Precision medicine integrates molecular pathobiology, genetic make-up, and clinical manifestations of disease in order to classify patients into subgroups for the purposes of predicting treatment response and suggesting outcome. By identifying those patients who are most likely to benefit from a given therapy, interventions can be tailored to avoid the expense and toxicity of futile treatment. Ultimately, the goal is to offer the right treatment, to the right patient, at the right time. Lung cancer is a heterogeneous disease both functionally and morphologically. Further, over time, clonal proliferations of cells may evolve, becoming resistant to specific therapies. PET is a sensitive imaging technique with an important role in the precision medicine algorithm of lung cancer patients. It provides anatomo-functional insight during diagnosis, staging, and restaging of the disease. It is a prognostic biomarker in lung cancer patients that characterizes tumoral heterogeneity, helps predict early response to therapy, and may direct the selection of appropriate treatment.
Key points
- •
PET is helpful to stage patients with lung cancer.
- •
PET is a prognostic biomarker that is key in the initial treatment strategy of lung cancer patients by noninvasively assessing disease pathobiology so that appropriate therapy can be instituted.
- •
PET is helpful for the subsequent treatment strategy in lung cancer patients, including monitoring therapy response, detecting recurrence, and predicting outcomes.
Lung cancer and precision medicine
Lung cancer is the second most commonly diagnosed malignancy in both men and women, accounting for approximately 235,760 new cases and 131,880 deaths in 2021 according to the American Cancer Society. Although localized disease may be curable, metastatic lung cancer remains the leading cause of cancer-related death today, accounting for almost 25% of all cancer-related deaths and surpassing deaths from breast, prostate, and colorectal cancer combined.
