Tell me and I forget. Teach me and I remember. Involve me and I learn.
31.1 Introduction
Perceptual scientists have learned much about how humans perceive medical images. Until recently, there has been limited application of this knowledge in developing educational tools for healthcare practitioners. This chapter will examine the historical and current models for training in medical image interpretation. Subsequently, we will review how our knowledge of medical image perception may be useful for developing rational educational tools to help healthcare practitioners learn how to evaluate medical images. Simulation and computer algorithms as a means of deploying perceptual training tools will also be discussed, as well as obstacles to implementation.
31.2 Historical Training Methods Image Interpretation in Medicine
Many healthcare practitioners view medical images. To date, most of the research on medical image perception has focused on radiologists. Radiologists are physicians with a primary area of practice being the interpretation of medical images. As defined by the American College of Radiology (ACR), “Radiologists are medical doctors (MDs) or doctors of osteopathic medicine (DOs) who specialize in diagnosing and treating diseases and injuries using medical imaging techniques” (ACR, 2017). Many healthcare practitioners view medical images and may act on their own interpretation. However, radiologists are generally the physicians who provide the official interpretation of a medical imaging study.
31.2.1 Radiologists
All physicians seeking to become a radiologist in the USA must first complete medical school and obtain a medical doctorate, doctorate of osteopathy, or an equivalent non-US degree. After completing their general medical training, radiologists work 1 year in clinical medicine as an intern. An intern may be defined as “a resident member of the medical staff of a hospital, usually a recent medical school graduate serving under supervision” (Dictionary.com, 2017).
After completing internship, diagnostic radiologists in training enter a residency program in radiology lasting typically 4 years. Residency is basically an apprenticeship during which residents learn the skills needed to become a radiologist. During residency, residents learn about: the physics of medical imaging and imaging modalities, imaging of normal anatomy, imaging of the spectrum of disease processes throughout the body, and appropriate management recommendations. After completing residency, many radiologists complete an additional 1 or 2 years of postresidency subspecialty training called a fellowship. The educational process for a radiologist is summarized in Figure 31.1. Note that the training for interventional radiologists, radiologists who spend a significant portion of their time performing procedures, will differ. During and after training radiologists must take a series of examinations to be eligible to practice.
Figure 31.1 Pathway for a physician to become a radiologist. DO, doctor of osteopathic medicine; MD, doctor of medicine.
Note that the training for radiology residents and fellows is similar, but differs in the depth of training. Radiology residents receive greater breath of training and fellows greater depth. Future descriptions of training will be made with reference to radiology residents only. It should be noted that, with minor differences in the number of years spent in residency and fellowship, other clinicians who interpret medical images as part of their daily practice (e.g., pathologists, ophthalmologists, those practicing telemedicine) also go through similar general training.
Until approximately 2000, radiology residencies and fellowships followed a similar training model for many years. The primary mechanism of education was, and still is, teaching via an apprenticeship model where residents work side by side with a more senior faculty radiologist who supervises the residents’ work. The typical workflow (Figure 31.2) is for a resident to review a set of cases, generate a preliminary report, and then review this report with the faculty radiologist. The faculty radiologist then critiques the resident’s work, providing input on relevant image findings, differential diagnosis for these findings, an overall interpretation of the study, as well as pertinent management recommendations for the referring healthcare provider.
Figure 31.2 Typical case/image review workflow for a radiology resident.
While many would argue that the apprenticeship method provides the best opportunity to learn radiology, there are several disadvantages. The most notable is that residents will only gain experience with the cases they see as part of their work day. It may be that a residency program may not get adequate case volume in particular disease processes, and limit the educational experience of residents. There is considerable variation in the case mix between training programs, which may be partially due to climate, local industries, patient demographics, and patient referrals. Another disadvantage is that the resident learning will depend on the expertise of the faculty radiologists. If there is a rare case and the faculty radiologist is not familiar with the disease process involved, the resident’s learning experience may be less than optimal.
Residents supplement their education while not in the reading room via several means. Radiology residencies typically contribute to their residents’ education by didactic lectures. In addition to lectures, residents will often have case conferences led by a faculty radiologist. During case conferences, unknown cases are presented, residents describe the findings, and offer differential diagnoses and management recommendations. Residents can also supplement their learning through reading textbooks and journal articles in their time outside of work.
