6 Thrombolysis Most strokes occur when a clot occludes an artery that supplies the brain. This underlies the rationale of administering a clot-dissolving (thrombolytic) agent that can reduce the damage from ischemic infarction by restoring blood flow. This is most likely to be successful when blood flow is re-established shortly after the occlusion. The danger of this therapy is that it may lead to hemorrhage in brain areas that have been softened due to ischemia. As of 1992, ~700 patients had been treated by intravenous thrombolysis in six randomized studies. A Cochrane Review published in 2003 covered 5727 patients treated with thrombolytic agents (Wardlaw et al. 2003), and the most recent Cochrane Review (Ward-law et al. 2009) covered 7152 patients. Most patients had been treated within 6 h from the onset of their stroke. The most widely used agent, approved for use in the United States, Canada, and most European countries, was rtPA (alteplase). The thrombotic occlusion of a cerebral artery may be embolic or may result from local thrombosis due to endothelial damage, platelet activation and aggregation, and thrombin formation. The complex coagulation system has four main components: • Endothelium • Soluble and cellular components of the coagulation and fibrinolytic system • Cellular blood constituents, especially platelets • Blood flow These four components interact in an tight-knit fashion to regulate coagulation (Preissner 2004). The role of the endothelium is to shield potentially thrombogenic vascular contents from the environment, so its basic function is to prevent coagulation and maintain unrestricted flow. Activated platelets can bind only to endothelium that is not fully intact. Figure 6.1 shows the processes that are associated with intact and damaged endothelium. Important properties of the endothelium that can initiate and reinforce clotting are the formation and secretion of plasminogen activator inhibitor-1 (PAI-1), initiation of the endothelial factor VII-tissue factor complex, the formation of von Willebrand factor, and platelet adhesion. Conversely, endothelium in an intact state has considerably more antithrombotic properties such as thrombomodulin formation, protein C activation, antithrombin binding, and the formation and secretion of prostacyclin, which inhibits platelet aggregation. Fig. 6.1 Graphic representation of coagulation cascades in the case of intact endothelium (left) and damaged endothelium (right; after Hamann). AT III, antithrombin III; Cact, protein C activator; T, thrombin; tPA, tissue-type plasminogen activator; PGL2, prostacyclin; Va, VII, VIIa, VIII:c, IXa, X, Xa, and protein C, coagulation factors. • Exogenous system: This is the system by which platelets aggregate at the site of an endothelial defect and form a thrombus (clot). Extravascular tissue factor from subendothelial cells forms a calcium-dependent complex with factor VII or VIIa, which activates factor VII as well as factors IX and X. The factors Xa and Va can form a calcium-dependent complex that activates factor II to IIa—that is, converts prothrombin to thrombin. This step is called initiation. The activation of factor IX by the tissue factor VIIa complex is an amplification mechanism that serves to increase thrombin formation (the “Josso loop”). The resulting thrombin can now activate factor XI to XIa, which creates another mechanism, called propagation, that can increase thrombin generation. Thrombin activates factors V, VIII, and XIII and is also a potent platelet activator. It converts fibrinogen to fibrin, splitting off the fibrin peptides A and B. Even small amounts of thrombin can convert very large amounts of fibrinogen to fibrin. • Endogenous system: This is an in-vitro phenomenon. Nevertheless, it is useful to recognize the endogenous system because one of the most widely used clinical tests, the activated partial thromboplastin time (aPTT) test, covers this part of the coagulation system and exploits the fact that factor XII can be activated by contact with nonphysiologic foreign surfaces (Hamann 2010). Fibrin formation is accompanied by a simultaneous activation of the fibrinolytic cascade. The endothelial cells mainly secrete tissue-type plasminogen activator (tPA), which binds to fibrin and forms a fibrin-tPA-plasminogen complex. One result of this process is the formation of plasmin from plasminogen. Plasmin splits fibrin and dissolves temporary clots. The resulting cleavage products lead to an increased interaction between plasminogen and tPA, stimulating the formation of Lys-plasminogen, which is more fibrin-specific. Plasmin also initiates the formation of the activated two-chain form of tPA. Dissolution of the clot destroys the platelets contained in the clot, causing them to release PAI-1. Extracellular fibrinolysis stimulates neoangiogenesis and tissue remodeling (Hamann 2010). • tPA: tPA is a single-chain glycosylated serine protease with a molecular mass of 70 kDa. The molecule has four different domains: a finger (F) domain, an epidermal growth factor (E) domain, a two-kringle domain (K1 and K2), and the serine protease domain. The C-terminal serine protease domain has an active site where plasminogen cleavage can occur. The two-kringle domain of tPA resembles the plasminogen domain. The finger domain and K2 domain are responsible for binding to fibrin. Single-chain tPA can be converted by plasmin cleavage to a double-chain form, which is also active. The plasma half-life of the single- and double-chain forms of tPA is ~3–8 min, averaging 3.5 ± 1.4 min. tPA is naturally released by endothelial cells, neurons, astrocytes, and microglia. It is broken down in the liver. tPA is classified as a “fibrin-specific” thrombolytic because it activates plasminogen most effectively when it is bound to fibrin. Desmopressin can raise the blood levels of tPA, while heparin and heparan sulfate can increase its activity. Today a single-chain form of tPA is produced by recombinant technology and is marketed under the generic name alteplase. • Urokinase: Urokinase is a plasminogen activator whose precursor prourokinase is formed in renal endothelial cells and by malignant cells. Single-chain prourokinase binds fibrin-selective plasminogen. Prourokinase can be produced by recombinant technology. The cleavage of prourokinase yields a heavy double-chain urokinase molecule with a molecular mass of 54 kDa, which is split further into a low-molecular-weight (31 kDa) urokinase activator. Both the high- and low-molecular forms are enzymatically active and convert plasminogen to plasmin. Their plasma half-life is ~9–12 min, similar to that of rtPA. These compounds are typically derived from nonhuman tissues or