Introduction
Sonography is often the first-line imaging technique for the evaluation of vascular disease, with the various aspects of this modality, including gray-scale, color or power Doppler, contributing to the success of the examination. The multiparametric nature of sonography has been expanded with the introduction of ultrasound contrast agents (UCAs). Non–contrast-enhanced ultrasound imaging has inherent limitations in the assessment of vascular diseases, such as the inadequate detection of blood flow signals in markedly stenotic lesions and in small or deep-lying blood vessels. The lack of sensitivity to slow blood flow near vessel walls becomes more evident in the assessment of plaque ulceration. Moreover, technical artifacts that include blooming, aliasing and Doppler angle dependency can hinder the ultrasound examination. All of these may result in spurious Doppler signals in the vascular lumen. These limitations may decrease accuracy particularly for distinguishing severe arterial stenosis from occlusion. Failure of the sonographic technique can lead to the use of other imaging modalities (e.g., computed tomography angiography [CTA] or magnetic resonance angiography [MRA]), thereby increasing the time needed for final diagnosis, patient cost, and anxiety.
Contrast-enhanced ultrasound (CEUS) is characterized by improved blood flow visualization in superficial and deep blood vessels with the added benefit of increased spatial and temporal resolution. These advantages in blood flow visualization apply to all types of scenarios, including visualizing slow flow in preocclusive stenosis. Although the use of UCA is licensed and well established for vascular applications in Europe, it is only recently that a UCA has been approved for noncardiac applications in the United States, albeit solely for the use of characterizing focal liver lesions.
Although UCAs were initially developed as a Doppler rescue tool during conventional color Doppler examinations, contrast-specific sonographic techniques now offer improved and prolonged visualization of the UCA within the vascular space, creating sonographic angiographic images. CEUS studies rely on low mechanical index (MI) techniques, differ from conventional color Doppler images, and are characterized by the ability to image only the intravascular UCA by suppressing the normal tissue signals.
Technical Aspects of Contrast-Enhanced Ultrasound
Types of ultrasound contrast agents
The first UCA described and widely used for sonographic evaluation of the cardiac chambers was nothing more than manually agitated saline administered into a peripheral vein to increase blood reflectivity on gray-scale and color Doppler images. Nonetheless, this primordial UCA could only be used to evaluate the right cardiac chambers or to detect right-to-left intracardiac shunts because agitated saline will not cross the pulmonary capillary circulation.
Advancements have since been made in the field of UCAs with the introduction of a series of commercially available agents. UCAs are characterized as blood-pool agents with the inherent property of strictly remaining within the vascular space. These agents consist of microbubbles, whose small size (approximately 10 µm) prevents their passage through the vascular endothelium. UCAs are capable of traversing the pulmonary capillary circulation, allowing prolonged enhancement of both arteries and veins. The first generation of UCAs included Levovist (Schering AG, Berlin, Germany), an encapsulated air bubble stabilized with palmitic acid, that was widely used and investigated but is no longer manufactured. Second-generation UCAs offer improved enhancement and longer circulation times, taking advantage of low-solubility gases that replaced air, and a more robust shell. Optison (GE Healthcare, Milwaukee WI) and SonoVue (Bracco Imaging SpA, Milan, Italy) are two examples of second generation UCAs. The latter is the most frequently used UCA in Europe, registered for vascular, cardiac, breast, and liver applications. SonoVue consists of sulfur hexafluoride gas encapsulated within a phospholipid shell that increases in vivo stability. It is approved in the United States for noncardiac applications under the commercial name Lumason (Bracco Imaging SpA, Milan, Italy). When administered intravenously, the Lumason microbubbles remain inside the blood vessels until the microbubbles rupture; the contained gas is then exhaled, and the liver metabolizes the remnants of the phospholipid shell. Because there is no renal excretion of the UCAs or their breakdown products, they can be safely used in patients with impaired renal function. All currently marketed and registered UCAs in the United States and Europe are listed in Table 35.1 .
