10
Nuclear Imaging
Thomas A. Farrell
Chapter Outline
Thyroid and Parathyroid Imaging
Angiotensin-Converting Enzyme Inhibitor Renal Scintigraphic Imaging for Renal Artery Stenosis
Ventilation and Perfusion Lung Imaging for the Diagnosis of Pulmonary Embolism
Positron Emission Tomography and PET/CT
Nuclear medicine uses small amounts of radioactive materials (radiopharmaceuticals) to diagnose and treat disease. The subspecialty is unique, because it provides information about both organ structure and function in patients and the techniques used often identify abnormalities very early in the progress of a disease—often before other diagnostic tests. Because the images generated by nuclear medicine represent a physiologic map revealing less anatomic detail than radiologic studies such as CT or MR, it is necessary to correlate the nuclear medicine images with the corresponding radiologic images.
RADIOPHARMACEUTICALS
When molecules with radionuclide components are prepared for administration to human beings, they are called radiopharmaceuticals and they participate in, but do not alter, various physiologic processes. Specific radiopharmaceuticals with particular physiochemical properties are used to study an organ or organ system. The radionuclide portion of the radiopharmaceutical typically emits gamma rays and/or x-rays that can be detected and create a scintigraphic image (often referred to as scan). There are several possible routes of patient administration of radiopharmaceuticals including intravenous, oral, and inhaled.
Over 30 radiopharmaceuticals use technetium-99m (Tc-99m) which has many useful properties as a gamma-emitting tracer nuclide. It is eluted from a Tc-99m generator as the soluble pertechnetate and then either used directly as a soluble salt, or combined with a number of Tc-99m–based radiopharmaceuticals which determine its uptake by various organs. Other radiopharmaceuticals incorporate a radioactive tracer atom into a larger pharmaceutically active molecule, which is localized in the body, after which the radionuclide tracer atom allows it to be detected with a gamma camera. An example is fluorodeoxyglucose (FDG) in which fluorine-18 is incorporated into deoxyglucose to give 18-FDG which is commonly used in positron emission tomography (PET) scanning. Some radioisotopes such as gallium-67 and radioiodine are used directly as soluble ionic salts, without further modification.
The most commonly used nuclear medicine imaging system is a gamma camera which is composed of an array of photomultiplier tubes. Each photomultiplier tube contains a sodium iodide crystal which produces light when struck by gamma or x-rays. The light scintillations are digitized and then processed into an image for physician interpretation. The image is essentially a physiologic map of the radiopharmaceutical distribution within the body. Table 10.1 lists the radiopharmaceuticals and the corresponding imaging procedures that are discussed in this chapter.
Tomography is a basic radiographic technique which improves the visualization of the organ being imaged by blurring or eliminating the adjacent tissue. This technique is widely used in nuclear medicine to improve image quality, for example, single photon emission computed tomography (SPECT) which is an array of gamma cameras mounted on a gantry which rotates around the patient. Resolution of the organ of interest is improved by obtaining images in multiple projections which allows reduction of scatter gamma and x-rays.
SKELETAL IMAGING
Skeletal scintigraphy, more commonly referred to as a bone scan, is a valuable tool for the investigation of a number of disorders of the skeletal system. A Tc-99m–labeled diphosphonate derivative is used in skeletal scintigraphy because this radiolabeled agent is adsorbed onto the surface of newly forming hydroxyapatite crystal in the bone. New bone formation occurs in response to almost all skeletal pathology such as fracture, infection, or tumor and therefore scintigraphic images will demonstrate increased gamma-ray activity at the site of increased bone turnover. The normal bone scan appearance in an adult and child are shown in Figure 10.1. Note the multiple areas of increased activity in the child’s epiphyses.
FIGURE 10.1. Normal bone scans. Whole-body images of a normal bone scan in an adult (A) and child (B). Note the increased epiphyseal activity on the child’s scan.
A bone scan is very sensitive for detecting metastases and in general will identify a lesion before it is seen in a conventional radiograph. However, it is often not possible to determine whether lesions seen on a bone scan are malignant or benign, and this is particularly true for a single lesion, which often is caused by a benign process such as a fracture or infection. The skeletal system is a common site for metastatic spread of many malignancies such as breast, lung, prostate, and renal carcinomas. Because skeletal metastases usually arise as a result of hematogenous seeding of tumor cells in the bone marrow, most bony metastases are detected in the axial skeleton and are seen as numerous foci of increased radionuclide uptake (Fig. 10.2). Well-differentiated thyroid cancer is also prone to disseminate to sites in bone, but these lesions are probably better detected with iodine-131 imaging.