cells. • Streptokinase: The best-known exogenous plasminogen activator, streptokinase is a single-chain polypeptide derived from phemolytic streptococci. It binds plasminogen and activates circulating plasminogen to plasmin. In the process, it is transformed into a streptokinase-plasmin complex. This complex can then be inactivated by α-2-antiplasmin. Previous strep-tococcal infections may have stimulated the formation of antibodies that can neutralize streptokinase and reduce its efficacy. Streptokinase has a plasma half-life of 4 min, and its complex has a second half-life of 30 min. • Anisoylated plasminogen-streptokinase activator complex (APSAC): This agent consists of plasminogen non-covalently bound to streptokinase. APSAC has a half-life of 70 min, which is longer than that of tPA. It has been used in the treatment of myocardial infarction. • Desmoteplase: This agent is a recombinant plasminogen activator produced from the saliva of the vampire bat (Desmodus rotundus). Its α form is even more fibrin-selective than tPA. It has a longer half-life and appears to be the most potent plasminogen activator now available. • Staphylokinase: This enzyme is derived from Staphylo-coccus aureus. Its fibrin specificity is low. • Tenecteplase: This is the primary thrombolytic agent now used in the treatment of myocardial infarction and pulmonary embolism. It is a mutant of tPA with a longer half-life of ~17 ± 7 min, higher fibrin specificity, higher resistance to PAI-1, and greater lytic activity on clots. Microplasmin is a new thrombolytic agent that has a special role because it consists only of the active portion of the plasmin molecule. This enables it to dissolve blood clots directly rather than by plasminogen activation. Its efficacy therefore does not depend on the local abundance of plasminogen. There are significant differences in the coagulation physiology of different animal species, and between animals and humans. As a result, we must be very careful when applying the results of animal studies to clinical medicine. As early as 1963, Meyer et al. used cats and monkeys as experimental models for investigating the intravenous or intra-arterial administration of bovine or human plasmin in stroke (Meyer et al. 1963). These agents dissolved blood clots with a high rate of intracerebral hemorrhage. In 1985, Zivin et al. showed that tPA could improve neurologic outcomes following embolization with artificially produced clots (Zivin et al. 1985). In the following year, del Zoppo et al. found that 3 h after reversible balloon compression of the MCA in baboons, the intracarotid infusion of urokinase improved neurologic outcome and reduced infarct size without evidence of significant intracerebral hemorrhage (del Zoppo et al. 1986). In 1987, Slivka and Pulsinelli observed that both tPA and streptokinase, when administered 24 h after experimentally induced stroke in rabbits, increased the rate of intracerebral hemorrhage (Slivka and Pulsinelli 1987). They also found that administering the agent early after the occlusion did not increase the risk of hemorrhage. On the other hand, a 1989 study by Lyden et al. showed that rtPA did not increase the incidence of cerebral hemorrhage in rabbits, regardless of when it was administered (10 min, 8 h, or 24 h after cerebral embolism; Lyden et al. 1989). Lyden et al. later compared different thrombolytic agents—tPA, streptokinase, and saline as a control—in the rabbit embolic model (Lyden et al. 1990). They found that streptokinase, but not rtPA, caused a significant increase in the rate and size of intracerebral hemorrhages. rtPA was administered at 90 min in three doses (3, 5, and 10 mg/kg body weight) whereas streptokinase was administered in a dose of 30 000 U/kg at 5, 90, and 300 min. No clear dose dependence was observed, but results varied with the agent used: streptokinase was significantly less effective than rtPA. Interestingly, these investigators already noted the fact that when tPA did dissolve the clot, it resulted in an incidence of cerebral hemorrhage twice high as the placebo. This finding led to the conclusion that reperfusion was responsible for the higher rate of hemorrhagic transformation. In 1991, Clark et al. showed that aspirin and tPA acted synergistically to cause intracerebral hemorrhage in a rabbit embolism model (Clark et al. 1991). It was later found that tPA in a rat model caused microvascular damage at high doses (comparable to human use) but caused significantly less damage at lower doses (Burggraf et al. 2003). Tenecteplase appears to be associated with higher hemorrhage rates in animal studies, whereas microplasmin appears to be better tolerated when used in neural tissue (Burggraf et al. 2010). Note In summary, experimental studies found that tPA had a better safety profile than other thrombolytic agents, especially streptokinase. It was safe when administered early after the occlusion. Cerebral hemorrhage was most strongly associated with recanalization and later administration. The initial results of attempts to introduce thrombolysis as a treatment option for acute stroke were extremely discouraging. This applies particularly to studies that were done in the pre-CT era. Besides suffering from a lack of imaging options to exclude intracerebral hemorrhage, these studies also employed a significantly longer time window. In 1965, Meyer et al. conducted a study in 73 patients with acute progressive strokes (Meyer et al. 1965). The treatment group received streptokinase + heparin, whereas the control group received heparin only. There were significantly more deaths in the treatment group, and the control group had better outcomes. In 1976, Fletcher et al. studied 31 patients with acute stroke who received three different doses of intravenous urokinase or a placebo (Fletcher et al. 1976). The time window for these cases was 36 h. The study showed that urokinase could be administered without causing serious deficits. The number of patients was very small; the mortality rate was 16%. In the wake of these two studies by Meyer et al. and Fletcher et al., thrombolysis was considered unsuitable for the treatment of strokes. Once the safety and efficacy of tPA had been documented in animal models, del Zoppo et al. conducted a study in 193 patients with acute is-chemic stroke who received different doses of rtPA 8 h after the onset of stroke symptoms (del Zoppo et al. 1992). Angiography documented the occlusion of an extra- or intracranial cerebral artery in all patients. Exclusion criteria were a minor deficit, transient ischemic attacks, severe stroke with hemiplegia, decreased level of consciousness, gaze deviation, blood pressures higher than 200/120 mmHg, and CT evidence of