Agent, Manufacturer | Countries Licensed | Approved Indications/Properties |
---|---|---|
Optison (GE Health-care, Princeton, NJ) | USA, EU | LVO/EBD |
Definity (Lantheus Medical Imaging, N Billerica, MA) | Canada, Mexico, Israel, New Zealand, India, Australia, USA, EU, South Korea, Singapore, United Arab Emirates | LVO/EBD, liver, kidney LVO/EBD |
SonoVue/Lumason (Bracco Imaging S.p.A., Milan, Italy) | EU, Norway, Switzerland, China, Singapore, Hong Kong, South Korea, Iceland, India, Canada, USA | LVO/EBD, breast, liver, portal vein, extracranial carotid, peripheral arteries (macrovascular/microvascular) LVO/EBD, diagnostic assessment of vessels LVO/EBD, liver (also for pediatric use) |
Sonazoid (Daiichi Pharmaceutical Co., Ltd., Tokyo, Japan; manufactured and distributed in partnership with GE Healthcare) | Japan | Focal liver lesions |
Ultrasound contrast agent administration
A CEUS study is usually performed after an unenhanced ultrasound study. UCAs are administered via a peripheral vein. Various types of catheters or needles ranging in size from 18 to 21 gauge can be used without affecting the quality of enhancement or concentration of microbubbles. The dose required depends on the application, machine sensitivity, and transducer frequency. With SonoVue or Lumason, the recommended dose for a liver examination is 2.4 mL, but the dose may vary considerably between 1.0 and 2.4 mL depending on the type of study. The UCA is usually administered as a bolus followed by a normal saline flush of 5 to 10 mL. Vascular lumen enhancement commences a few seconds after UCA administration and lasts for several minutes. If required, a second dose of UCA may be safely administered as soon as vessel lumen enhancement decreases, or when additional imaging is needed, for example of the contralateral side during an examination of the carotid arteries. As an alternative to a bolus injection, UCAs can also be administered via slow intravenous infusion, allowing for prolonged vascular enhancement in demanding or time-consuming examinations.
Basic principles of contrast-enhanced ultrasound technique
UCAs can be visualized with conventional gray-scale and color or power Doppler techniques, termed Doppler rescue because the blood’s echogenicity is increased on gray-scale imaging and the Doppler signals are significantly increased on color Doppler and duplex imaging. However, this technique results in a number of artifacts, including blooming and saturation that limit image quality. This technique is not capable of evaluating the microvasculature and tissue perfusion in solid organs, but it may be used for the evaluation of the macrovasculature, for example to distinguish between carotid occlusion and preocclusive stenosis.
A significant advancement in CEUS imaging technology was the introduction of harmonic imaging. The UCA microbubbles tend to oscillate in a nonlinear fashion when exposed to the acoustic energy of an ultrasound beam; microbubbles expand more than they contract. This asymmetric fluctuation in microbubble size leads to reflected echoes containing both the fundamental (emitted) frequency as well as harmonic and subharmonic frequencies (higher and lower frequencies). Harmonic ultrasound contrast imaging takes advantage of this phenomenon by configuring the transducer to receive and to visualize only echoes within a specified frequency range (i.e., typically double the frequency of the emitted frequency [first harmonic]). A convenient and fortunate coincidence is that the natural frequency of microbubble oscillation for commercially available contrast agents lies at approximately 3 MHz, the frequency typically used for abdominal examinations. This explains the excellent signal provided by UCAs in spite of the small doses administered. As a result, echoes reflected from microbubbles are visualized with an improved signal-to-noise ratio when compared with conventional gray-scale ultrasound imaging. Nevertheless, image quality is lowered by the simultaneous generation of harmonic echoes from static tissues. This harmonic response of static tissues is known to be proportional to the MI, one reason why low-MI should be used for CEUS examinations.
Pulse-inversion harmonic imaging allows visualization of echoes reflected from UCAs while suppressing those arising from static tissues. To understand how this technique works, it is important to appreciate that static tissues are mainly linear reflectors, meaning that they emit echoes with the same frequency as that transmitted by the transducer. Static tissues may also respond nonlinearly when high-MI waves are used, but this effect is minimized because the current devices use low-MI pulses. UCAs respond to the ultrasound wave in a nonlinear fashion because of asymmetric microbubble oscillation and will emit echoes in the harmonic frequencies. In pulse-inversion imaging, the transducer emits a sequence of two pulses identical in terms of frequency and amplitude, but the second pulse is 180 degrees out of phase compared with the first. The returning echoes from these pulses cancel one another when they encounter soft tissues that act as linear reflectors. However, when these pulses encounter nonlinear reflectors such as UCAs, the reflected harmonic components are combined to produce signals of higher intensity. As a result, this technique has the potential to visualize returning signals from microbubbles while erasing static tissue signals in real-time. This technique can be used to produce both two-dimensional (2D) and three-dimensional (3D) images.