FIGURE 10.2. Bony metastases. Whole-body bone scan in anterior and posterior projections of a 65-year-old man with diffuse skeletal metastases from prostate carcinoma. Images reveal numerous metastatic lesions (black foci), primarily in the axial skeleton and also in the proximal femurs and humeri (arrows).
Table 10.2
Other skeletal abnormalities are also readily detected with a bone scan. Like metastases, osteomyelitis can be detected earlier with a bone scan than with a plain film (Fig. 10.3). The bone scan may also be useful to detect a fracture which may not be easily seen on plain films. For example, stress fractures (Fig. 10.4) and shin splints (Fig. 10.5) are readily detected on a bone scan but may not be seen on a radiograph. In most cases, a fracture through the full thickness of the bone cortex is readily detected by a plain radiograph. Some full-thickness fractures, such as those in the sacrum, scapula, femoral neck, and small bones of the wrist and ankle, are occasionally difficult to visualize on a radiograph but are detectable by a bone scan (Fig. 10.6). Although regarded as a sensitive test, bone scintigraphy may have false-positive and false-negative results (Tables 10.2 and 10.3).
HEPATOBILIARY IMAGING
Patients with acute cholecystitis classically present with right upper quadrant pain/tenderness, fever, and leukocytosis. However, the signs and symptoms of acute cholecystitis often vary and there are a number of conditions that may present in a similar fashion. Consequently, the provisional diagnosis of acute cholecystitis typically requires confirmatory testing with ultrasound and/or hepatobiliary scintigraphy.
Table 10.3
FIGURE 10.3. Acute osteomyelitis. Skeletal scintigraphic images (whole body, A; regional view, B) from an 18-year-old girl with diabetes who presented with 3 to 4 weeks of low back pain. Radiographs of the vertebra were unremarkable. The images show abnormally increased Tc-99m methylene diphosphonate (MDP) activity in the L3 vertebral body (arrow). Biopsy of the site confirmed osteomyelitis. Notice the normal intense uptake of Tc-99m MDP at the growth plates in the lower extremities (arrowhead) on the whole-body images.
FIGURE 10.4. Stress fracture. A 20-year-old runner with pain in the right calf. Radiographs were normal. Scintigraphic images of the distal lower extremities show focal lesion in the posterior medial aspect of the right distal tibia consistent with a stress fracture (arrow S Fx). Notice that the lesion does not involve the full thickness of the tibia. Fibula indicated by arrow Fib.
FIGURE 10.5. Shin splint. Bone scan of a patient with calf pain, showing linear pattern of increased uptake (arrows) along the posterior aspect of the tibia (enthesopathy).
Hepatobiliary scintigraphic imaging is performed using a Tc-99m–labeled iminodiacetic acid (HIDA) derivative that is an analog of bilirubin. This radiopharmaceutical is actively transported into hepatocytes similar to bilirubin and is then excreted unchanged into the biliary tract. Normally HIDA accumulates within the gallbladder within 1 hour of intravenous injection (Fig. 10.7). However, in acute cholecystitis, the gallbladder fails to fill with the radiotracer because of cystic duct obstruction. This test is extremely sensitive, and a normal result (i.e., visualization of the gallbladder) virtually excludes acute cholecystitis.
FIGURE 10.7. Normal hepatobiliary (HIDA) study. Images obtained in the anterior view every 2 minutes (moving from left to right and top to bottom) following injection of the hepatobiliary radiotracer show good extraction of the agent by the liver (L). The common bile duct (arrow CD) is seen along with the duodenum (arrow D) and gallbladder (arrow GB).
False-positive scans are caused by prolonged fasting or recent ingestion of food (Table 10.4). The use of IV morphine has been found helpful in reducing the number of false-positive HIDA scans, thereby improving the specificity of the test. Morphine causes constriction of the sphincter of Oddi, which augments bile flow through the cystic duct, improving gallbladder visualization (Figs. 10.8 and 10.9). HIDA scanning is also useful in the diagnosis of postoperative bile leaks where accumulation of the radionuclide corresponds to that of extrahepatic bile (Fig. 10.10).
FIGURE 10.8. Normal HIDA study with IV morphine in a patient with right upper quadrant pain. A: Initial set of images show normal uptake and excretion by the liver, but over time the gallbladder is not visualized and consequently morphine is given at approximately 40 minutes into the study. B: Images obtained immediately following administration of morphine show the gallbladder visualization (arrow GB), which effectively rules out acute cholecystitis. Note activity in the small bowel (arrow SB).