hemorrhage or mass effect with a midline shift. Patients with early CT changes were not excluded from the study. The primary end point was the angiographic detection of recanalization and/or intracerebral hemorrhage with neurologic deterioration. This landmark study documented the clinical possibilities of thrombolysis. Approximately 40% of all patients achieved recanalization of the occluded artery. There was no relationship between dose and recanalization. Patients with smaller clots (i.e., distal vascular occlusions) showed the best recanalization rates. The incidence of all possible hemorrhagic changes was 30.8%. Symptomatic hemorrhages occurred in 9.6% of the patients, and the mortality rate was 12.5%. Hemorrhagic transformation did not increase at the dose that had been used for coronary thrombolysis. This led the authors to conclude that the dose of rtPA used for coronary thrombolysis was also the perfect dose for the treatment of acute stroke. The first NINDS study was published in 1992 (Brott et al. 1992). In a dose-escalation study design, 74 patients with acute ischemic stroke were treated with incremental doses of rtPA (0.35–1.08 mg/kg body weight) within 90 min of symptom onset. Intracerebral hemorrhage did not occur in any of the 58 patients treated with ≤ 0.85 mg/kg rtPA. Intracranial hemorrhage occurred only at higher doses. Hemorrhage with neurologic deterioration (symptomatic hemorrhage) occurred only in patients treated with rtPA at doses ≥ 0.95 mg/kg. Significant neurologic improvement occurred in 30% of the patients at 2 h from the start of treatment and in 46% at 24 h. Clinical neurologic improvement was not found to be dose-dependent. The investigators concluded that the highest possible safe dose of rtPA was probably < 0.95 mg/kg. However, this conclusion was ultimately based on just three symptomatic hemorrhages that occurred in a total of 74 patients, so it was possible that a higher dose could be both safe and more effective. In the same year, Haley et al. (1992) conducted another dose-escalation study in 20 patients with acute ischemic stroke who received rtPA between 91 and 180 min after symptom onset. The risk of symptomatic intracerebral hemorrhage was stated as 10% overall and 17% in the two higher-dose groups. Three different doses were used: 0.6, 0.85, and 0.95 mg/kg body weight. Three patients had improved by at least 4 points at 24 h. That same year, Mori et al. (1992) did a study to determine whether rtPA was safe when administered intravenously at a dose of 6 or 12 million units within 6 h after symptom onset. Angiograms were obtained before and after thrombolytic therapy. These Japanese researchers confirmed that rtPA improved recanalization rates. The functional outcome (Barthel score) also showed significant improvement. This study, like the study by del Zoppo et al. (1992), confirmed the capability of rtPA to open occluded vessels. The Bridging Trial was published in 1993. Haley et al. investigated 27 patients who had received 0.85 mg/kg intravenous rtPA or a placebo within 3 h after stroke onset (Haley et al. 1993). The study was a randomized, double-blind, placebo-controlled trial. Despite its small size, the study showed that patients treated within 90 min from symptom onset did show neurologic improvement at 24 h. Six of 10 patients treated with rtPA within 90 min improved by 4 or more points on the NIHSS at 24 h, compared with just one patient in the placebo group. In the groups that were treated at 91–180 min after onset, two patients in the rtPA group improved at 24 h, and two improved in the placebo group. Published in 1995, the European Cooperative Acute Stroke Study (ECASS I) study included 620 patients treated with 1.1 mg/kg body weight of intravenous rtPA or placebo within 6 h from stroke onset (Hacke et al. 1995). This study found no significant efficacy of intravenous thrombolysis in an intention-to-treat analysis. The 109 patients with protocol violations (17.4% of all patients) were excluded, leaving a target population of 511 patients. The protocol violations consisted mainly of including patients with major strokes (> 1/3 hypodensity of MCA territory on CT) who were taking anticoagulants or hemodilutants, missed hemorrhages on initial CT, uncontrolled hypertension, and lack of follow-up. The target population showed a significant point difference in the mRS scale between the two treatment groups, with no difference in rates of intracerebral hemorrhage but with a higher rate of large parenchymal hemorrhages in the rtPA-treated patients. More hemorrhagic infarcts were documented in the placebo group. The mortality rates at 30 days were the same. The ECASS study also showed a significant difference when the NINDS global end point was applied. This was a post-hoc analysis, however, and the overall results of the study do not support a recommendation for routine intravenous thrombolysis in acute ischemic stroke patients. Also published in 1995 (National Institute of Neurological Disorders and Stroke rtPA Stroke Study Group 1995), the NINDS study was the first randomized, placebo-controlled multicenter study that showed the efficacy of rtPA in the treatment of acute stroke when administered within 3 h of symptom onset. The NINDS study differed from the ECASS study in several respects: perhaps the major difference was that the rtPA dose in the NINDS study was only 0.9 mg/kg. Moreover, the NINDS study was strictly controlled for blood pressure, which had to be less than 185/95 mmHg. The NINDS study had two parts with an identical protocol but different end points. Part 1 tested whether rtPA had clinical activity as indicated by an improvement of 4 points on the NIHSS or a complete resolution of neurologic deficits by 24 h. Part 2 assessed clinical outcome at 3 months according to scores on the Barthel index, mRS, Glasgow outcome scale, and NIHSS. The numbers of patients enrolled were 291 in Part 1 (144 in the rtPA group, 147 in the placebo group) and 333 in Part 2 (168 in the tPA group and 165 in the placebo group). In Part 1, 67 patients (47%) improved in the tPA group and 57 patients (39%) improved in the placebo group. This difference was not statistically significant (p < 0.21). In Part 2 of the study, assessing clinical outcome, it was found that the number of patients with good outcomes was higher in the tPA group than in the placebo group. The probability of having little or no disability at 3 months was 30–50% higher in the rtPA group. Specifically, the percentage of patients with a mRS of 0 or 1 at 3 months was 39% in the rtPA group vs. only 26% in the placebo group. This was a statistically significant difference in favor of rtPA. Symptomatic hemorrhage occurred