curvilinear calcification and fat density, but without expansion or foraminal distorsion demonstrated on CT (see Fig. 8 ).
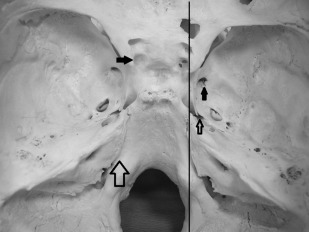
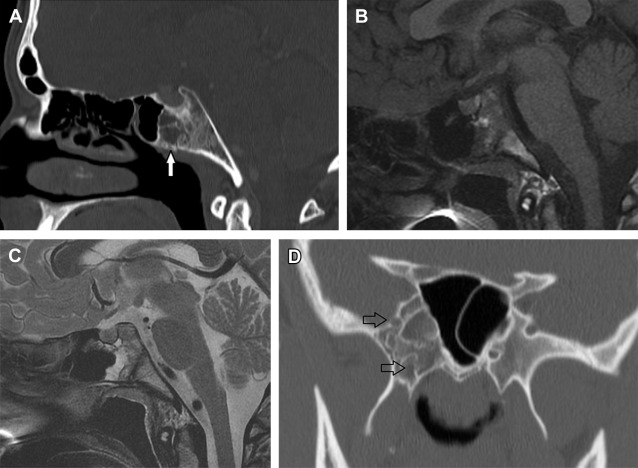
A corticated defect extending from the floor of the sellar within the central sphenoid bone is thought to be associated with a Rathke pouch remnant and has been termed the craniopharyngeal canal . This may be an incidental variant when small, or may contain ectopic adenohyphysis, cephaloceles, and tumors when larger ( Fig. 9 ). Sphenoid ossification occurs in numerous centers and is not complete until the spheno-occipital synchondrosis fuses at 12 to 16 years (see Fig. 9 ). Pneumatization of the sphenoid progresses inferiorly and posteriorly. It develops most rapidly between the ages of 1 and 5 and is generally thought to reach its full extent by age 12 to 14, although it may continue until adulthood. Pneumatization is most frequently postsellar in configuration, whereby it extends below or more posterior to the sellar floor and hence is directly related to the inferior pituitary gland. Other forms of presellar (10%–25%) or rudimentary conchal-type (2%) pneumatizations are less frequent but less favorable for transsphenoidal surgery.
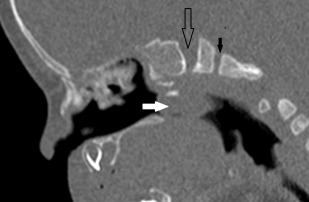
There may be further challenges to sphenoid sinus surgery posed by the considerable variation in the configuration of intersphenoid sinus septations. Although a simple configuration is present in a majority of individuals, up to 30% of sphenoid sinuses demonstrate 2 or more asymmetric or additional horizontal septae ( Fig. 10 ). When a horizontal septation is demonstrated, the radiologist should consider whether this represents an Onodi (sphenoethmoidal) cell extending superior to the sphenoid sinus, because this is a surgically important variation (see Fig. 10 ).
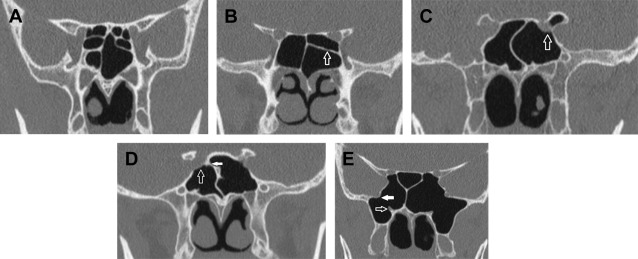
The lateral walls are thin bony layers that are related to the orbits and superior orbital fissures anteriorly as well as the optic nerves, medial cavernous sinuses, and internal carotid arteries (ICAs) posteriorly. Dehiscence of the optic nerves may predispose to inflammatory complications or iatrogenic injury (see Fig. 10 ). A further critical lateral relationship of the sphenoid sinus is the neurovascular structures of cavernous sinus, which may be a source, pathway of spread, or a target for paranasal sinus pathology. The cavernous carotid artery and the VI nerve are contained within the cavernous sinus itself, whereas the III, IV, VI, and V2 nerves are interposed between the inner and outer dural layers along its lateral wall. Foci of fat density and signal within the cavernous sinuses are more common in obese patients or those with high corticosteroid levels. Variable contrast opacification on CT venography studies may also be a normal imaging feature, depending on the relative inflow of the ophthalmic veins, pterygoid plexi, and sylvian veins.