FIGURE 10.9. Acute cholecystitis. Hepatobiliary study in a patient with fever and right upper quadrant pain. A: Initial set of images show normal uptake and excretion by the liver, but the gallbladder is not visualized and consequently morphine is given at approximately the time of the image at bottom right. B: Images obtained immediately following injection of morphine continue to show absence of gallbladder activity, indicating cystic duct obstruction and acute cholecystitis. Note the reflux of radioactive bile into the stomach (arrow S).
THYROID AND PARATHYROID IMAGING
Graves Disease
Graves disease is characterized by the association of thyrotoxicosis, diffuse goiter, infiltrative ophthalmopathy, and occasionally infiltrative dermopathy. The thyroid scintigram typically shows a symmetrically enlarged gland with homogeneous tracer distribution and a prominent pyramidal lobe (Fig. 10.11) and the patient’s radioactive iodine uptake (RAIU) is increased. While there is no cure for Graves disease, the goal of treatment is to reduce the thyroid’s ability to produce hormones. Three treatment options are available: Medical therapy, radioactive iodine (I-131) therapy, and surgery. I-131 therapy partially destroys the thyroid parenchyma resulting in fibrosis. Studies evaluating the safety of radioactive iodine therapy have failed to show any significant carcinogenic effect in doses used to treat hyperthyroidism.
FIGURE 10.10. Postcholecystectomy bile leak. HIDA scan shows extravasation and accumulation of radiopharmaceutical in the gallbladder bed.
FIGURE 10.11. Graves disease. Tc-99m pertechnetate scan shows a diffusely increased uptake with visualization of a pyramidal lobe (superiorly from the midline). The diagnosis must be confirmed by an elevated radioactive iodine uptake.
Thyroid Nodules
Nuclear imaging can be used to describe a thyroid nodule as hot or cold on the basis of its relative uptake of radioactive isotope such as Tc-99m or Iodine-123. Hot nodules indicate autonomously functioning nodules and cold nodules indicate hypofunctional or nonfunctional thyroid tissue (Fig. 10.12). Radionuclide imaging is unreliable in diagnosing thyroid cancer as 4% of hot nodules are shown to contain tumor, compared with 16% of cold nodules. This technique has largely been replaced by ultrasound and ultrasound-guided biopsy in the diagnostic workup of thyroid nodules. See Thyroid Biopsy in Chapter 12.
FIGURE 10.12. Thyroid cold nodule. There is a focus of reduced uptake in the mid right thyroid lobe (arrow).
FIGURE 10.13. Parathyroid adenoma in a patient with hypercalcemia. Delayed imaging after Tc-99m sestamibi injection shows increased uptake in the right neck consistent with a parathyroid adenoma.
Parathyroid Imaging
Tc-99m sestamibi is absorbed more quickly by a hyperfunctioning parathyroid gland than by a normal gland. Over 60% of parathyroid adenomas may be successfully imaged using this technique. Chief cell parathyroid adenomas in particular have a very high avidity for sestamibi, in contrast to oxyphil/clear cell parathyroid adenomas which have almost no avidity. Images of the neck and chest are obtained immediately and 2 hours postinjection of Tc-99m sestamibi (Fig. 10.13). Smaller-volume parathyroid adenomas, those glands in the upper position, and patients with multiglandular disease are all less likely to be reliably imaged with sestamibi scans.
RENAL IMAGING
Tc-99m dimercaptosuccinic acid (DMSA) localizes in the proximal and distal convoluted renal tubules and is the tracer of choice for renal parenchymal and anatomical evaluations (Fig. 10.14). It is not suitable for dynamic perfusion studies. On the other hand, Tc-99m diethylenetriamine pentaacetic acid (DTPA) is the tracer of choice for dynamic renal perfusion and the detection of renal and ureteral obstructions. About 95% of the dose is filtered through the glomeruli with a normal mean renal transit time of 3 minutes. At 2 hours there is less than 10% renal retention.
FIGURE 10.14. Renal DMSA scan shows upper pole cortical loss in the right kidney (arrowhead).