in 6.4% of the rtPA-treated patients compared with only 0.6% of the placebo-treated patients. The mortality rate at 3 months was essentially the same in both groups (17% in the rtPA group vs. 21% in the placebo group). This means that, despite an increased risk of hemorrhage, treatment with rtPA did not affect mortality. Treatment with rtPA resulted in a more favorable prognosis. Interestingly, this effect did not depend on the subtype of stroke and applied equally to micro- and macroangiopathic strokes and cardioembolic strokes. Factors that were particularly associated with an increased risk of hemorrhage after rtPA treatment were a severe stroke, pronounced brain edema, or a midline shift. It was also found that patients treated with rtPA had at least a 30% greater likelihood of living independently at 1 year than patients treated with placebo. This improved outcome was not associated with increased disability or mortality. The rates of recurrent strokes at 1 year were the same in the tPA and placebo groups (Kwiatkowski et al. 1999). Note The NINDS study opened the window for the widespread use of rtPA in humans with acute ischemic stroke within 3 h after symptom onset. The ECASS II study (Hacke et al. 1998), like ECASS I, was a multicenter, double-blind randomized trial. It investigated 800 patients with acute stroke treated with 0.9 mg/kg of rtPA or a placebo within 6 h of stroke onset. The exclusion criteria were similar to the NINDS criteria but also included early infarct signs on CT involving more than one-third of the MCA territory, decreased level of consciousness, or hemiplegia with fixed deviation of gaze (conjugate deviation). Patients who had received anticoagulants or antithrombotic therapy during the first 24 h were also excluded. There were no significant differences in the rate of patients who had a good prognosis (mRS = 0–1 on day 90): 40.3% in the rtPA group and 36.6% in the placebo group. The rate of intracerebral hemorrhage was significantly higher in the rtPA group (8.8%) than in the placebo group (3.4%). Three studies investigated the use of streptokinase in acute stroke patients: the MAST-E, MAST-I, and ASK studies. MAST-E (Multicenter Acute Stroke Trial—Europe Study Group 1996) was a double-blind, placebo-controlled randomized study. Patients were treated with 1.5 million units of intravenous streptokinase or placebo within 6 h. No difference was found in the primary outcome parameters (death or severe disability). More patients died in the streptokinase group, which also showed an increased incidence of symptomatic intracerebral hemorrhage. Approximately 60% of the streptokinase patients and 75% of the placebo patients also received heparin, and 20% in each group received aspirin. Assessment at 6 months showed a small, not statistically significant proportion of deaths in the streptokinase group plus a trend toward less disability in that group. In the ASK study (Australian Streptokinase Study; Donnan et al. 1996), 340 patients randomized from various centers were treated with intravenous streptokinase or a placebo within 4 h of stroke onset. The study was terminated early due to the poorer outcomes in the streptokinase-treated patients. Intracerebral hemorrhage occurred in 9.6% of the patients in the streptokinase group and none of the patients in the placebo group. The streptokinase patients had a higher incidence of hypotension and a significantly higher mortality rate. Note All three studies supported the conclusion that streptokinase is not indicated in the treatment of acute stroke. The ATLANTIS study (Alteplase ThromboLysis for Acute Noninterventional Therapy in Ischemic Stroke; Clark et al. 1999) was a double-blind randomized study that tested the safety and efficacy of 0.9 mg/kg body weight intravenous rtPA in patients within 6 h of symptom onset. The time window was later shortened to 5 h. The study was terminated early when an interim analysis showed that it was unlikely that any benefit would be derived from rtPA. There was no difference in the primary end point. As expected, the rate of symptomatic intracerebral hemorrhages was higher in the rtPA-treated group. A post-analysis of patients treated with rtPA within 3 h of stroke onset showed that those patients were more likely to have a favorable outcome (60.9% in the rtPA group vs. 26.3% in the placebo group). This difference was statistically significant, but it was based on a post-hoc analysis. Symptomatic intracerebral hemorrhage occurred in 13% of the rtPA-treated patients and none of the placebo-treated patients. The ECASS III study (Hacke et al. 2008b) tested the efficacy and safety of alteplase in the treatment of acute stroke within a 3- to 4.5-h time window after symptom onset. A total of 821 patients were enrolled in the double-blind study and were randomly assigned to receive intravenous alteplase (0.9 mg/kg body weight) or a placebo. The primary end point of the study was a good treatment outcome defined as an mRS of 0 or 1, in contrast to a poor outcome (mRS = 2–6). The secondary end point was a global outcome analysis of four neurologic and disability scores. Safety end points included death and symptomatic intracerebral hemorrhage. Of the 821 stroke patients studied, 418 were assigned to the alteplase group and 403 to the placebo group. The median time to alteplase administration was 3 h 59 min. Approximately 10% of the patients were treated in the time window from ≥ 3 to ≤ 3.5 h, 46% from > 3.5 to < 4 h, and 40% from > 4 to ≤ 4.5 h. Significantly more patients treated with alteplase had a good outcome at 90 days (mRS = 0 or 1; 54% vs. 45.2%; OR 1.34; 95% CI 1.02–1.76; p = 0.04). A global outcome analysis also showed a benefit of alteplase therapy (OR 1.28; 95% CI 1.00–1.65; p < 0.05). The incidence of any intracerebral hemorrhage and symptomatic intracerebral hemorrhage was significantly higher in the alteplase group (any intracerebral hemorrhage: 27.0% vs. 17.6%; p = 0.001; symptomatic intracerebral hemorrhage: 2.4% vs. 0.2%; p = 0.008). The mortality rates were the same in both groups (7.7% vs. 8.4%; p = 0.68). The ECASS III study (Bluhmki et al. 2009) joins the NINDS study of 1995 as the second large, randomized multicenter study to document the efficacy of rtPA in the treatment of acute stroke. Nevertheless, it should be noted that the treatment benefit achieved in the 3- to 4.5-h time window is moderate. The likelihood of a good treatment outcome not requiring help with daily activities increases by 28%, and the number of treatments necessary to achieve an mRS of 0 or 1 is 14. The overall risk of symptomatic intracerebral hemorrhage, while low and within expected limits (2.4% vs. 0.2%), was still significantly