Lung cancer is a spectrum of disease morphologically, both functionally and genetically. Typically classified into two main subtypes, non-small cell lung cancer (NSCLC) accounts for approximately 85% of cases and includes adenocarcinoma and squamous cell carcinoma (among others), while small cell lung cancer (SCLC) accounts for approximately 15% of cases and is thought to be of neuroendocrine origin. While smoking tobacco is a risk factor for all lung cancer types, adenocarcinoma is more common in patients who have never smoked. Therapy may include surgery, radiation, chemotherapy, and immunotherapy, as well as an array of targeted drug therapies such as epidermal growth factor receptor (EGFR) inhibitors (eg, Erlotinib) and drugs targeting genetic mutations (eg, ALK, ROS1), among others. Localized NSCLC is often managed by surgery and adjuvant therapy; SCLC is rarely localized and tends to be treated nonsurgically.
Coupling the genetic underpinnings of malignancy with the use of molecular imaging to assess disease extent, its biologic behavior and metabolic response to therapy, contributes essential information to the personalized management of the lung cancer patient. , More specifically, it aids in the a priori identification of appropriate treatment for a specific patient at a given point in time, in order to maximize benefit while limiting toxicity. Positron emission tomography (PET) plays an important role in lung cancer patients both for initial staging and for planning the subsequent treatment strategy ( Figs. 1–3 ). PET contributes to precision medicine by characterizing tumor heterogeneity, by directing appropriate therapy at a metabolic-molecular level, and by providing predictive and prognostic insight into the therapy response. Further, PET can identify disease sites that are becoming resistant to treatment such that changes can be made at an earlier time point. The primary strength of PET has remained unchanged over the years, namely its ability to noninvasively evaluate in vivo tumor extent and heterogeneity over both time and space. Current scanners incorporate PET with computed tomography (CT) or magnetic resonance imaging (MRI). While PET/CT is more widely available than PET/MR, our experience with PET/MR will likely grow in the coming years. Further, technological advances are increasing our ability to image faster, with improved