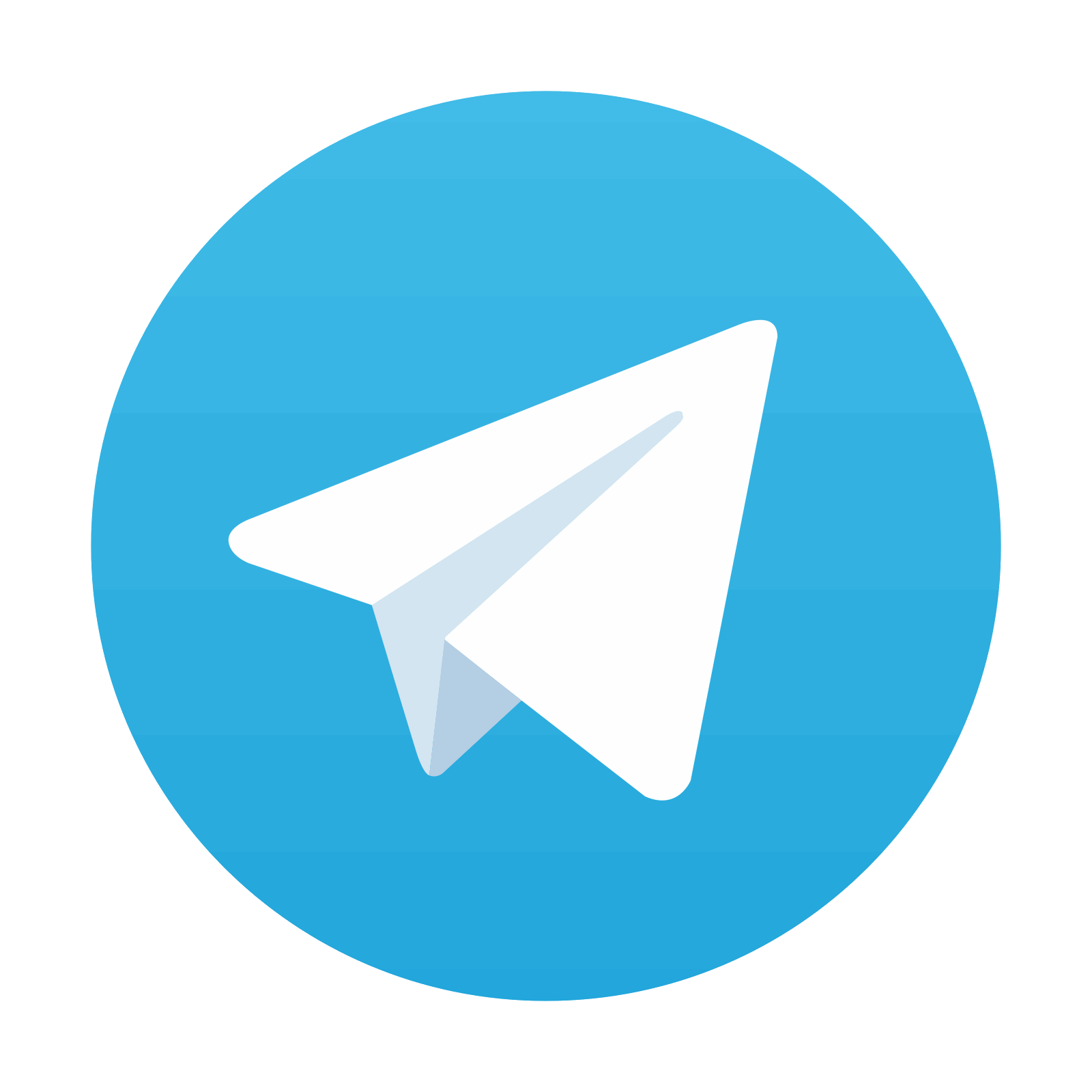
Stay updated, free articles. Join our Telegram channel

Full access? Get Clinical Tree
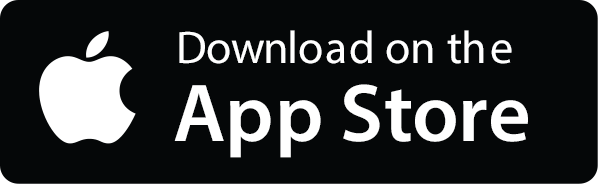
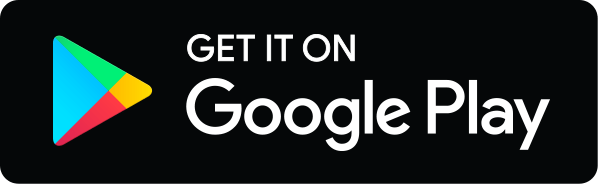