increased. On the positive side, the ECASS III study was a “true” 3- to 4.5-h study: 87% of the patients were treated within a time window of 3.5–4.5 h. Thus, the crucial question of efficacy in the latter part of the 3- to 4.5-h time window—a question not answered in the SITS registry (Safe Implementation of Treatments in Stroke)—has now been positively confirmed. Analysis by subgroups (patients grouped by age, sex, stroke severity, time to treatment, diabetes, hypertension, atrial fibrillation, smoking, or use of platelet inhibitors) showed no benefit differences in any of the subgroups (Bluhmki et al. 2009). It remains unclear why the 45% rate of good outcomes in the placebo group in ECASS III was ~6% higher than in the old NINDS study of 1995. Is this a stroke-unit effect or just a coincidence? Note Even in an expanded time window of 3–4.5 h, thrombolysis has been shown to be safe and effective. This does not mean, however, that we can take our time in treating patients for stroke. The cardinal rule still applies: Time is brain! In an initial multicenter open-label single-arm study (Yamaguchi et al. 2006), 103 patients in Japan received intravenous rtPA at a dose of 0.6 mg/kg body weight; 38% of the patients had a very good outcome with mRS = 0 or 1, and 6 symptomatic intracerebral hemorrhages occurred. The authors theorized that the lower dose of rtPA may be just as safe and effective in Japanese patients as the approved dose of 0.9 mg/kg. The J-ACT II study (Mori et al. 2010) investigated the efficacy and safety of 0.6 mg/kg body weight of rtPA administered within 3 h after onset of ischemic stroke symptoms in patients with an MCA occlusion confirmed by MRA. In the 58 patients studied, a recanalization rate of 52% was achieved by 6 h and 69% by 24 h. In all, 46.6% of the patients had a good clinical outcome (mRS = 0 or 1) after thrombolytic therapy, and no hemorrhages occurred. Recanalization at 6 h was an independent predictor of favorable outcome. No difference was found relative to treatment with 0.9 mg/kg of rtPA. No information is currently available on the follow-up of low-dose intravenous thrombolysis, and corresponding action recommendations cannot be made. Thijs et al. (2009) published the results of an intravenous microplasmin phase II study that had a double-blind, randomized, placebo-controlled design. The 40 patients in the study had been admitted between 3 and 12 h after an ischemic stroke: 10 of them received a placebo, 6 received microplasmin at a dose of 2 mg/kg body weight, 12 received 3 mg/kg, and 12 received 4 mg/kg. The therapy was well tolerated; only one fatal hemorrhage occurred. Microplasmin leads to the neutralization of α-2-antiplasmin in the serum. Clinical parameters were not significantly altered given the small case numbers, and currently there are no plans to conduct larger randomized efficacy studies with this agent. A desire for higher recanalization rates and lower rates of symptomatic intracerebral hemorrhage in an expanded time window beyond 3 h has prompted efforts to develop new thrombolytic agents. Desmoteplase is a fibrin-specific plasmin activator found to have an acceptable safety profile in earlier phase II studies—DIAS (Hacke et al. 2005 and DEDAS (Furlan et al. 2006)—also showing a trend toward higher reperfusion rates relative to placebo and greater clinical efficacy in a time window of 3–9 h. The DIAS II study (Hacke et al. 2009) was a phase III study aimed at confirming the results of DIAS and DEDAS, documenting safety and efficacy in patients with acute stroke, and detecting the presence of tissue at risk by diffusion- and perfusion-weighted MRI and CT perfusion (CTP). In a randomized, placebo-controlled, double-blind double-dose study, patients were randomly assigned to a group treated with 90 or 125 μg/kg of desmoteplase or placebo. One inclusion criterion was the presence of a 20% mismatch, i.e., visual estimation of a perfusion abnormality (penumbra) 20% larger than the diffusion abnormality. The primary end point was clinical improvement of ≥ 8 points on the NIHSS score or an NIHSS score of ≤ 1 point at 90 days, an mRS of 0–2 points, and a Barthel index of 75–100 points. Secondary end points were change in lesion volume between baseline and day 30, rate of symptomatic intracerebral hemorrhage, and mortality rate. In this study 193 patients were randomized, and 186 were treated: 57 received 90μg/kg desmoteplase, 66 received 125μg/kg, and 63 received placebo. The median baseline NIHSS score was 9 points, and 30% (53 of 179) of the patients had a vascular occlusion detectable by MRA. The core lesion and mismatch volumes were small (median initial infarct volume was 10 cm3, mismatch volume was 52 cm3). The clinical response rates did not differ significantly among the different groups. The changes in lesion volume over time were also similar. The rates of symptomatic intracerebral hemorrhage were higher in the treatment groups (3.5% and 4.5% vs. 0% for placebo). The mortality rate in the 125-μg group, at 21%, was markedly higher than in the low-dose and placebo groups. One-third of the patients were treated in the 3- to 6-h time window and two-thirds in the 6- to 9-h time window. The results of the DIAS II study are disappointing. It is interesting to analyze the study, however, because it suggests which patients may benefit from thrombolysis over an expanded time window of up to 9 h based on MRI or CT findings: • Patients with a large mismatch volume, i.e., a small diffusion abnormality and a large perfusion abnormality • Patients with a proximal vascular occlusion (M1 or M2 segment) • Patients who have had a mild stroke (NIHSS ≥ 14 with a proximal vascular occlusion) • Patients who recanalize These patients were in the minority in the DIAS II study. Only 30% of the patients had a vascular occlusion that was detectable by MR angiography (MRA) or CT angiography (CTA). The mismatch volume was ~80 cm3, meaning that only a small tissue volume was salvageable by recanalization. Another reason for the disappointing result was the MR and CT criteria. A mismatch volume of 20% of the tissue area with abnormal perfusion is too small and mainly encompasses oligemic tissue. Given this baseline condition, there was no reason to expect a significant benefit from reperfusion therapy. Criteria for effective thrombolysis in the expanded time window are a high recanalization rate and low rate of intracerebral hemorrhage. Desmoteplase was unable to meet these criteria in the DIAS II study. A clinical phase IIb study with tenecteplase was designed to define the optimum dose while demonstrating safety and efficacy in comparison with rtPA. The study in question was a multicenter, double-blind, randomized