resolution and reduced patient radiation dose exposure. Ultimately, careful review of all images and attention to artifacts (ie, respiratory motion or metal artifact) remains key. In our experience, nonattenuation corrected images are particularly helpful to detect small pulmonary nodules. Also, respiratory gating may be helpful to decrease artifacts, although this is rarely done in routine clinical practice. Regardless of whether a PET/CT or PET/MR scanner is used, PET is important in evaluating lung cancer patients, with a significant impact on precision management including therapy intent (ie, cure vs palliation) and treatment selection (ie, surgery vs radiation).
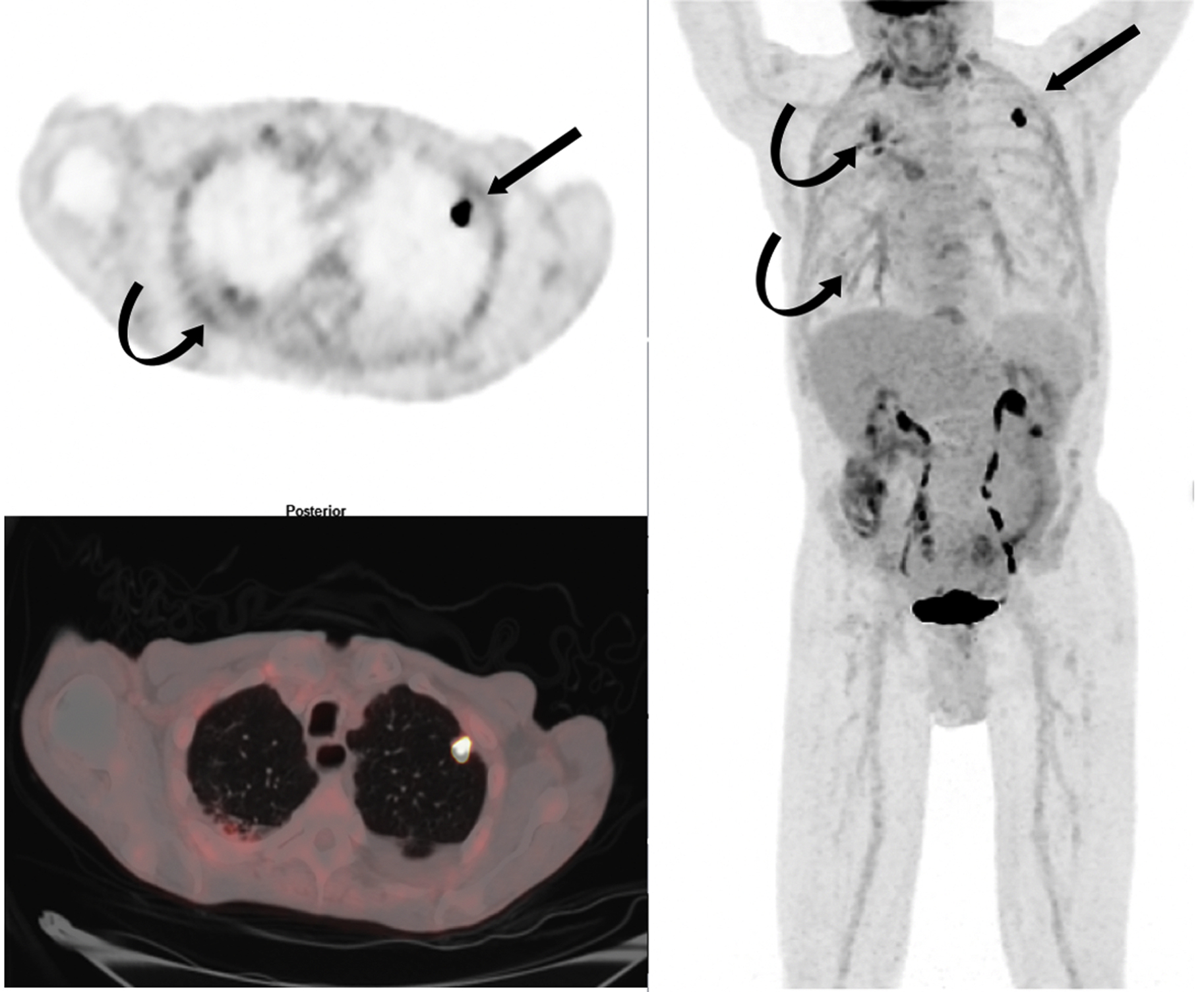
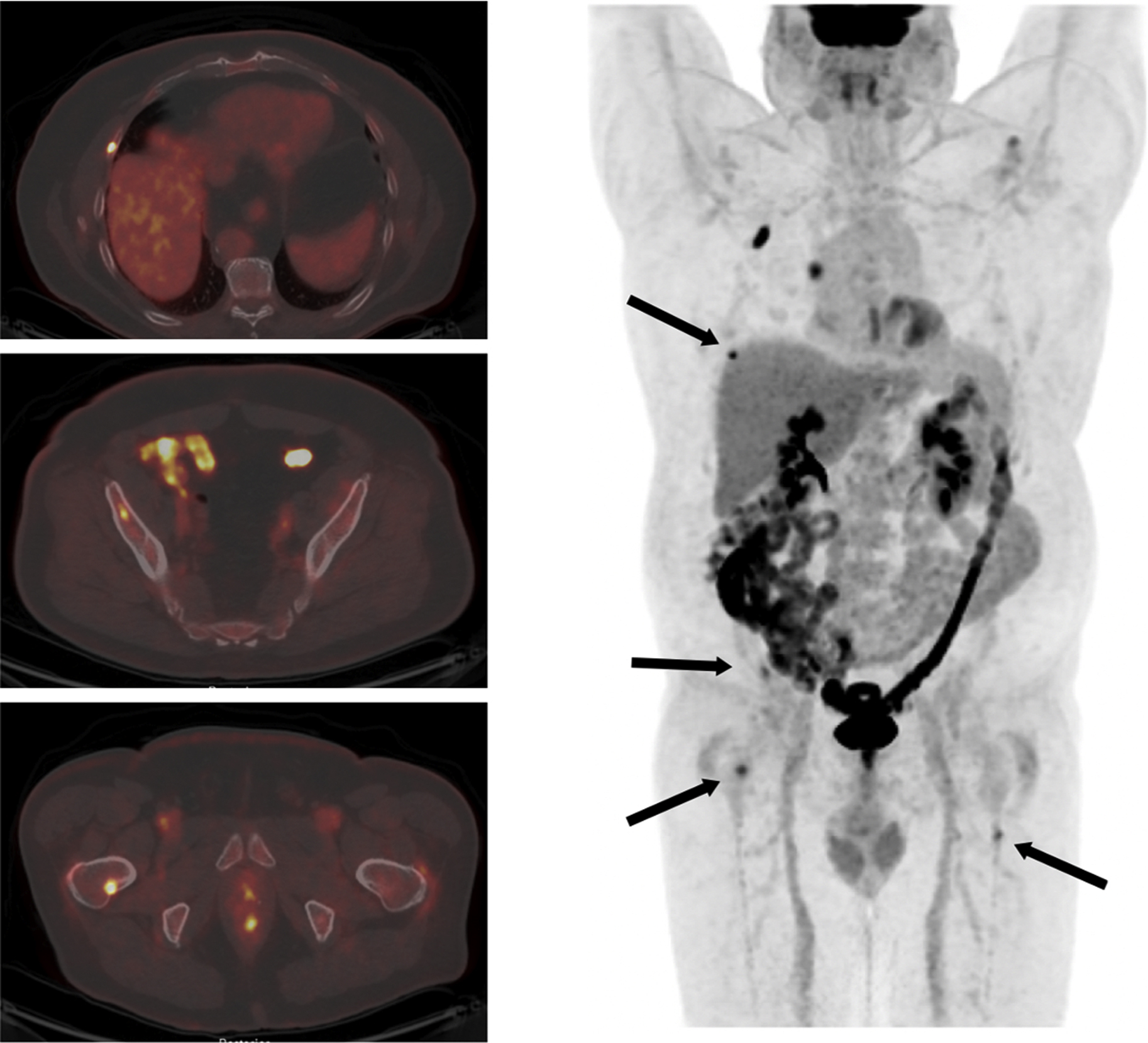
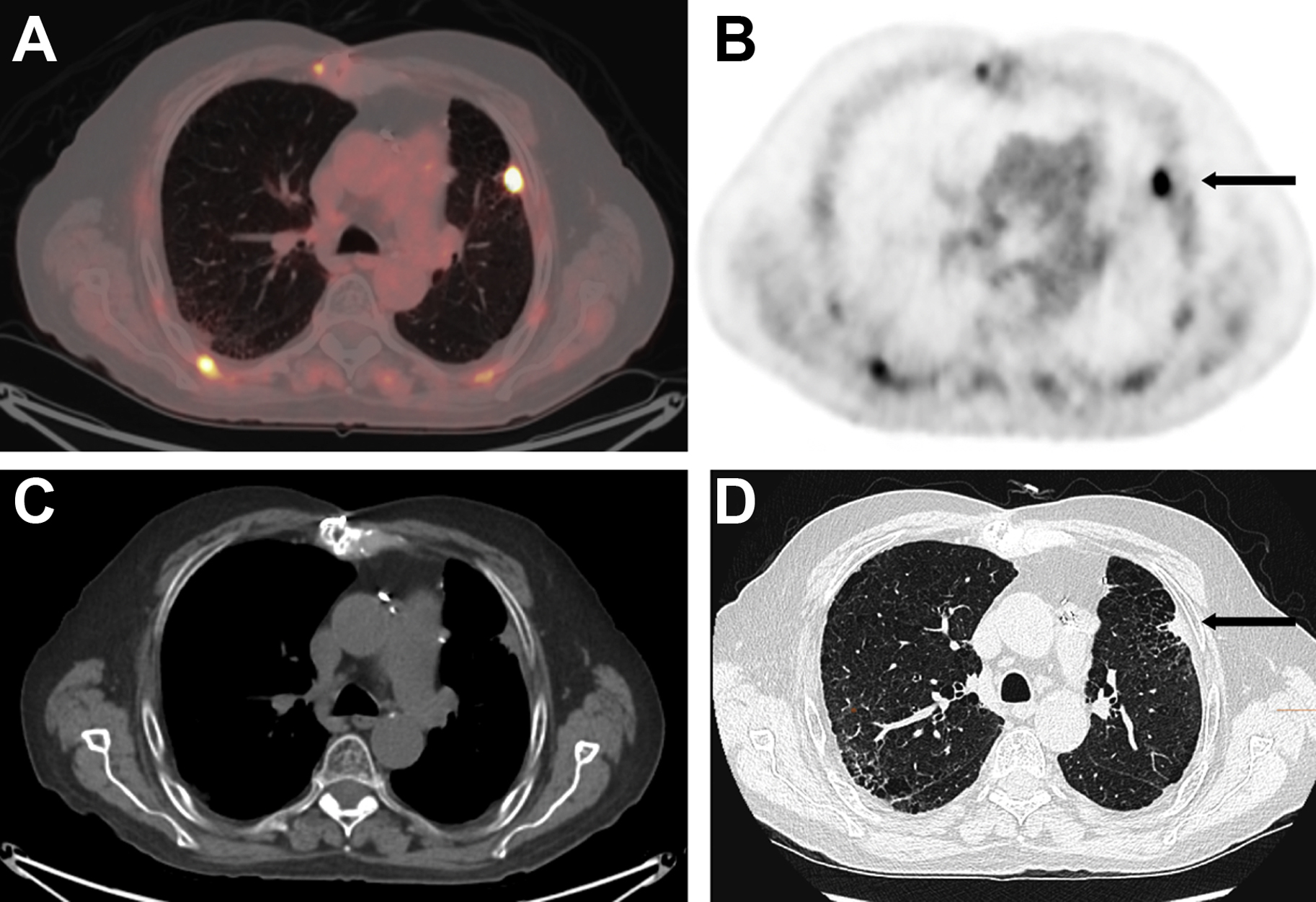
There is ongoing investigation into radiopharmaceuticals that may be helpful for imaging lung cancer patients with PET. Neoplastic cells tend to have higher glucose metabolism than normal tissue and 2-deoxy-2-[ 18 F]fluoro- d -glucose (FDG), a radioactive glucose analogue, is preferentially taken up by neoplastic cells throughout the body and can be imaged with PET. , Also, changes in glucose metabolism may precede anatomic changes and both FDG-PET/CT and FDG-PET/MR are helpful to assess early therapy responses. Since the intensity of FDG uptake depends on factors such as cell density, aggressiveness, and technical parameters, among other things, using standardized parameters is important. Further, FDG uptake may be seen in lung cancer patients related to nonmalignant causes and evaluating imaging in the clinical context is critical ( Figs. 4–6 ). There are several PET radiopharmaceuticals that may be helpful in imaging patient with lung cancer. For example, these include [ 18 F]fluoroazomycin arabinofuranoside (FAZA) and [ 18 F]fluoromisonidazole (1-(2-nitroimidazolyl)-2-hydroxy-3-fluoropropane) (FMISO) for hypoxia imaging, and more recently the quinoline-based ligands targeting cancer-associated fibroblasts (fibroblast-activating protein inhibitors or FAPI), among others. , However, to date, FDG remains the only PET radiopharmaceutical used in routine clinical practice.