Another reason low-MI real-time sonography is particularly important is that high-MI ultrasound waves rupture microbubbles, resulting in a decrease in microbubble enhancement. This adverse effect is avoided with low-MI imaging, leading to optimized and prolonged visualization of UCAs thereby increasing the clinical utility of CEUS. However, the destruction of microbubbles by high-MI pulses can be used for diagnostic purposes by assessing the perfusion pattern within a targeted organ or soft tissue. A brief and short-lived high-MI pulse can be applied to purposely cause the destruction of microbubbles lying within the imaging field. Reversion to low-MI imaging can then image reperfusion as the microbubbles return into the imaging field. This technique can be useful in the evaluation of tumor neovascularity or the vascular pattern of focal liver lesions. Temporal maximum intensity projection (MIP) imaging is another CEUS technique making use of high-MI pulses to cause microbubble destruction in order to monitor the subsequent replenishment of intact microbubbles within the targeted soft tissue and to create cumulative images, similar to the spatial MIP images generated for CTA and MRA. In temporal MIPs, the ultrasound device acts as an open-shutter camera recording, aggregating the bright echoes originating from intact microbubbles as they enter the imaging field that was left devoid of microbubbles after their destruction by a high-MI pulse. This process is valuable in evaluating the architecture of the microvasculature of liver tumors or for characterizing vascular lesions such as extracranial carotid artery stenosis. These contrast-specific techniques have been extensively studied in clinical trials and are the dominant methods for CEUS examinations in every day clinical practice.
Another CEUS scanning technique used to limit microbubble destruction is intermittent imaging. In this imaging mode, the ultrasound transducer is configured to emit and to receive an ultrasound pulse in a fixed and operator-defined time interval or in a predefined part of the electrocardiogram when imaging the heart. This method of imaging allows time for microbubbles to enter the scanning field and increases reflectivity, but sacrifices real-time information. Depending on the manufacturer, ultrasound devices have the potential for combining real-time low-MI imaging with intermittent high-MI imaging in a dual-image display pattern.
Quantification of contrast enhancement
CEUS examinations are qualitatively evaluated so that a subjective impression can be rendered. An advantage of CEUS when compared with conventional sonography is its potential to provide quantification of the contrast enhancement. The main advantage of this type of analysis is deriving quantitative variables that describe the pharmacokinetic curve of UCA enhancement, allowing reproducibility and increased interobserver and intraobserver agreement. These variables include time-to-peak enhancement, duration of enhancement, and wash-in and wash-out times and are computed from the time-intensity curves sampled within a region-of-interest. Quantification of CEUS examinations can be performed at the time of examination with software incorporated in the ultrasound device or with software for offline analysis. VueBox (Bracco Suisse SA, Geneva, Switzerland) is a widely used software application for quantification of CEUS examinations. Quantification analysis of CEUS examinations can be simplified by analyzing only a selection of frames and not the entire examination video loop. Examples where the quantification of CEUS examination has been valuable include tumor response to biologic therapy, intraplaque neovascularization in carotid plaques, and bowel wall vascularity in patients suffering from Crohn disease.
Artifacts
Like any ultrasound technique, CEUS has inherent artifacts. Use of UCAs generates additional technical artifacts that need to be appreciated by the sonographer. For example, continuous scanning may lead to the degradation of image quality as a result of the destruction of microbubbles. The blooming artifact in the Doppler rescue technique refers to the excessive signal enhancement that causes color Doppler signals to appear outside the vessel lumen and lead to the erroneous perception of blood flow. This artifact is similar to that seen with conventional color Doppler examinations and can be resolved by reducing the color gain setting or setting a higher pulse repetition frequency.
Spectral Doppler artifacts related to the administration of microbubbles include spectral broadening and the presence of high-intensity spikes scattered throughout the waveform caused by microbubble disruption (bubble noise). Spectral waveform Doppler analysis should be performed prior to the administration of microbubbles because UCAs may increase the measured peak velocity.
The pseudoenhancement artifact refers to the presence of echo signals situated in the vascular wall or within atherosclerotic plaques lying distally to the transducer and is created by the nonlinear propagation and distortion of ultrasound pulses through the cloud of microbubbles in the vascular lumen. This artifact mimics the enhancement demonstrated by intraplaque neovascularization. This issue is being addressed by the development of new ultrasound pulse sequences. The term incomplete suppression artifact refers to the visualization of hyperechoic areas lying outside the vascular lumen on images produced by contrast-specific techniques. These signals are caused by the reflection of ultrasound by heavily reflective objects and can be differentiated from true enhancement because such echoes appear constantly static, whereas echoes originating from microbubbles are moving.