When there is extensive anterolateral sinus pneumatization, the sphenoid sinus may also occupy the greater wing of sphenoid and pterygoid base, abutting the structures of the parasagittal CSB compartment. Sternberg’s canal (or lateral craniopharyngeal canal) is a potential bony defect, resulting from incomplete fusion between the basisphenoid and greater wing of sphenoid. It has been proposed to be the origin of cephaloceles, which extend into the lateral recesses of the sphenoid sinus, although this has been disputed. Extension of the sphenoid sinus between the vidian canal and foramen rotundum (containing the maxillary nerve) occurs in 40% of sinuses, with nerve protrusion and dehiscence having been associated with iatrogenic injury and neuritis secondary to sphenoid sinusitis (see Fig. 10 ).
Sinonasal pathology extending to the skull base
Neoplastic
Malignant
Imaging may depict the intracranial extension of malignancy, either directly through the bone of the skull base or via foramina and fissures ( Table 2 ). It helps predict the involvement of structures, such as the dura, cisternal cranial nerves, brain parenchyma, cavernous sinus, and carotid artery, and thus plays a critical role in the staging, treatment planning, and prediction of clinical outcome (see Table 2 ).
Imaging Appearance | Implications | Pitfalls in Imaging Assessment |
---|---|---|
| Endoscopic resection with reconstruction or craniofacial resection is required to obtain tumor clearance. |
|
| Indicates there is intracranial (extradural) extension of tumor and potential for dural invasion. Impacts on T staging. |
|
| Impacts on resectability and T staging | If no pregadolinium or fat-saturated postgadolinum T1W imaging is performed, then (enhancing) tumor may appear similar to the normal marrow signal and not be detected. |
| Impacts on T staging and outcome. Dural resection and reconstruction will be required. When dural invasion is extensive or extends laterally over the orbital roofs, then an open surgical approach is preferred to an endoscopic approach. | Dural inflammation may result in a thin rim of dural enhancement and should not be misinterpreted as tumor invasion. |
| Impacts on T staging and radiotherapy planning and it is important for prognosis (with a reduction in the local control). |
|
| Impacts on T staging, resectability, and outcome | Resectability depends on tumor histology and local surgical protocols. |
Sinonasal carcinomas are the most frequent malignant tumor and are usually of advanced stage at the time of diagnosis. The importance of skull base involvement by carcinoma is reflected by the TNM classification, which stages such maxillary and nasoethmoid carcinomas as T3 or T4 ( Table 3 ). Skull base or intracranial extension are also features of staging systems for other malignant neoplasms, such as olfactory neuroblastoma (Kadish stage C) and melanoma (T4).
Imaging Feature | T Stage |
---|---|
Invading the bone of the cribriform plate | T3 nasoethmoid carcinoma T4a maxillary carcinoma |
Minimally invades the anterior cranial fossa | T4a nasoethmoid carcinoma |
Invading the dura, brain, clivus, and middle cranial fossa | T4b nasoethmoid and maxillary carcinoma |
Direct extension
Direct skull base extension of malignant tumor usually involves the ASB and is most frequently seen in the context of squamous cell carcinoma or sinonasal undifferentiated carcinoma (SNUC). When such patients undergo surgery, they require endoscopic resection with reconstruction or craniofacial resection to gain tumor clearance. The radiologist must first assess whether there is contact of tumor with the skull base, which requires the intrasinus delineation of tumor from inflammatory changes or sinonasal secretions ( Fig. 11 ). Fortunately, most malignant high-grade tumors tend to be intermediate T2W signal due to the high cellularity, and they may be delineated from the T2W hyperintensity of inflammatory change and secretions (see Fig. 11 ), although there are pitfalls (see Table 2 ).