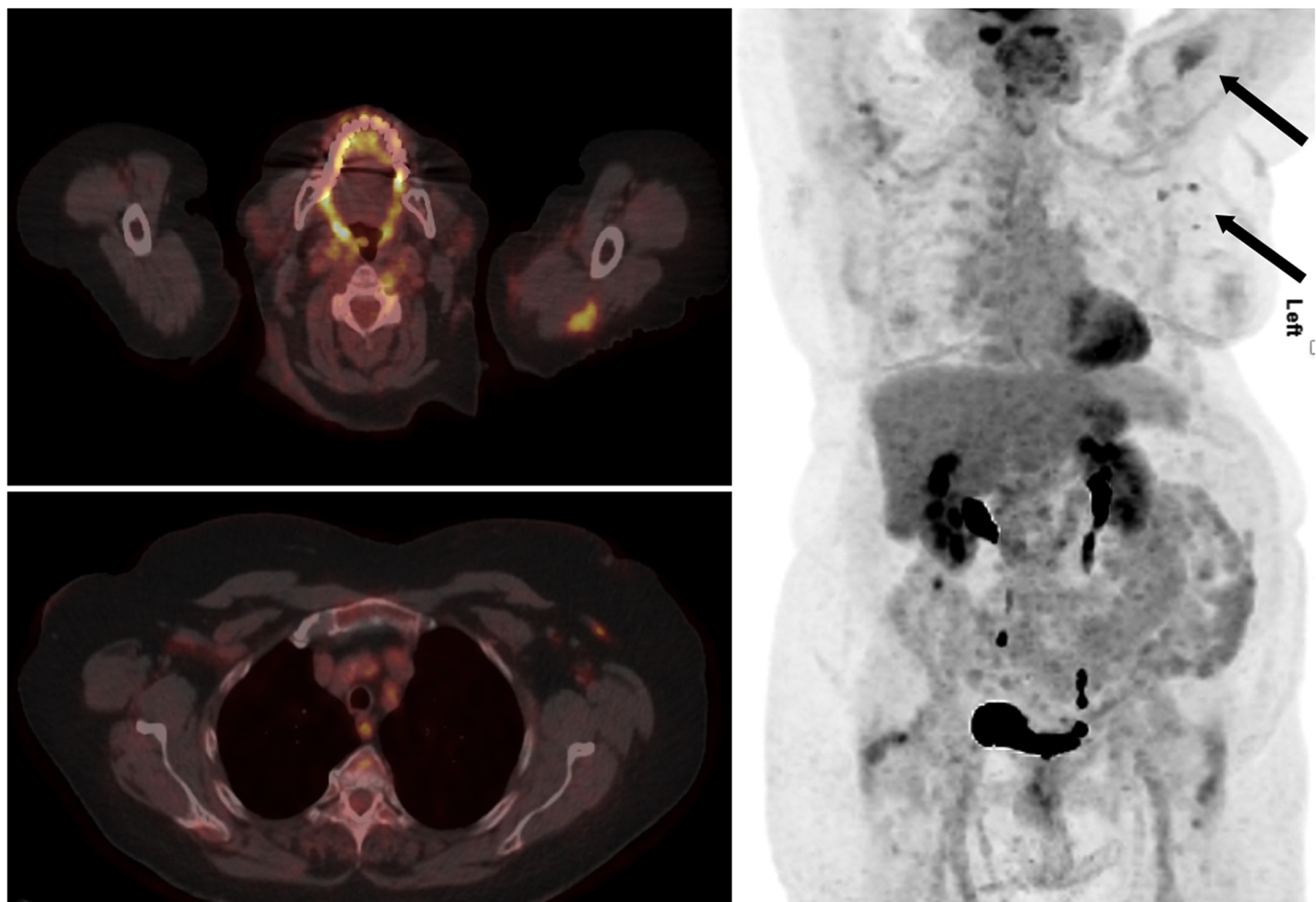
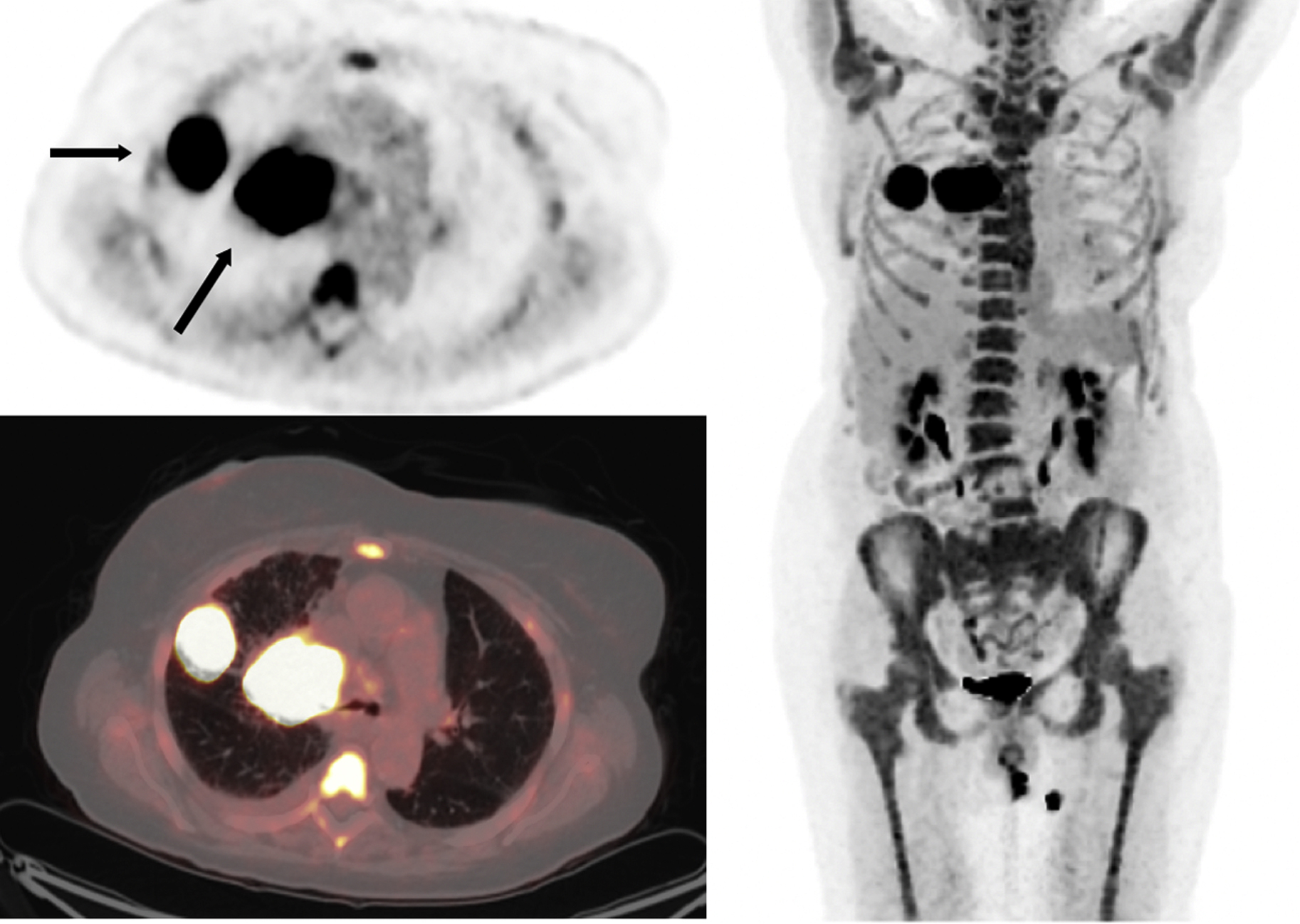
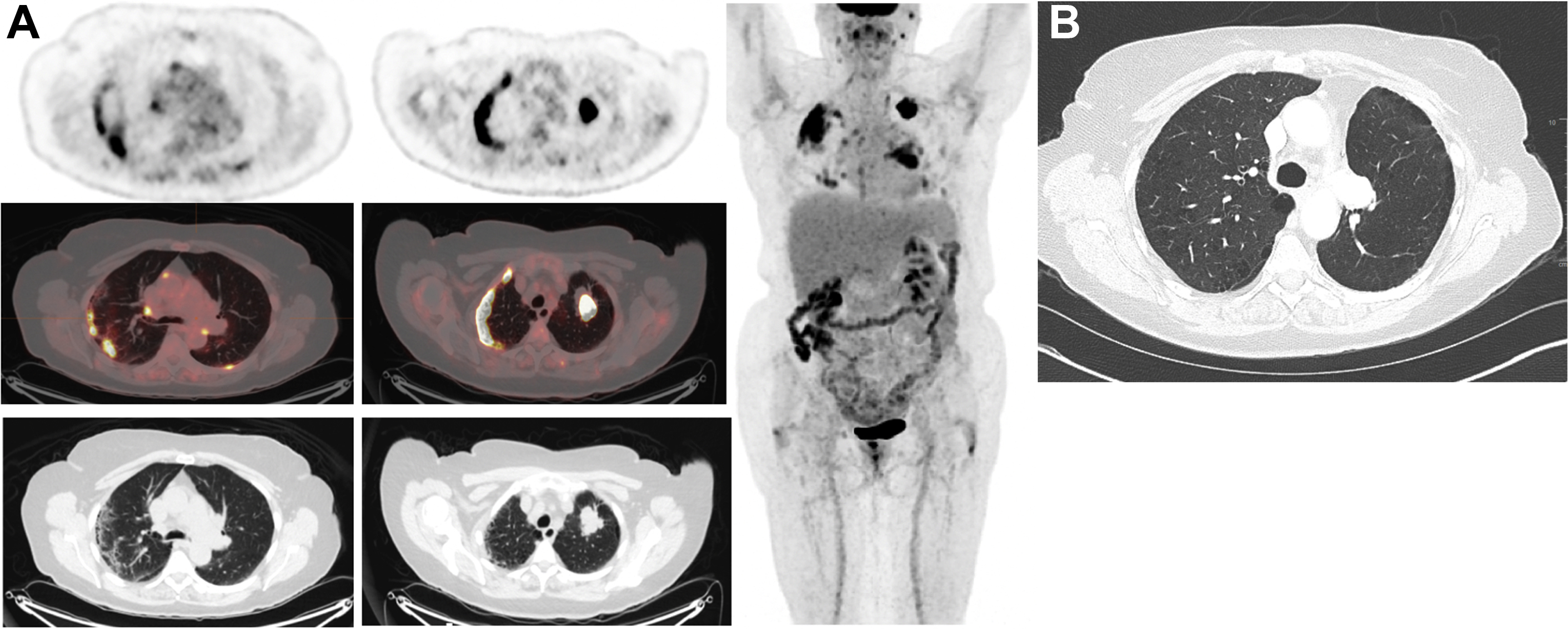
The solitary pulmonary nodule and lung cancer staging
FDG-PET/CT has proven to be helpful to: (1) characterize an indeterminate solitary solid pulmonary nodule that is greater than 8 mm on anatomic imaging; (2) guide biopsy to the site of most aggressive disease; and (3) evaluate disease extent including the primary disease, lymph node spread, and distant metastases. FDG-PET/CT should be interpreted in the clinical context since certain lung cancer subtypes such as carcinoid and rarely well-differentiated adenocarcinoma may have low metabolic activity, while benign processes such as infection or inflammation can be intensely FDG-avid. Further, intense FDG avidity can be associated with interventions such as talc pleurodesis and radiation, among others. In certain settings, such as talc pleurodesis, correlation with CT findings can be particularly helpful. In other cases, delaying imaging after an intervention may be key. For example, the general recommendation is to delay patient imaging for 3 to 4 months after radiation treatment to minimize false-positive FDG uptake due to inflammation, although there is some latitude on this. To wit, Hicks and colleagues showed inflammation after radical radiotherapy did not interfere with response assessment on PET if acquired at a median of 70 days after therapy completion.