Safety of Ultrasound Contrast Agents
UCAs are considered safe, with a low reported rate of adverse events. The frequency of severe hypersensitivity or anaphylactoid reactions is lower than that seen for iodinated contrast agents and comparable to that of magnetic resonance contrast agents. Potentially fatal allergic reactions have been reported in less than 0.002% of examinations. Regardless of this excellent safety profile, resuscitation facilities must be available where CEUS examinations are performed. A relative contraindication for the administration of an UCA is unstable ischemic heart disease in the week prior to the CEUS examination. There is insufficient evidence to establish the association between the administration of UCAs and death in patients with severely compromised cardiac function.
UCAs are not nephrotoxic (as they are not excreted via the urinary tract) and can be administered in patients with impaired renal function with no requirement for any preceding biochemical profile and are not contraindicated in thyroid disease. As data are accumulating, UCAs should be used with caution during pregnancy and breastfeeding, although these relative contraindications may vary between UCAs and different countries.
- •
UCAs can be imaged without the use of harmonic imaging.
- •
Harmonic imaging increases the versatility of the CEUS examination.
- •
The resonant frequency of microbubbles is close to 3 MHz.
- •
The harmonic signals generated from microbubbles are a result of their nonlinear response to the ultrasound beam: they expand more than they contract.
- •
Specific artifacts linked to the use of rescue Doppler are blooming, bubble noise, and apparent increase in the Doppler spectral envelope and measured velocity.
- •
In addition to harmonic imaging, inversion recovery pulses can be used to evaluate tissue perfusion.
- •
Dynamic perfusion curves and quantitative measurements of blood flow and perfusion can be generated either during the examination or offline.
- •
UCAs such as Lumason are not contraindicated in patients with decreased renal function.
- •
The rate of allergic reactions to UCAs is similar to that seen with gadolinium contrast agents.