Since about 2000, the increasing prevalence of computers for personal use and online educational resources has revolutionized both education in general and radiology education in particular. Now radiologists are able to use online case files and other electronic media to supplement their learning. This allows residents to gain exposure to cases of disease processes that may not occur in adequate numbers at their training institution.
It is important to note that these learning resources are generally based on understanding and describing a disease process and its imaging findings. Little emphasis is given to how the image findings for each modality are perceived (or not) by the human visual system, missing educational opportunities to improve detection and classification of potential abnormalities.
31.2.2 Nonradiologists
Nonradiologist healthcare providers (Table 31.1) often need to evaluate radiologic images. These providers will typically not render the formal interpretation of an imaging study. However, they may provide preliminary interpretations overnight when the radiologist is not evaluating images. Additionally, the nonradiologists may need to understand the findings of an imaging study to be able to direct therapy and refine medical decisions.
Table 31.1 Examples of nonradiology healthcare providers who may need to evaluate medical images
• Physicians in practices other than radiology |
• Physician assistants |
• Nurse practitioners |
• Respiratory therapists |
• Physical therapists |
• Radiology technologists |
The training of nonradiologists at image interpretation is limited and dependent on the specialty of interest and the individual training program. Radiology education in medical school is often directed toward fundamental concepts that most practitioners should understand. Such concepts include when to order an imaging study, basics of physics for radiography (e.g., chest, abdomen and bone computed/digital radiography), and key image findings. There is significantly less training on cross-sectional imaging modalities such as computed tomography (CT) or magnetic resonance imaging. Nonphysicians typically receive even less formal exposure to radiology images, principles, and training.
Nonradiologists will often receive more focused training in interpretation of examinations they will encounter as part of their work (e.g., orthopedic surgeons will get significant exposure to bone images). This often occurs during an apprenticeship period after their degree program, similar to the radiologist residency. Note that there is typically less of an emphasis on imaging interpretation, which is taught by individuals who may themselves lack formal imaging training.
31.3 Simulation for Training in Medicine and Radiology
Simulation has proven to be a way to safely train specific skill sets in an environment similar to practice. Simulation has become a useful tool for training in several fields, aviation being a notable example (Allerton, 2010). Simulation has found application in medicine in many fields, often related to performing physical procedures as in surgery (Samia et al., 2013).
Simulation has recently been used for radiology education. To date, simulation has focused on noninterpretive radiology skills such as interventional radiology procedural skills (Meng and Lipson, 2011), and response to adverse reactions to contrast for CT and magnetic resonance examinations (Sarwani et al., 2012).
To date there has been limited emphasis on using simulation for training to improve nonprocedural skills such as image interpretation. This is somewhat surprising, as it is well known that the repetition of an activity will on average improve learning and retention.
To date, when simulation has been used in the realm of image interpretation, it has been used largely as a method to augment case volume. For instance, simulation has been used as a tool to train residents for taking overnight call (Towbin et al., 2008). While such applications harness the attributes of simulation, they do not encompass our knowledge about the image perception process, again missing out on educational opportunities.
31.4 Perceptual Training and its Prior Applications
Perceptual training in radiology has existed for many years, although it is not formally thought of as such. During a typical radiology residency, a trainee learns several “tips and tricks” for avoiding perceptual pitfalls. However, they are generally not referred to as perceptual training. In addition, the techniques taught, while valid, do not result from a systematic review of the perceptual literature or understanding of the human eye–brain system. Historical perceptual training techniques were obtained from anecdotal (albeit valid) experience of practitioners.
As an example, for many years radiologists have been aware of the presence of Mach bands. Mach bands are visual illusions occurring at the neuronal level via lateral inhibition. Basically the visual system uses filtering to enhance edges like an edge enhancement algorithm hard-wired into our eyes (Chasen, 2001). Radiologists teach their trainees to be aware of such artifacts, and look for specific imaging and clinical findings to compensate for them. One location where Mach bands are commonly seen is on the odontoid view of cervical spine radiographs. On such radiographs, the bottom of the skull often projects over the base of the odontoid process (a part of the second cervical vertebra). The resultant Mach band at this interface may simulate a fracture, as shown in Figure 31.3. Radiologists instruct their trainees to look for other findings suggestive of a fracture, such as discontinuity of the bone cortex and/or trabeculae to confirm the presence of a fracture. In the absence of these findings, radiology residents are instructed that the apparent dark line is probably due to a Mach band, a perceptual illusion.