A solitary pulmonary nodule (SPN) is defined as less than 3 cm in size, may be solid or subsolid, and is often detected incidentally as a part of routine anatomic imaging. Subsolid pulmonary nodules are categorized as ground glass or mixed solid and ground glass. Typically, FDG-PET/CT is not included in the work-up of incidentally detected subsolid nodules, given the low metabolic activity and risk of false-negative results; in this case, the Fleishner Society guidelines recommend short-interval follow-up CT to establish stability, with continued CT surveillance for up to 5 years as needed. FDG-PET/CT is helpful for the evaluation of solid nodules. FDG-PET/CT has high sensitivity and specificity to characterize an SPN as malignant, and high negative predictive value to exclude malignancy in nodules over 8 mm. For example, in patients with an indeterminate SPN on CT and low probability of lung cancer, low FDG avidity suggests observation may be the most appropriate management. In patients with an indeterminate SPN on CT and intense FDG avidity, histopathology is suggested with surgical resection if this is the only site of malignant disease. The Society of Nuclear Medicine and Molecular Imaging (SNMMI) recommends FDG-PET/CT to: (1) detect a potentially malignant SPN early in the course of disease and potentially enable curative surgery in high-risk patients; (2) to exclude malignancy in low-risk patients with an indeterminate lesion; and (3) to improve healthcare outcomes by avoiding futile surgery in these patients.