Clinical Applications of Ultrasound Contrast Agents
Cerebrovascular applications
CEUS is used to evaluate the extracranial and intracranial vasculature in patients with carotid atherosclerotic disease and stroke. The superficial location and accessibility of the extracranial carotid arteries are ideal for ultrasound imaging with and without contrast agents. CEUS has the potential to generate real-time angiographic ultrasound images. The administration of UCAs also significantly improves the quality and reliability of transcranial Doppler (TCD) sonography.
Extracranial vessels
The benefits of CEUS include superior blood flow visualization and vascular lumen delineation even in very stenotic arteries, small-caliber vessels, and vessels with low blood flow velocities. CEUS is superior to unenhanced ultrasound when differentiating between carotid occlusion and preocclusive stenosis ( Figs. 35.1–35.3 ). CEUS results are consistent with angiography and surgical findings in detecting carotid occlusion or pseudo-occlusion. Other studies have demonstrated that CEUS is superior to time-of-flight MRA and equivalent in diagnostic accuracy to contrast-enhanced MRA and CTA for classification of a carotid artery as occluded or highly stenotic. UCAs significantly improve the diagnostic accuracy of ultrasound in cases of suspected carotid occlusion, readily and confidently excluding the presence of flow signals and averting the need for additional imaging. This benefit is greatest in patients with renal failure where CTA and contrast-enhanced MRA are contraindicated.
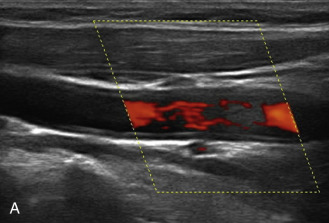
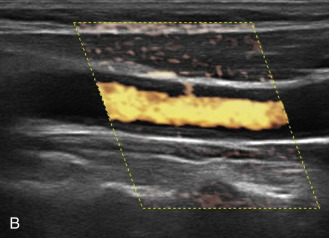
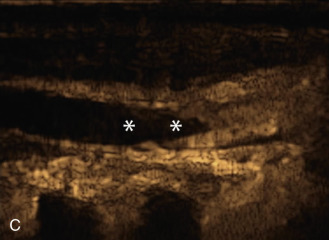
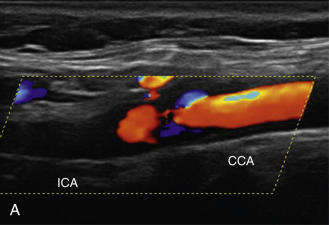
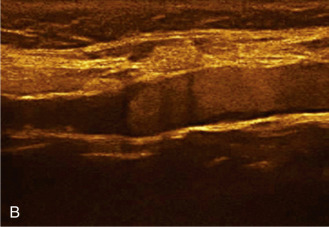
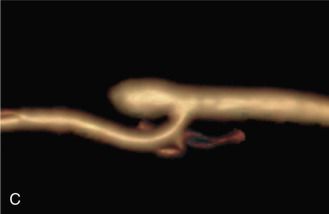
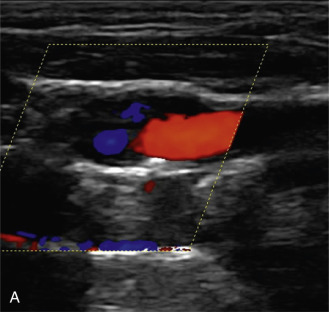
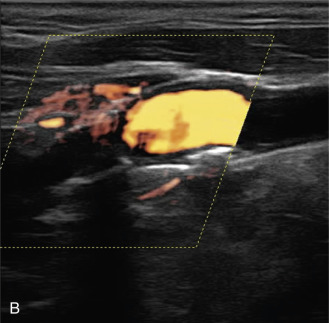
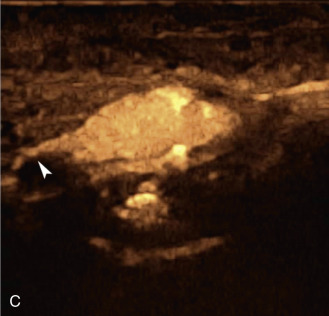
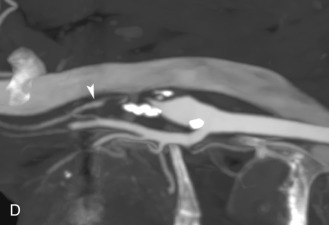