trial using tenecteplase doses of 0.1, 0.25, and 0.4 mg/kg body weight, which were compared with 0.9 mg/kg of rtPA. After the best dose was found, it was supposed to be used in treating 100 pairs of patients with tenecteplase and rtPA. The study was terminated due to slow recruitment and could not establish the effective dose (Haley et al. 2010). The high tenecteplase dose was associated with the highest bleeding rate, which was not unexpected on the basis of experimental data (Burggraf et al. 2010). It remains to be seen whether further studies on the agent will be forthcoming. “Mismatch” is the difference between the size and extent of the DWI lesion (diffusion abnormality) relative to the larger PWI lesion (perfusion abnormality). The DWI/PWI mismatch is useful for defining tissue at risk, i.e., tissue that is at risk for infarction but is still potentially salvageable by treatment. In a German multicenter study of 174 MRI-selected patients (Thomalla et al. 2006), 62% of the patients were treated with tPA within 3 h and 38% within 3–6 h on the basis of MRI mismatch findings. The outcome data were compared with pooled data from the ATLANTIS, ECASS, and NINDS trials. A good outcome was achieved in 48% of the MRI-selected tPA patients compared with 33% of pooled placebo patients and 40% of pooled tPA-treated patients. This indicates a slight superiority of MRI-based therapy. Safety was dramatically improved, however. The rate of symptomatic intracerebral hemorrhage was only 3% in the MRI-selected patients, compared with 8% in the pooled tPA group and 2% in the pooled placebo group. The Australian EPITHET study (De Silva et al. 2010) is an important study that investigated the efficacy of intravenous thrombolysis in stroke administered within a 3- to 6-h time window after symptom onset. Besides delayed thrombolysis, this study indirectly investigated the mismatch concept. While this concept seems logical and sound from a pathophysiologic standpoint, it has not been prospectively evaluated in selecting patients for thrombolytic therapy. In the EPITHET study, patients were selected by cranial CT. Afterward they were randomly assigned to rtPA or placebo groups, and then diffusion-weighted MRI was performed. Lesion size on baseline MRI was later compared with T2w MRI performed at 90 days. The primary end point was increase in lesion size between the two MRIs. Unfortunately, the primary end point was not significantly different between the two examinations, resulting in a negative study. While all the results tended to support the efficacy of thrombolysis, the benefit was not statistically significant. It is noteworthy that only a slight improvement was achieved in tPA patients with good outcomes (mRS = 0–3) (22% vs. 20% for all patients; 19% vs. 17% for mismatch patients). On the other hand, thrombolysis was associated with a nonsignificant but notable increase in the incidence of death or severe disability (18% vs. 9% for all patients; 15% vs. 8% for mismatch patients). A meta-analysis of MRI-based intravenous thrombolysis (Mishra et al. 2010) calls for more rigorous evaluation of the mismatch concept and a large, randomized confirmation study. Note Patient selection based on DWI/PWI mismatch by MRI allows for successful thrombolysis at 4.5 h, but further prospective studies are needed to investigate and confirm this concept. A meta-analysis (Lees et al. 2010) analyzed the individual data for rtPA-treated patients and placebo patients from clinical studies to determine the efficacy of this agent over time. This meta-analysis added the 821 ECASS III patients and the 100 EPITHET patients to the 2775 patients that had previously been pooled in the meta-analysis. The analysis included patients treated up to 6 h after stroke onset, yielding a total of 1850 rtPA-treated patients and 1820 placebo-treated patients. The earlier the patients were treated, the higher the percentage of patients with an mRS of 0 or 1 at 3 months. A benefit of intravenous thrombolysis was no longer seen by 270 min (4.5 h) after stroke onset (Fig. 6.2). Patients treated at 0–90 min had an OR of 2.55 (95% CI 1.44–4.52) for a favorable outcome at 3 months. Patients treated at 91–180 min had an OR of 1.64 (95% CI 1.12–2.40), and patients treated at 181–270 min had an OR of 1.34 (95% CI 1.06–1.68). These differences were significant. The OR at 270 min was 1.22 (95% CI 0.92–1.61), which was not statistically significant. Large symptomatic hemorrhages occurred in 96 of the alteplase-treated patients and 18 of the placebo patients. Mortality rates increased significantly with time from stroke onset to start of treatment (mean 95% CI 0.78 [0.41–1.48] for 0–90 min; mean 95% CI 1.13 [0.70–1.82] for 90–180 min; mean 95% CI 1.22 [0.87—1.71] for 180–270 min; mean 95% CI 1.49 [1.00–2.21] for 271–360 min). The authors concluded that the meta-analysis supported the efficacy of intravenous thrombolysis within a time window of 4.5 h, but that every effort should be made to shorten the delay in initiating treatment. Fig. 6.2 Dependence of the efficacy of intravenous thrombolysis on time to treatment. Thrombolysis is effective when started up to 4 5 hours after symptom onset (black bar) Odds ratio refers to the group with an mRS of 0—1 (after Lees et al. 2010). Intravenous thrombolysis is effective when started up to 4.5 h from the onset of acute stroke. Individual patients should be treated as soon as possible. In June 1996, the U.S. Food and Drug Administration (FDA) approved the use of intravenous rtPA at a dose of 0.9 mg/kg body weight when administered within 3 h after onset of an acute stroke. This regimen was approved in Europe in April 2002. Since then, there have been numerous observational studies documenting the use of thrombolysis in routine treatment settings. There is no need to review all of this research, but here we take a closer look at two of the larger studies: CASES and SITS-MOST. CASES (Canadian Activase for Stroke Effectiveness Study), published in 2005 (Hill et al. 2005), analyzed 1135 stroke patients treated with rtPA between February 1999 and June 2001. The mean NIHSS score was 15. Follow-up at 90 days showed that 30% of the patients had minimal or no neurologic sequelae and that 46% were independent. The rate of intracerebral hemorrhage was 4.6%. There was a 15% rate of protocol violations. The SITS-MOST registry (Safe Implementation of Thrombolysis in Stroke—Monitoring Study) compiled data from over 11 000 stroke patients treated by thrombolysis at more than 700 stroke units in 35 countries (Wahlgren et al. 2007). The registry, mandated by the European Medicines Evaluation Agency (EMEA) to provide more data on the safety and efficacy