PET/CT is helpful for lung cancer staging and impacts management, since treatment strategy is related to staging, as well as tumor biology and genetic mutations. Locoregional and oligometastatic disease may be amenable to surgical resection, adjuvant chemotherapy, and/or targeted radiation. Chemotherapy, molecular targeted treatment, and immunotherapy are typically preferred for patients with distant metastases. The 5-year overall survival for patients with lung cancer localized to a small pulmonary nodule post surgery is 90% or higher; , survival remains poor (<10%) for patients with distant metastases. While contrast-enhanced CT is preferred to evaluate size, location, and invasion of adjacent structures by lung cancer, PET provides complementary metabolic information. In terms of the primary disease (T status), FDG-PET/CT helps distinguish metabolically active tissue from an adjacent benign process such as atelectasis. For disease that has spread to lymph nodes (N status), an irregular margin or measurement of more than 1 cm in lymph node short axis on diagnostic CT is suggestive of disease spread. FDG-PET/CT is more accurate than CT for evaluating mediastinal disease. However, false-negative results may be seen in the setting of micrometastases or when the primary disease avidity is low and false-positive results may occur in the setting of inflammation or infection. Ultimately, tissue sampling is recommended for the purpose of confirmation. A major strength of FDG-PET/CT is its ability to detect distant metastases (M status). It is estimated that over 50% of patients with lung cancer have metastases at presentation, with common sites including the adrenal glands, liver, brain, and bones. Also, the incidence of occult disease using anatomic imaging is relatively high; in clinical stage I disease it exceeds 10%. , Metabolic imaging can be very helpful in this setting; the sensitivity, specificity, and accuracy of PET for detecting systemic metastases are higher than 90% to identify occult systemic disease on anatomic imaging. To date, PET/CT and PET/MR have shown similar results for lung cancer staging.
PET/CT-based molecular imaging and precision medicine in subsequent lung cancer treatment strategy
Precision medicine integrates the molecular pathobiology and genetic make-up of a disease to suggest targeted therapy, and predict treatment response and outcomes. In this way, “preventive or therapeutic interventions” can be tailored to provide benefit while avoiding the expense and toxicity of futile treatment given to those who will not respond. The goal is to offer the right treatment, to the right patient, at the right time. Tissue biopsy or circulating free-cell DNA is becoming increasingly important to genotype the disease and help select optimal therapy. For oncogene-driven lung cancer that includes any of EGFR, ALK, ROS1, BRAF, TRK, RET, and MET, among others, specific targeted drug therapies may be suggested. For patients that lack an actionable tumor oncogenic driver, immune-checkpoint therapy may be preferred. Discussion is ongoing regarding the best first-line therapy, the potential need for combination therapy and the role of neoadjuvant therapy prior to resection. Further, there remains a risk of recurrence in patients with NSCLC following initial treatment.
PET-based molecular imaging of lung cancer is a predictive biomarker of response to cytotoxic and cytostatic therapy, radiotherapy, and image-guided interventions as well as a biomarker of prognosis . This contributes to precision medicine by influencing the decision to treat and the type of treatment to give. Accurate timely treatment response assessment is critical for adjusting treatment to achieve maximal impact on outcome, although adopting a standardized definition of treatment “success” remains enigmatic. PET is also helpful to detect residual disease following therapy. He and colleagues performed a meta-analysis including 1035 patients and 13 articles. The authors concluded that the pooled sensitivity (95% confidence interval [CI]) for the detection of lung cancer recurrence by PET/CT versus conventional imaging techniques (CITs) was: 0.90 (0.84–0.95) versus 0.78 (0.71–0.84), respectively. The pooled specificity (95% CI) was 0.90 (0.87–0.93) versus 0.80 (0.75–0.84), respectively. According to the Society for Nuclear Medicine and Molecular Imaging, FDG-PET/CT is appropriate for: (1) restaging patients with lung cancer after treatment; (2) detection of recurrence; and (3) treatment response evaluation.
PET as a biomarker of response to lung cancer therapy
The criteria for response assessment have evolved over the years. The first criteria to be widely adopted were based on anatomic findings (ie, WHO, RECIST); however, these had shortcomings for assessing therapy response in lung cancer patients. While cytolytic therapy may cause tumor mass reduction, cytostatic therapy tends to slow or stop tumor cell proliferation without a reduction in tumor mass. More recently, Wahl and colleagues proposed criteria (ie, PERCIST) that included an assessment of metabolic response (PET) to monitor treatment effect. The clinical superiority of FDG-PET/CT as a predictive biomarker of lung cancer response to cytotoxic and cytostatic therapies is largely attributable to the fact that metabolic and pathophysiological changes can precede alterations in lesion morphology. Thus, PET-based molecular imaging may predict treatment response earlier than anatomic imaging modalities (ie, CT and MRI). Further, in general, the earlier the response, the better the progression-free and overall survival of the cancer patient.
The advent of immunotherapy, however, has made response assessment more challenging. Efficacy rests on proliferation of immune cells with tumor infiltration that may lead to a response within days and can include an early, transient, increase in tumor burden and metabolic activity including the development of new lesions, despite apparent clinical benefit. Typically, these imaging findings stabilize or improve after 3 to 4 months. This phenomenon, termed pseudo-progression , may be seen in approximately 10% of patients and has suggested the need for new response assessment criteria in patients treated with immunotherapy. Response assessment criteria such as iRECIST, PECRIT, and PERCIMIT have been proposed. Validation of these criteria and their use in the lung cancer treatment arena remains a topic of current research. Ultimately, correlation of imaging findings with the clinical condition of the patient is key. For example, in patients with lung cancer treated with immunotherapy, imaging progression in the context of an improving or stable clinical condition suggests pseudoprogression. In this case treatment might be continued with response confirmed by follow-up imaging. On the other hand, patients with imaging progression and clinical deterioration are most likely progressing and discontinuing therapy may be warranted. Rarely, patients may experience rapid disease progression or hyperprogression in the setting of clinical deterioration. Also, immune-related adverse events such as thyroiditis, pneumonitis, myalgias, and reactive lymph node enlargement, among others, may be detected with FDG-PET/CT, sometimes weeks before these complications become clinically apparent.