Figure 31.3 Mach band at the base of the odontoid process (arrow).
Another example is the background effect. The background effect occurs when the perceived intensity of a structure is influenced by the intensity of its surroundings (Buckle et al., 2013). An example of the background effect is shown in Figure 31.4. In this figure, a renal lesion appears as low attenuation on a contrast-enhanced CT due to the surrounding enhanced renal parenchyma. A nonenhanced CT better demonstrates the lesion to be of indeterminate attenuation. This illusion is rather problematic, as CT can be used to differentiate lesions as benign or malignant based on X-ray attenuation (which correlates with pixel brightness on CT images). For this reason, radiologists tell trainees they should explicitly measure the pixel attenuation and not rely on their perception of the image alone.
Figure 31.4 Background effect. Top, the right renal lesion (arrow) appears to be low-density on contrast enhance image. Bottom, the lesion (arrow) has indeterminate attenuation (near unenhanced kidney) on noncontrast image.
There are a few other examples where perceptual knowledge is applied to the instruction of medical trainees. It is important to note, however, that there has never been a systematic study of the perception literature to look for areas where our perceptual knowledge may be applied to the education of medical trainees and personnel. To date, perceptual understanding has generally been applied to medical image interpretation training in a rather nonsystematic manner. Our fund of knowledge regarding medical image perception is large and growing. It is feasible to translate specific aspects of this knowledge to educational methods. Recent research has been performed identifying key areas where our perceptual knowledge may be used to develop improved educational tools.
One area where educational tools were specifically developed based on perceptual knowledge is the recent work on search pattern training for chest radiography.
One method for characterizing perceptual errors was developed by Kundel et al. (1978), where they proposed classifying perceptual errors as search, recognition, and decision errors. In this study, it was estimated that 30% of errors were due to search errors. It has been shown that search errors are partially due to failure of observers to move their high-resolution, central foveal gaze over the lesion of interest, especially novices (Kundel and La Follette, 1972). Thus, one of the reasons pulmonary lesions are believed to be missed, especially in novices, is due to inadequate visual search.
Consider the potential search pattern of a novice evaluating a chest radiograph with a simulated nodule in the right upper lobe (Figure 31.5). The novice’s search pattern is incomplete as it does not include the right upper lobe, resulting in the novice failing to identify the pulmonary nodule.
Figure 31.5 Potential incomplete novice search pattern (long white arrow starting at •, and ending at ▶), missing the simulated nodule right upper lobe (arrowhead).
All health professionals who examine medical images are novices at some point in their careers, and ways to decrease these errors would be helpful. One method is to teach a search pattern that ensures full coverage of the chest with the central part of the field of vision. This may initially seem straightforward, but is easier said than done. Radiology programs have encouraged trainees to look at “everything” for decades, yet the novice missing a nodule continues to be an issue.
Radiology training programs have attempted to help trainees “look at everything” by teaching search strategies for evaluation of various types of medical images. For example, one frequently used search strategy for chest radiography proposed by Felson (Goodman, 2014) is to look at the abdomen, bony thorax, mediastinum, and lungs, as summarized in Table 31.2. However, this strategy is essentially an ordered checklist of structures to evaluate. This does not explicitly tell a trainee how to look at each structure in question. That is, trainees are instructed to evaluate the lungs, but a specific pattern for evaluation of the lungs is not suggested. This is one example of how radiology residents are encouraged to fully evacuate medical images, without formally being told exactly how to accomplish this goal.
Table 31.2 Typical conventional radiology search strategy, an ordered checklist of structures to evaluate
Trainees should look at the: |
• Abdomen |
• Bony thorax |
• Mediastinum |
• Lungs |
An informal unpublished survey of medical trainees asked whether or not medical trainees had ever been shown a specific eye movement pattern for evaluation of chest radiographs. The overwhelming majority of respondents said they had not. Further anecdotal data gathered by speaking with medical trainees at various levels of training indicated that virtually none of the trainees had ever been shown a specific eye movement search pattern for evaluation of medical images. Trainees stated that they arrived at their search patterns on their own, essentially through trial and error, with too much of the latter.
Given how critical it is for novices to perform a comprehensive search of an image, this is rather surprising. Leaving the development of a comprehensive search pattern to individuals to figure out on their own does not seem to be the optimal method for ensuring high-quality image interpretation. Consequently, it seems reasonable to hypothesize that teaching comprehensive search patterns would decrease the false-negative rate when evaluating medical images.