of thrombolysis within the 3-h time window, has become an important database for the everyday practice of thrombolysis. The SITS registry has not only confirmed the efficacy of thrombolysis but has shown a lower rate of intracerebral hemorrhages outside of study conditions (1.7% vs. 5.1%). Another important aspect revealed by the comparison of participating centers is the enormous gap between door-to-imaging times and door-to-needle times. Door-to-imaging times ranged from 9 to 90 min (averaging 28 min for all centers), while the door-to-needle times ranged from 20 to 139 min (average of 73 min). Short door-to-needle times are an important quality indicator, and ~30 min is a reasonable time goal with proper organization. One study used the SITS registry to assess the efficacy and safety of thrombolysis within the expanded time window of 3–4.5 h (Wahlgren et al. 2008). A total of 664 ischemic stroke patients that received thrombolysis from 3 to 4.5 h after stroke onset (average 195 min) were compared with 11 865 patients treated within 3 h (average 140 min). Study end points were the occurrence of symptomatic intracerebral hemorrhage detected by cranial CT, clinical deterioration by NIHSS ≥ 4 within 24 h, mortality at 3 months, and favorable outcome at 3 months defined as a score of 0–2 on the mRS. The 3- to 4.5-h group was 3 years younger on average, had less severe strokes (NIHSS = 11 vs. 12), and had lower rates of hypertension and hyperlipidemia. The mean treatment times differed by 55 min between the two groups (140 vs. 195 min), and 50% of the 3- to 4.5-h group were treated within 15 min past the 3-h time window. None of the end points differed significantly between the two treatment groups: the rate of symptomatic intracerebral hemorrhage was 2.2% vs. 1.6%, mortality rate was 12.7% vs. 12.2%, and favorable treatment outcome was 58% vs. 56.3%. It is beyond the scope of this chapter to review the ~50 publications dealing with single-center or pooled experience on the routine use of rtPA. Note Intravenous rtPA can be used in everyday practice with the same safety and efficacy as in large studies. It is reasonable to conclude that intravenous thrombolysis with rtPA in the 3-h and even 4.5-h time window can reduce deaths and severe disability by ~30%. Different guidelines offer different recommendations on intravenous thrombolysis. The guidelines stated below are the ones that are currently followed by the American Heart Association (Adams et al. 2007; del Zoppo et al. 2009): • The intravenous administration of rtPA is recommended within a 4.5-h time window for the treatment of ischemic stroke at centers experienced with this therapy (0.9 mg/kg body weight, maximum dose 90 mg, 10% of total dose given as initial bolus, the remaining 90% by infusion over 60 min). • Blood pressure should be less than 185/110 mmHg before and during thrombolytic therapy. Thrombolysis is generally contraindicated in patients with major strokes (NIHSS > 25) and extensive early ischemic changes, due to the risk of secondary cerebral hemorrhage. Thrombolysis cannot be recommended in patients with uncontrolled hypertension (blood pressure > 185/110 mmHg despite multiple treatment attempts). Guidelines in the United States (Adams et al. 2007) recommend intravenous thrombolysis with rtPA administered at 0.9 mg/kg body weight (maximum of 90 mg) over a 60-min period, with the initial 10% given by bolus injection within 3 h after symptom onset. Besides intracerebral hemorrhage, the risk of airway swelling and obstruction is noted as a potential serious side effect (Adams et al. 2007). Patients with epileptic seizures may receive thrombolysis if the treating physician believes that the residual symptoms are due to ischemia rather than Todd’s (postictal) paralysis. According to German approval criteria, the treatment may be administered only by a physician trained and experienced in critical care neurology. Other approval restrictions in Germany pertain to patients with a history of stroke and coexisting diabetes and a blood glucose level < 50 mg/dL or > 400 mg/dL. These restrictions are not adequately supported by study data, however. Labeling also cautions against treating patients > 80 years of age with intravenous rtPA, although several observational studies have shown that intravenous thrombolysis is also safe and effective in older patients. According to the Ad Hoc Committee representing the National Stroke Foundation and Stroke Society of Australasia (2009) and the American Heart Association (AHA) Statement on expanding the time window (del Zoppo et al. 2009), the following criteria should be applied in selecting patients for intravenous thrombolysis: • Treatment is initiated within 4.5 h from the onset of clinical symptoms. • A measurable, clinically significant neurologic deficit is present (NIHSS > 0). • Cranial CT shows no hemorrhage or other cause for the clinical symptoms. • The patient is over 18 years of age. Absolute contraindications to thrombolysis are as follows: • Unknown time of onset • Coma • Complete hemiplegia with fixed deviation of gaze • Rapid resolution of symptoms • Seizure at symptom onset • Blood pressure > 185 mmHg systolic and/or > 110 mmHg diastolic in repeated measurements despite therapy • Clinical suspicion of subarachnoid hemorrhage, even with negative cranial CT • Presumed septic embolism • Known bleeding diathesis, heparin administration in prior 48 h with increased partial thromboplastin time • Prothrombin time > 1.7 • Platelets < 100 000/μL • Blood glucose < 50 or > 400 mg/dL The following are relative contraindications that still allow for thrombolysis but only after detailed consideration and informed consent: • NIHSS > 22 • Age > 80 years • Hypodensities in cranial CT > 1/3 % of MCA territory • Stroke or head trauma in prior 3 months • Major surgical procedure in prior 2 weeks • Earlier subarachnoid hemorrhage, intracerebral hemorrhage due to angioma or other bleeding source • Myocardial infarction in prior 30 days • Needle biopsy of hollow viscus during prior 30 days with risk of uncontrolled bleeding • Trauma with internal injuries in prior 30 days • Gastrointestinal or other bleeding in prior 30 days • Arterial puncture in prior 7 days • Severe, untreatable comorbid condition that may jeopardize the success of thrombolysis (e.g., terminal cancer) The following factors predict a favorable outcome of intravenous thrombolysis, listed in order of descending importance (Demchuk et al. 2001): • Mild clinical severity • Absence of diabetes mellitus • Normal cranial CT • Normal blood pressure before treatment Note Always look for reasons to proceed with thrombolysis in stroke patients, not for reasons to withhold it.