Response Assessment to Chemotherapy and Radiotherapy
Several years ago, PET was shown to be a reliable way to assess response to chemotherapy and radiation treatment. In addition, response on PET, both early in the course of treatment and at the end of therapy, was shown to portend improved survival. There are several supporting studies. Among them, for example, Eschmann and colleagues reported on a study of 70 patients with stage III NSCLC who had FDG-PET before and at the conclusion of neoadjuvant radiochemotherapy. The authors found that a negative PET or a reduction in the standardized uptake value (SUV) by more than 80% at the end of therapy suggested a better outcome. Also, FDG-PET detected residual viable primary tumor with a sensitivity, specificity, and overall accuracy of 94.5%, 80%, and 91%, respectively. MacManus and colleagues showed in their study of 88 patients with NSCLC treated with either concurrent platinum-based radical chemoradiotherapy (73 patients) or radical radiotherapy alone (15 patients), that complete metabolic responders had a 1-year survival rate of 93% compared to 47% for nonresponders, and a 2-year survival rate of 62% versus 30%, respectively. Dooms and colleagues in their study of 30 patients with stage IIIA-N2 NSCLC, found that a decrease of 60% in primary tumor uptake and mild residual FDG avidity in mediastinal nodes after induction chemotherapy were associated with significantly longer 5-year overall survival and benefit. In Hoekstra and colleagues’ study of 47 patients with stage IIIA-N2 NSCLC, a 35% reduction in tumor FDG uptake after one cycle of induction therapy correlated with improved overall survival.
Assessment of Response to Targeted Drug Therapy and Immunotherapy
Studies of targeted drug therapy in lung cancer started to appear in the literature several years ago. In 2004, Paez and colleagues, and Lynch and colleagues showed that the tyrosine kinase inhibitor (TKI) gefitinib stopped the growth of lung tumors expressing somatic mutations of the epidermal growth factor receptor gene (EGFR). , Today, oncogene-targeted drug therapies are often used to treat lung cancer, thus the subclassification of malignancy as a result of identifying genetic mutations ( genomic profiling ) at the time of diagnosis is critical to determining optimal therapy. , ,
With the advent of cytostatic drug-targeted therapy, a change in tumor size was not always consistent with efficacy and the use of RECIST or WHO criteria could underestimate the efficacy. PET-based molecular imaging was thought to be more appropriate to monitor the effect of these therapies. However, the ideal timing of PET during and after targeted therapy, as well as what should be considered a significant reduction in radiopharmaceutical uptake to be consistent with successful response to therapy, remains a topic of debate to this day. Several studies suggest that FDG and FLT uptake at the site of the primary lung lesion tends to increase in nonresponding patients, while earlier and larger reductions in radiotracer uptake with TKI therapy suggest longer survival and better quality of life. Other studies have shown that response to targeted therapy such as erlotinib and gefitinib on FDG-PET can be predicted early in the course of treatment, sometimes as early as 2 days after the start of therapy. , Additional reports have suggested an accurate prediction of response on FDG and FLT-PET was possible within 3 weeks of treatment initiation, as confirmed by conventional imaging and clinical evaluation 4 to 6 weeks later.
More recently, immune-checkpoint inhibitor (ICI) therapy targeting programmed cell death-1 (PD-1) and programmed cell death-ligand 1 (PD-L1) is becoming a prevalent therapy for patients with advanced NSCLC. In 2019, Yu and colleagues studied the association of survival and immune-related biomarkers with immunotherapy in patients with NSCLC through a meta-analysis including 14,395 patients. The authors concluded that immunotherapy was promising in terms of clinical outcome for patients with NSCLC and that the combined predictive utility of PD-L1 expression and tumor mutation burden was associated with prognosis. Several recent studies have assessed the role of PET response to immunotherapy and the potential value of radiomic features for predicting benefit. Currently, the choice of optimal therapy and response assessment (in the case of immunotherapy) remain topics of research.
PET image phenotype as a biomarker of prognosis in lung cancer
The PET image phenotype may facilitate identification of patients at high risk of disease progression regardless of their clinical stage at diagnosis. This information can then be used to personalize therapy. Examples of PET image phenotypes include the: intensity and patterns of tumor metabolic activity (SUV), the metabolically active tumor mass [total tumor glycolysis (TLG)], and tumoral metabolic heterogeneity assessed with texture analysis.
Tumor FDG Avidity
Intensity FDG uptake is associated with lung cancer aggressiveness and has prognostic value. Evidence suggests that high tumor uptake correlates with poor prognosis across different stages and histologic types of lung cancer. Since Ahuja and colleagues suggested primary lung tumor FDG uptake was an independent predictor of overall survival back in 1998, many studies have confirmed a good correlation between outcome and intensity of tumor uptake in patients with early as well as advanced stage NSCLC. For example, Ohtsuka and colleagues found that increased FDG avidity in the primary lung tumor correlated with shorter progression-free survival and a higher rate of recurrence. Based on their results, the authors recommended that in early-stage disease, the intensity of tumor uptake could be used to identify those patients who might benefit from adjuvant chemotherapy. Cerfolio and colleagues analyzed progression-free survival within different lung cancer stages as a function of FDG avidity and concluded that a higher primary tumor uptake within the same stage predicted progression of disease and a shorter overall survival. A National Cancer Institute-funded American College of Radiology Imaging Network/Radiation Therapy Oncology Group study (ACRIN 6668/RTOG 0235) found that in 253 patients with stage III NSCLC and FDG-PET obtained before and at 14 weeks of concurrent platinum-based chemoradiotherapy, a higher posttreatment SUV peak and SUV max were associated with poor survival. Studies have also been conducted to understand associations of FDG avidity with underlying protein expression in lung cancer patients. For example, Nair and colleagues correlated FDG avidity with NK-κBp65 expression and prognosis in 355 lung cancer patients, where NK-κBp65 controls DNA transcription, cytokine production, and tumor cell survival. The investigators found that a high tumor SUV coupled with high NF-κBp65 expression was seen in patients with more extensive disease, tumor invasion, and worse prognosis.