31.5 Teaching a Search Pattern
To address the need for residents to learn comprehensive search patterns, a study was performed in which medical trainees were taught a specific eye movement search pattern for evaluation of chest radiographs (Auffermann et al., 2015). The goal of this research was to determine if showing a specific eye movement pattern could improve their ability to identify pulmonary nodules on chest radiographs.
Medical trainees with some limited training in chest radiography were selected. Most were medical students. Subjects were divided into two groups, control and experimental. All subjects participated in this protocol individually, with one-on-one interaction with the study proctor. Subjects were shown chest radiograph images at a simulated radiology workstation and were asked to identify and mark pulmonary nodules. Subjects were also asked to give an estimate of their confidence in the nodule markings. After the first set of cases, the experimental group was shown a suggested search pattern for evaluation of a chest radiograph.
The search pattern instructed subjects to sweep their eyes along each intercostal space for both the right and left hemithoraces (shown in Figure 31.6), essentially a modification of the boustrophedon scan pattern. Subjects following this scan pattern would have the central portion of their field of view (corresponding to the fovea) cover the entire lungs, decreasing one of the many potential sources of perceptual error.
Figure 31.6 A recommended chest radiograph search pattern.
After search pattern training or the attentional control, a second set of cases was shown to both groups with the same tasks to perform. After the second set of cases, the control group also received search pattern training. Subsequently, a third set of cases was shown. Using this counterbalanced design, all subjects received search pattern training, and commented on their impression of its effectiveness as a training tool. Note that the third set of cases was not analyzed, but given to allow both control and experimental groups received educational benefit from this study.
Performance on case sets 1 and 2 was compared using localization receiver operating characteristic (LROC) analysis and area under the LROC curve (AUC). Results are summarized in Table 31.3, showing a statistically significant improvement in performance for the experimental group, but not for the control group.
Table 31.3 Results of search pattern training1
Group | Case set 1 | Case set 2 | △AUC | 95% CI | p-value |
---|---|---|---|---|---|
Control | 0.6662 | 0.7184 | 0.0521 | –0.0570–0.1530 | 0.191 |
Experimental | 0.6944 | 0.8024 | 0.1079 | 0.0111–0.2119 | 0.015 |
1 Note that the numbers given under case sets 1 and 2 represent the respective areas under the location-specific receiver operating characteristic (LROC) curves (AUC); CI, confidence interval.
While eye movements were not tracked, these results suggest that the proffered search pattern altered visual search behavior in a positive way to reduce false negatives. In fact, several subjects anecdotally stated that, after search pattern training, they saw many more potential lesions, and it was difficult to determine which were real. This suggests that AUC analysis may actually underestimate the improved lesion detection, as the AUC measure incorporates false positives. However, further study is needed before such statements can be made definitively.
31.6 Deployment of Search Patterns in an Educational Laboratory
The previously described search pattern training for pulmonary nodules was repeated in an educational laboratory (Auffermann et al., 2016). This study was conducted with physician assistant students. Unlike the previous study, where each subject had individual interaction with the study proctor, this study was conducted in computer classrooms where there were 24 subjects for each study proctor, with a total of 48 subjects in this study. The motivation for this study was to demonstrate that search pattern-training techniques may be used in conventional educational environments while maintaining the educational benefit.
As in the prior study, the goal was to determine if search pattern training at simulated radiology workstations improved subjects’ ability to identify pulmonary nodules on chest radiographs. The subjects were instructed to identify and mark pulmonary nodules and indicate their diagnostic confidence in the nodule being present. The computers used in this study were similar to the computers used by nonradiologists for image viewing. Once again, after the first set of cases, the experimental group was shown a suggested search pattern for evaluation of the lungs, and the control group was not. Results in nodule identification were similar to the earlier study. Subjects receiving search pattern training showed a statistically significant improvement in performance, while subjects not receiving search pattern training did not.
These results show that not only is search pattern training a useful educational tool, but it can be deployed in conventional educational settings using the computer equipment already present in many educational computer classrooms.