The use of a first-generation UCA improved assessment of the degree of stenosis based on the North American Symptomatic Carotid Endarterectomy Trial (NASCET) criteria, showing strong correlation with arteriography. It is likely that the newer contrast agents will perform similarly if not better than the earlier contrast agents. CEUS has shown potential to provide detailed characterization of plaque surface irregularities such as ulcerations or dissections that are not seen on unenhanced ultrasound images. Carotid plaque ulceration is associated with an increased risk of incident stroke and should be accurately diagnosed. The diagnostic accuracy of CEUS for the detection of ulcerated carotid plaques has been found superior to conventional unenhanced ultrasound when correlated with CTA and histology ( Figs. 35.4 and 35.5 ). CEUS can detect superficial ulcerations as small as 1 mm. CEUS has been successfully used for the detection of ulcerated carotid plaques in asymptomatic patients with diabetes mellitus. In asymptomatic patients with cardiovascular risk factors, the administration of a UCA enables the identification of subclinical atherosclerosis by demonstrating hypoechoic plaques not seen on conventional color Doppler images. Three-dimensional techniques coupled with CEUS demonstrate a 93% correlation with intraoperative findings in internal carotid artery stenosis. With improved delineation of the carotid artery lumen interface, CEUS can also be used to measure carotid intima-media thickness (IMT) with increased reliability when compared with unenhanced ultrasound.
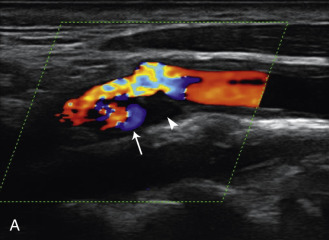
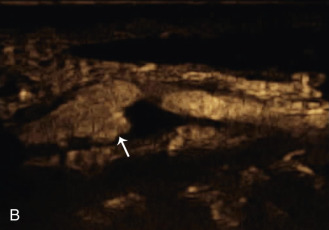
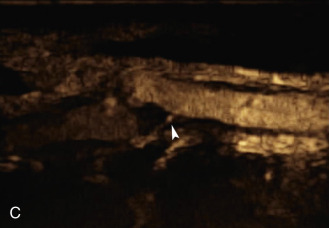
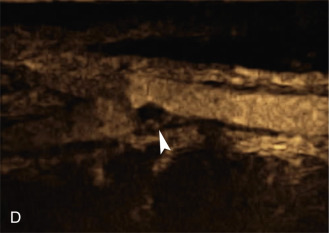
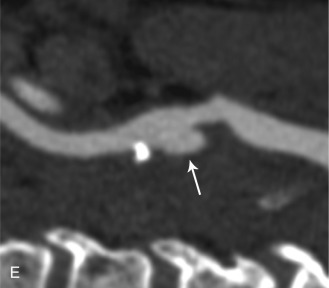
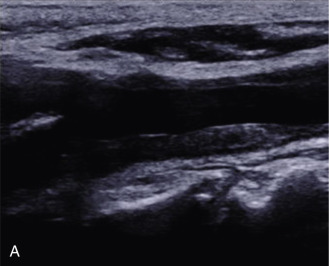
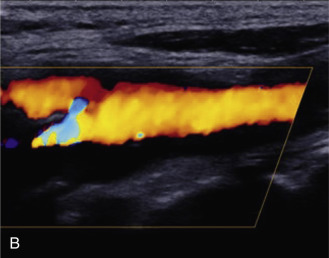
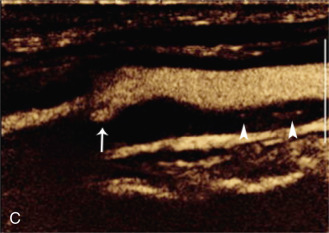
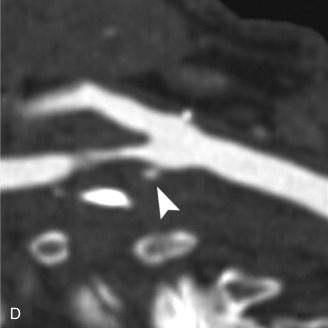