Metabolically Active Tumor Volumes
Tumor bulk is also a prognostic indicator in lung cancer patients. Three-dimensional volumetric indices of metabolic tumor activity can be derived using PET, namely the: (1) metabolic tumor volume (MTV) estimated using semiautomatic tumor-contouring software with a predefined SUV threshold to segment metabolic tumor boundaries and (2) total lesion glycolysis (TLG) estimated by multiplying the tumor’s mean SUV by the MTV. Whole-body MTV and TLG are obtained by summing the values for each lesion in the body. Studies of metabolically active lung cancer volumes suggest this may be a predictor of survival. ,
Metabolic Heterogeneity of Lung Cancer
Tumor heterogeneity has been shown to correlate with aggressiveness, therapeutic resistance, and therefore, poor survival. Various texture parameters can be used to noninvasively characterize tumor metabolic heterogeneity. , There are emerging data suggesting that measures of tumoral metabolic heterogeneity have a prognostic role in lung cancer patients. For example, Cook and colleagues reported on the role of FDG-PET-derived tumor textural features as biomarkers of response and outcomes in 47 NSCLC patients treated with TKIs. The investigators found that reduced metabolic heterogeneity at week 6 during treatment was consistent with a good response to erlotinib confirmed by CT at week 12. In addition, PET image-derived texture parameters were independent predictors of survival. These metabolic signatures of tumor heterogeneity have prognostic potential, although prospective, randomized trials are needed for further evaluation.
Summary
PET imaging has become an integral decision-making tool in the precision medicine algorithm of lung cancer patients. It provides anatomo-molecular insight during the course of care of the cancer patient. This PET-derived information is now used to make treatment decisions, to predict metabolic response during and after therapy, and as a clinical biomarker of survival in lung cancer patients.
Clinics care points
- •
PET and PET/CT imaging are very accurate imaging modalities for the non-invasive metabolic characterization of lung lesions as benign or malignant.
- •
However, FDG uptake in lung lesions is not always specific for malignancy, and therefore, evaluating imaging findings in the patient’s clinical context is critical.
- •
PET and PET/CT are very accurate imaging techniques to stage and restage lung cancer.
- •
PET and PET/CT imaging patterns serve as biomarkers of response to treatment and prognosis.
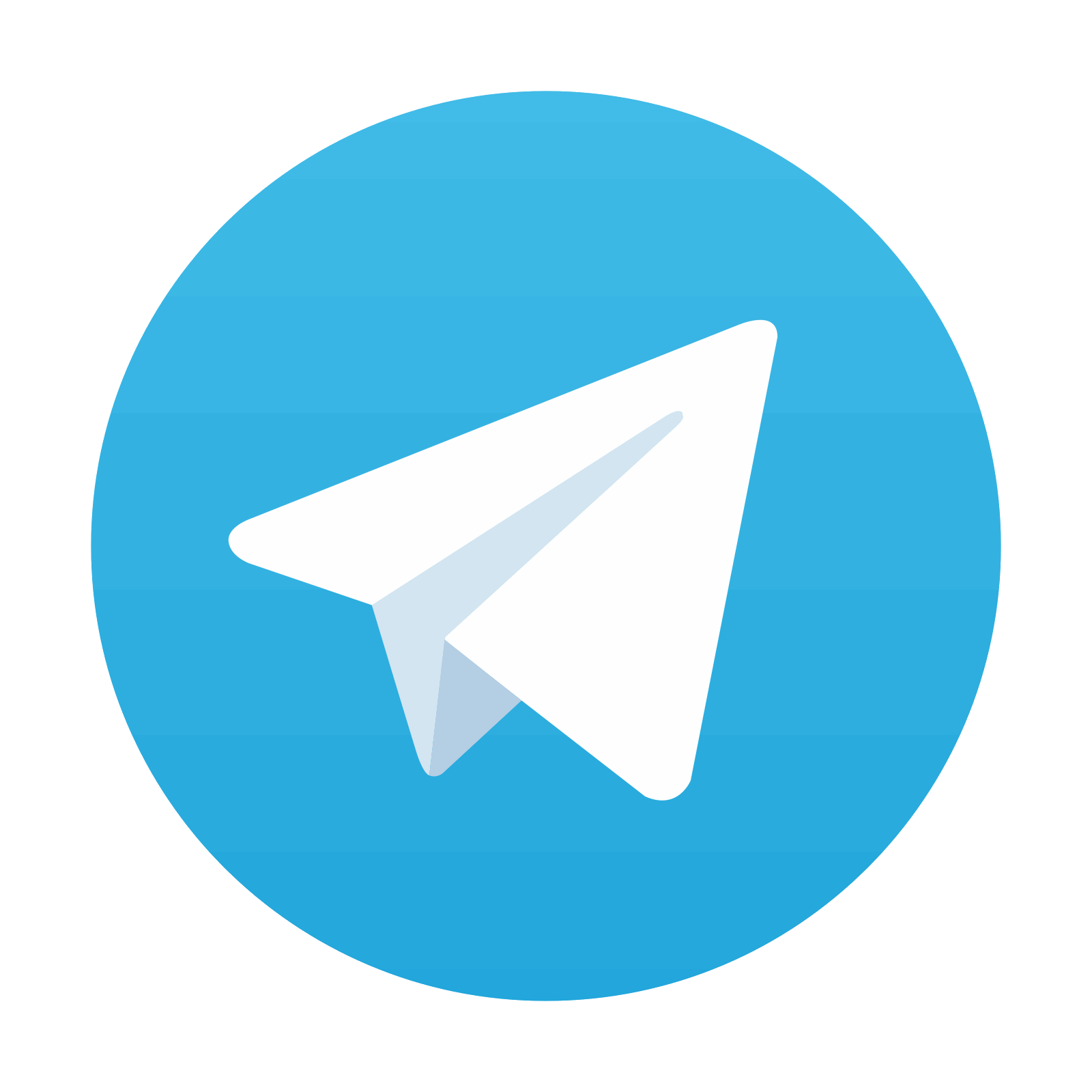
Stay updated, free articles. Join our Telegram channel

Full access? Get Clinical Tree
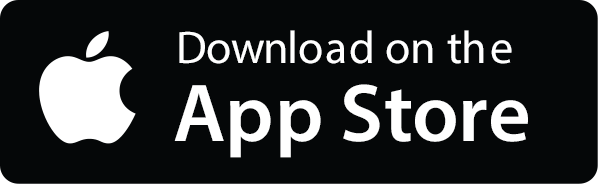
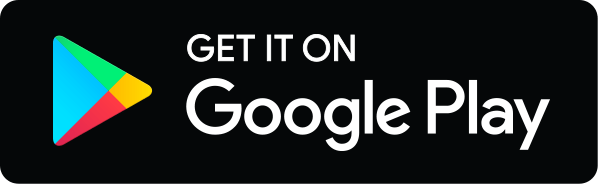