31.7 Trainee Feedback on Search Pattern Training
For both search pattern training studies, a survey was given to subjects after completion of the study. Survey topics are shown in Table 31.4. The goal was to determine how subjects felt about search pattern training and the use of simulated radiology workstations as an educational tool. Subjects were asked to respond to the statements using a 5-point Likert response format, where 1 = strongly disagree, and 5 = strongly agree. The results were analyzed using the Wilcox sign rank test. Recall that both control and experimental subjects received search pattern training, although the timing of their training differed.
Table 31.4 Questionnaire for search pattern training studies
Item# | Questionnaire statement |
---|---|
1 | The search pattern training was helpful for learning the skills needed to identify pulmonary nodules on chest radiographs (CXR) |
2 | The search pattern training has helped me feel more confident about my ability to identify pulmonary nodules on CXR |
3 | The radiology simulation environment (RSE) used for this study was helpful for learning the skills needed to identify pulmonary nodules on CXR |
4 | Compared with this study’s educational slides, the RSE provided a more effective way to develop the skills needed for pulmonary nodule identification on CXR |
5 | Compared with conventional learning materials (including printed/electronic textbooks and case files), the RSE provided a more effective way to develop the skills needed for pulmonary nodule identification on CXR |
6 | I think that an RSE would be a helpful way to learn about additional topics in radiology |
7 | I think that a simulation environment would be a helpful way to learn about additional topics in medicine in general |
8 | My participation in this study was an overall positive experience |
The responses were statistically significant for all statements. Subjects thought search pattern training was a useful adjunct to their educational curriculum, that simulation was a useful adjunct to training, and that it would be a useful way to learn additional high-yield skills in radiology.
31.8 Future Applications of Search Pattern Training
The results of these search pattern-training studies suggest several things. The first is that explicit search patterns are not being taught to medical trainees. As discussed earlier, the search strategies typically taught to trainees in the USA usually consist of an ordered checklist of structures to evaluate. Trainees are typically not taught how to move their eyes to evaluate a specific structure.
Conversations with the study participants indicated that they felt that there is inadequate focus on teaching comprehensive search patterns to healthcare trainees. The general consensus seemed to be that being specifically shown how to move one’s eyes to evaluate an organ system would be a useful adjunct to conventional training for many types of imaging studies and disease pathologies.
For example, search pattern training could potentially be used in abdominal imaging to follow the bowel to look for abnormalities, or in neuroimaging to provide a comprehensive evaluation of the cerebral hemispheres. The number of imaging studies, organ systems, and diseases make development of search patterns for every potential eventuality challenging. However, there are several commonly encountered examinations and disease entities for which search pattern training may be of educational value.
31.9 Simulation to Augment Training of Critical Perceptual Skills
The search pattern training studies discussed earlier also suggest that, in addition to teaching a search pattern, the simulated radiology workstation used to teach and assess for efficacy provided a helpful environment for trainees to practice their new skills. Conventional radiology educational media focus on delivery of information, but with less emphasis on the application of this information. Historically, practicing to develop such skill sets was reserved for the clinical work day. However, several studies have shown that simulation may provide a useful means to practice specific high-yield skills outside the workplace (Desser, 2007). In addition to being able to teach specific search patterns, practicing these techniques in a controlled simulation environment may provide a good venue to learn more skills and more rapidly develop expertise.
It is worthwhile noting that simulation, employed to its optimum capacity, may differ from real-world practice in key areas. In fact, one of the strengths of simulation is to rapidly increase expertise in specific high-yield areas. Simulated environments may be tailored to train and access high-yield tasks that are critical for individuals to perform their job safely and effectively, and to devote less time to tasks that are less critical.
Consider the simulation training a pilot receives. It has been estimated that pilots devote approximately 75% of their simulation training to taking off and landing an aircraft. This is in stark contrast to the relatively small fraction of a total flight devoted to these activities. The reason for this inequality is that takeoffs and landings are critical times during a flight and approximately 61% of accidents occur during this time period (Boeing, 2017).
It can be argued that the same is true of medical and radiology training and simulation. Medical trainees gain most of their experience developing perceptual expertise during the work day. For a radiology resident on a chest rotation, this may mean interpreting 50–80 chest radiographs in a day, although the actual number will vary by training program and individual resident. The number of radiographs interpreted by nonradiologists would likely be less. During the clinical work day the trainee has many tasks to perform, including: check the patient’s medical history, evaluate the image, compare with prior studies, formulate an interpretation of the study, document findings, and communicate with the referring medical providers as needed. While these aspects of a resident’s education are reflective of clinical practice and a necessary part of training, this may not be the best way to train high-yield perceptual tasks.