Intravenous Thrombolysis
Introduction
Basic Principles of Coagulation Physiology
Coagulation
Fibrinolysis
Endogenous Plasminogen Activators
Exogenous Plasminogen Activators
Direct Thrombolytic Agents
Experimental Studies of Thrombolysis in Acute Stroke
Clinical Studies of Thrombolysis in Acute Stroke
First Efficacy Studies
Large Randomized Multicenter Placebo-Controlled Studies
ECASS I Study
NINDS Study
ECASS II Study
Streptokinase Studies
ATLANTIS Study
ECASS III Study
Japanese Studies Using a Lower rtPA Dose
Microplasmin Study
Desmoteplase Studies
Tenecteplase Study
MRI-Based Thrombolysis
Meta-analysis
Everyday Practice of Intravenous Thrombolysis in Acute Stroke
CASES Study
SITS-MOST Study
Guidelines
Practical Recommendations
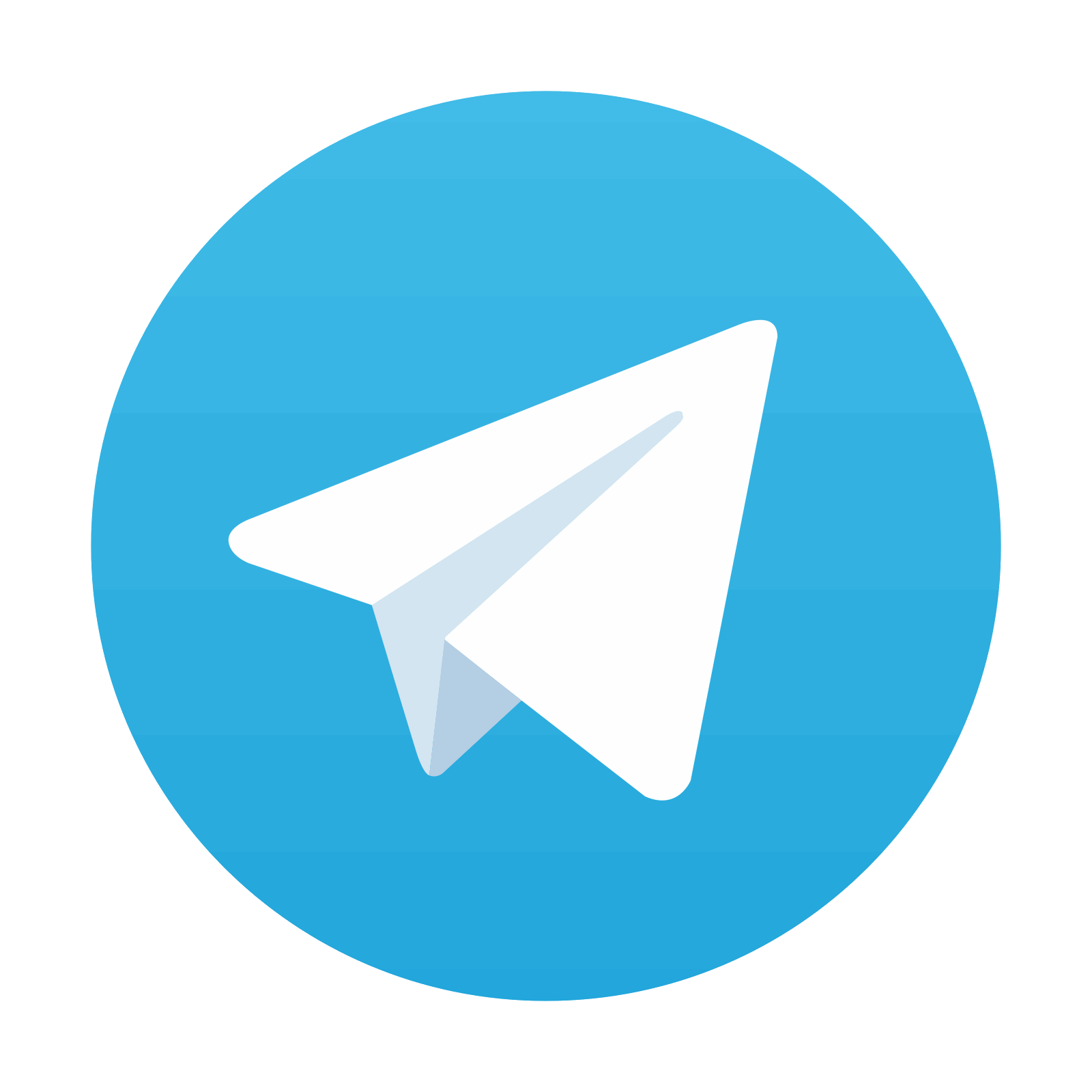
Stay updated, free articles. Join our Telegram channel

Full access? Get Clinical Tree
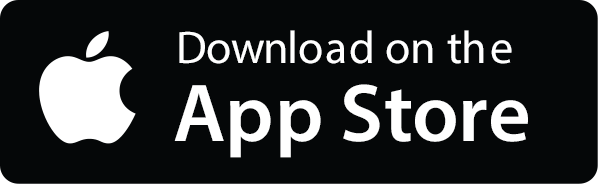
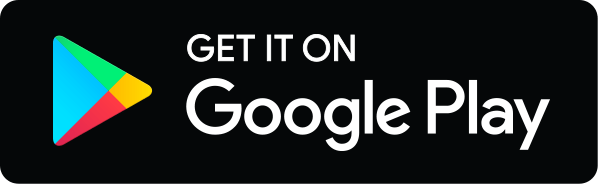