The evaluation of intraplaque neovascularization with CEUS should facilitate the detection of vulnerable plaques. Because microbubbles remain strictly within the intravascular space, moving microbubbles seen in an atherosclerotic plaque represent intraplaque neovascularity (see Figs. 35.4 and 35.5 ). This has been confirmed by studies showing a positive correlation between plaque enhancement on CEUS and histologically proven neovascularization in endarterectomy specimens. Plaque enhancement on CEUS has been significantly correlated with the occurrence of neurologic symptoms, ipsilateral embolic lesions on brain computed tomography (CT), ruptured plaques, and microembolic signals on transcranial Doppler sonography, highlighting the value of CEUS for evaluating intraplaque neovascularity.
Subjective evaluation of plaque enhancement on CEUS can be performed using a three-grade classification system: mild, moderate, and severe. Mild enhancement describes the presence of moving microbubbles only within the outer part of a plaque and near the adventitia. Moderate enhancement refers to microbubbles seen both at the plaque shoulder and within the plaque surface but not at the apex of the plaque. Finally, the term severe enhancement is used for plaques showing moving microbubbles scattered throughout the plaque surface, including the apex. Furthermore, CEUS plaque enhancement measured with a quantification system is consistent with histologic findings from endarterectomy specimens.
Quantification of carotid plaque enhancement is based on the analysis of time-intensity curves that track the level of enhancement in a region of interest with time ( Fig. 35.6 ). Variables derived from time-intensity curves show excellent interobserver and intraobserver agreement. The use of quantitative analysis confirmed the greater enhancement seen with symptomatic plaques when compared with asymptomatic plaques. Owen and colleagues have examined the value of delayed phase CEUS (6 minutes after the administration of microbubbles) in identifying intraplaque inflammation, concluding that delayed enhancement is greater in symptomatic plaques. CEUS has been evaluated in the experimental setting for monitoring and follow-up of plaque response to treatment, documenting the inhibition of adventitial vasa vasorum formation with the use of atorvastatin. Remaining issues to be addressed before incorporation of the detection of intraplaque neovascularization into everyday clinical practice include the selection of an optimal quantification method and the need for technique standardization.