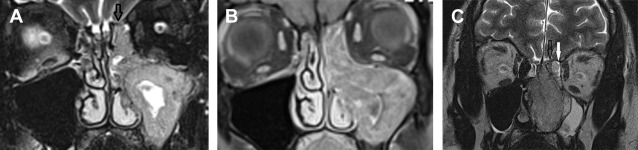
Having established skull base contact, it is important to determine whether there is direct extension through the bone and infiltration of the dura or brain. The breaching of the cortical bone of the ASB is well shown by CT ( Fig. 12 ). MR imaging is effective in demonstrating both the cortical bone and the periosteum of the skull base, which are seen together as a hypointense interface on all sequences. The nonmineralized periosteum is potentially more important because it may demonstrate greater resistance to tumor spread, and this layer is only visualized on MR imaging. The interruption of this hypointense line implies there is intracranial (extradural) extension of tumor and the potential for dural invasion ( Fig. 13 ).
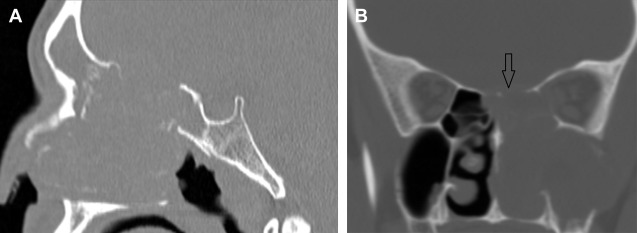
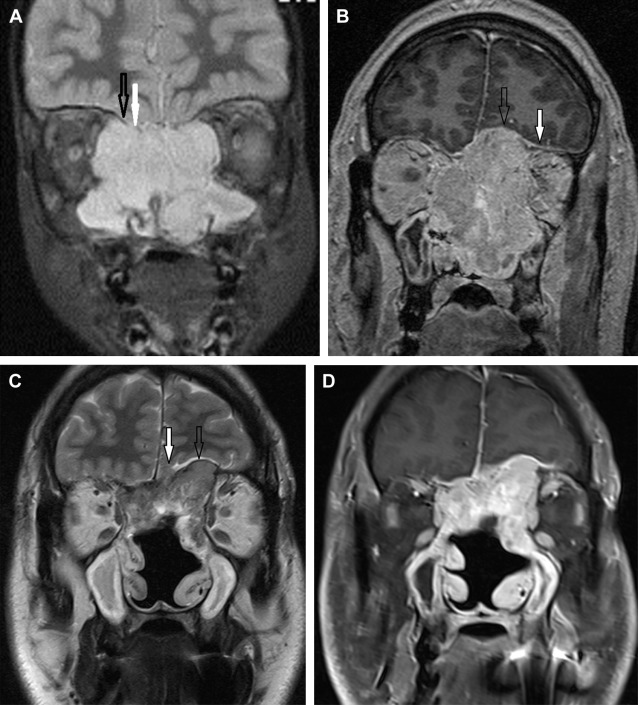
Dural tumor infiltration must be distinguished from reactive dural inflammation. To achieve this, MR imaging criteria for dural invasion are proposed. These include the presence of dural nodularity, marked dural thickening (which is variably stated as >2 mm or >5 mm) (see Fig. 13 ), or leptomeningeal enhancement. The presence of dural involvement has implications for treatment planning, because it requires resection and reconstruction to prevent CSF leak and generally requires postoperative radiotherapy. When dural invasion is extensive, or it extends laterally over the orbital roofs, then an open surgical approach is preferred to an endoscopic approach. There is also an impact on outcome with a reduction in disease specific and overall 5-year survival.
There may be further direct intracranial extension to involve the brain, which is characterized on MR imaging by effacement of the CSF signal and parenchymal edema ( Fig. 14 ). Limited brain invasion may be addressed with craniofacial resection with a nonsignificant decrease in survival compared with dural invasion alone. More extensive frontal lobe involvement by high-grade malignancy usually precludes surgical treatment and portends a poor outcome. Lower-grade malignant tumors, such as olfactory neuroblastoma, may be suitable for surgical intervention despite extensive intracranial involvement. The intracranial extension of olfactory neuroblastoma is characterized by peritumoural cysts on imaging studies ( Fig. 15 ).