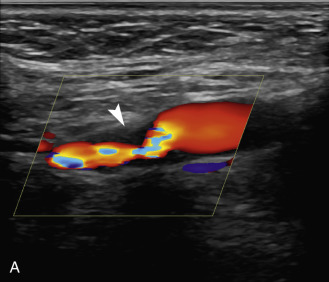
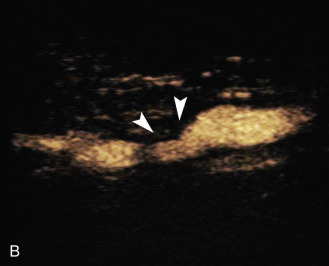
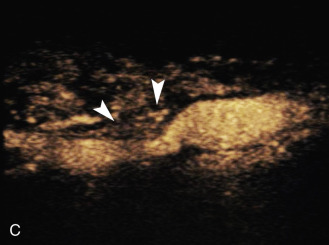
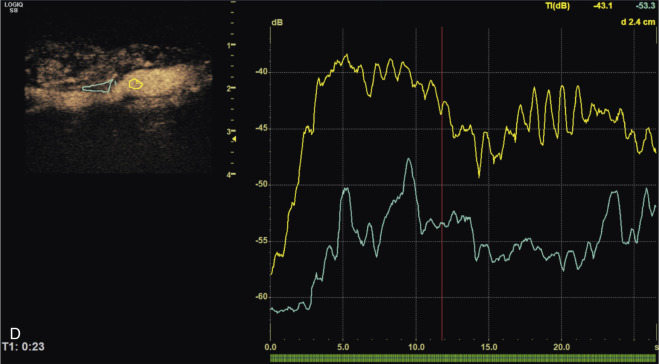
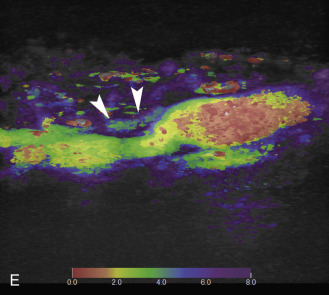
CEUS is a valuable adjunct to the assessment of suspected restenosis after angioplasty or endarterectomy. UCAs have been used to increase the diagnostic accuracy of ultrasound in cases of suspected carotid dissection by taking advantage of the improved spatial and temporal resolution provided by CEUS. The administration of a UCA helps identify intimal flaps and helps distinguish the true from the false lumen because UAC arrival in the true lumen precedes that of the false lumen, similar to findings on CTA and MRA ( Fig. 35.7 ). CEUS is more sensitive than unenhanced ultrasound in visualizing slow flow within the false lumen, a flow pattern that indicates a higher likelihood of thrombus formation. As with other applications of CEUS, UCAs can be administered to patients who cannot undergo CTA or contrast-enhanced MRA because of impaired renal function. Other reported applications of CEUS of the carotid arteries include the evaluation of carotid body tumor perfusion prior to and after embolization, the visualization of slow blood flow within true or pseudoaneurysms, the detection of carotid-jugular arteriovenous fistulas after jugular vein catheterization, and the assessment of carotid wall vascularization in patients with Takayasu or giant cell arteritis before and after treatment ( Fig. 35.8 ).
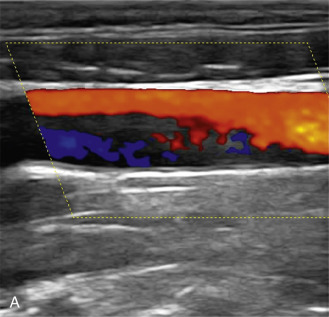
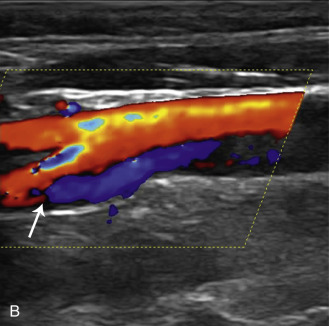
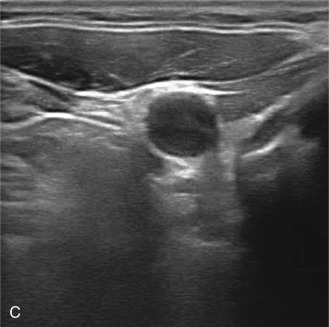
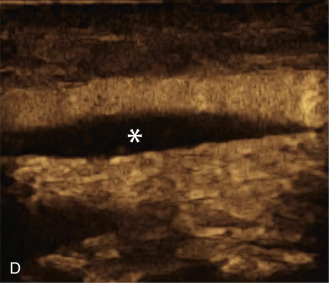
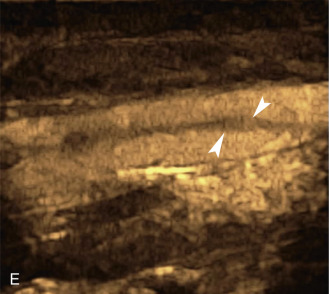
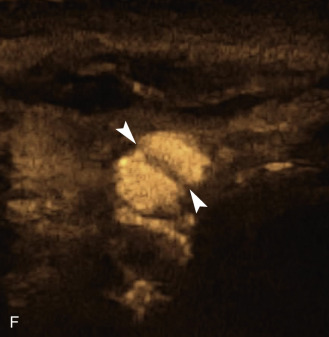
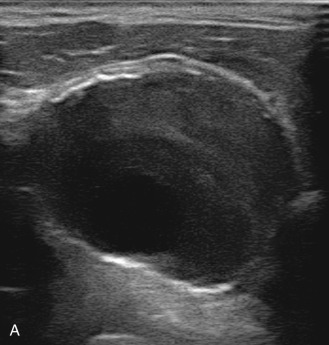
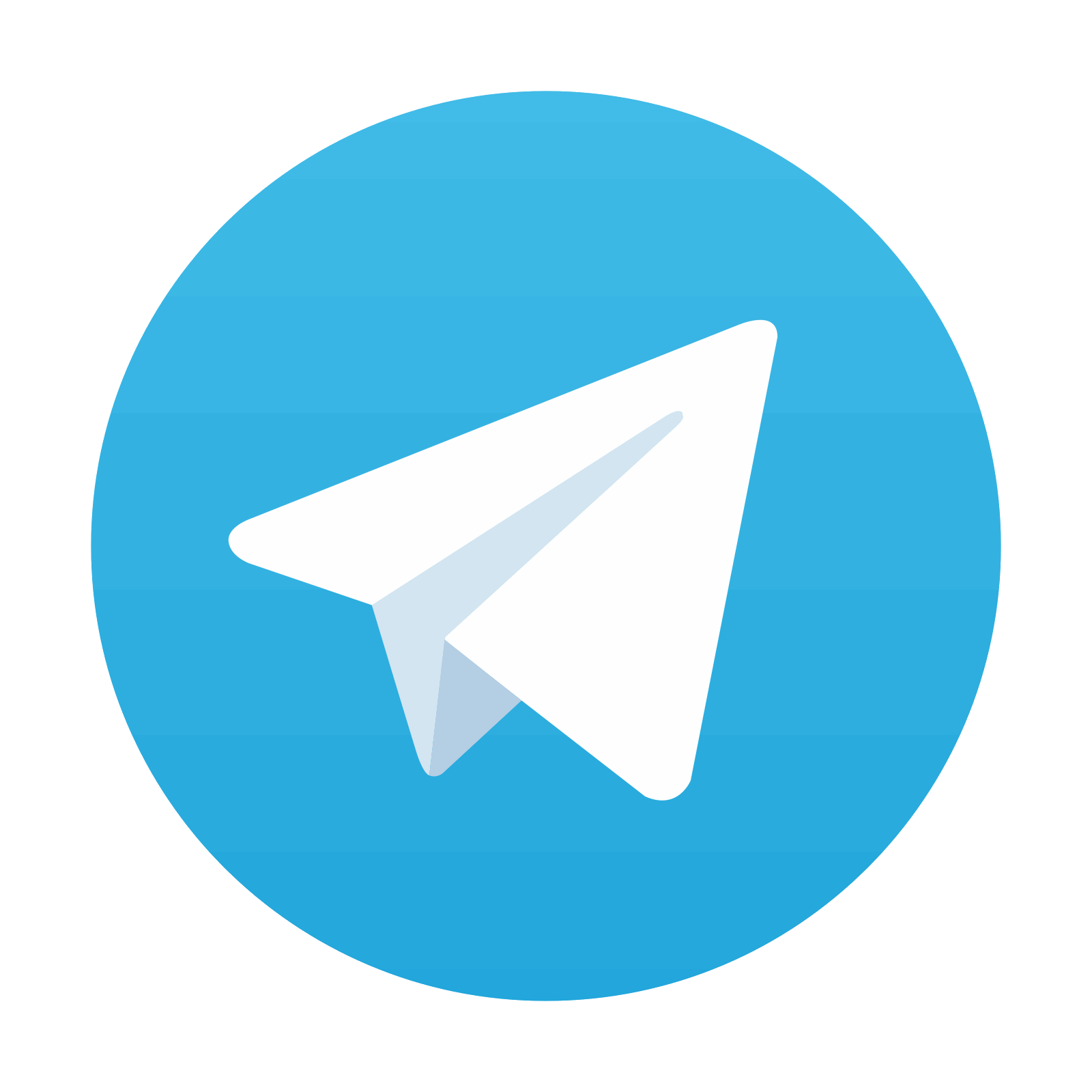
Stay updated, free articles. Join our Telegram channel

Full access? Get Clinical Tree
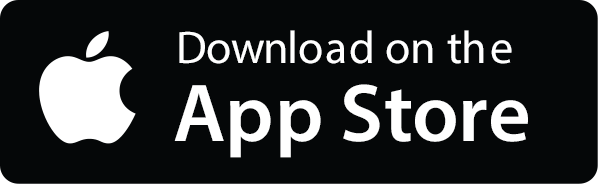
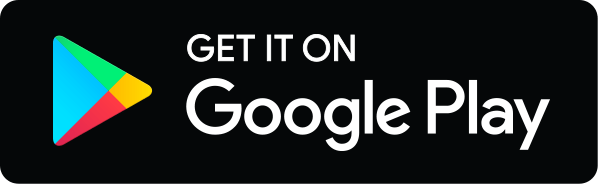