Instead, perhaps a portion of a healthcare practitioner’s training could be dedicated to gaining proficiency at high-yield tasks. For example, a respiratory therapist or physician’s assistant is likely to be exposed frequently in practice to chest X-ray exams; thus, being able to recognize something as critical as a malpositioned central venous catheter or breathing tube is a high-yield task where this type of dedicated training would be useful. Deploying targeted perceptual training techniques in a simulated environment may provide the optimum platform for gaining proficiency at high-yield tasks quickly. Consider the previously discussed studies on search pattern training for nodule identification. Offering such training at simulated radiology workstations provides the opportunity to hone a critical skill in a safe environment, where patients will not be harmed. Upon gaining proficiency in the simulated environment, the trainee may be better situated to employ the new interpretive skills in a clinical environment.
Much conventional educational media is static (although this is changing). Textbooks and journal articles are a resource for information, but without active involvement. Simulation as a means of conveying perceptual skills may be a helpful means to involve trainees and facilitate learning.
31.10 Future Directions
The application of our knowledge about perception to developing new training algorithms has been limited to date. It is probable that much of the knowledge we have about medical image perception may be used toward the development of training tools.
For example, it is known that when recognition and decision false negatives occur, the observer tends to fixate on the abnormality longer than true-negative areas (Manning et al., 2004). True and false positives also are associated with increased fixation times, but tend to be longer than false negatives. When I was a trainee, one of my supervising radiologists would jokingly say, “If I look at something on an image for more than 30 seconds, I call it abnormal.” While this was stated in jest, there may be a kernel of wisdom worth considering. Trainees could potentially be told that the longer they evaluate an area, the more likely they are to have a false-negative, as well as a false-positive, decision. Potential educational interventions could be developed to help the trainee in such circumstances: specific imaging signs to look for, things to consider in the patient’s history, and ways to further manage the patient that will not result in the unneeded use of medical tests and resources.
Such educational methods could be deployed using conventional educational media, including textbooks, journal articles, and static media on the internet. However, a more pragmatic means of deployment may be using simulation software. As in the studies cited previously, perceptual training can be conducted at simulated radiology workstations, with practice cases tailored to reinforce perceptual training techniques.
To date, radiology educational software has largely used static media, although this is changing. Practice cases have long been a component of radiology education, deployed in both paper and electronic format, yet the mode of interaction with the cases generally lacks the instructiveness that is typical at the radiology workstation during the clinical work day. Only recently has the radiology educational community begun to include educational packages with a greater degree of interaction.
One example is the emergent/critical care imaging SIMulation (SIM) (University of Florida, Gainesville, FL) (SIMulation, 2017). This package uses software to simulate a radiology workstation and assess radiology residents for readiness for call. Unlike many other educational tools for radiology, this package uses full Digital Imaging and Communications in Medicine (DICOM) data sets with abnormal and normal cases, more closely simulating the practice environment when compared with many other conventional educational media and platforms. One could envision a similar product used not only for assessment, but for teaching medical trainees key skills for image interpretation. It also seems plausible that, by using perceptual training, we may discover new aspects of medical image perception that were not known previously.
31.11 Steps Toward Implementation and Acceptance
Several steps are required before we can readily deploy simulation tools. These include, but are not limited to, development of perceptual training tools, availability of training tools to trainees, and acceptance of perceptual training via simulation as an adjunct to learning.
31.11.1 Development of Perceptual Training Tools
Perceptual training has only recently been formally realized as a potential means for developing educational tools. However, the software needed for deployment of these tools has not been developed yet. Consequently, software solutions will need to be developed. While several radiology software products exist, their development may be somewhat slower relative to nonmedical software products, perhaps due to the time and effort needed to develop suitable software, collect relevant cases, and disseminate the software product.
31.11.2 Availability of Perceptual Training Tools for Trainees
Once perceptual training tools have been developed, they need to be made readily available to medical trainees. It is important to note that such training methods would be useful to any healthcare professional needing to evaluate a medical image (Table 31.1), not just radiologists.
In addition to high-performance computers, a simulated radiology workstation requires appropriate monitors and the software to simulate a radiology viewer, an interface that records decision responses, the means to provide feedback regarding performance, and the ability to generate, save, analyze and summarize performance results.
The typical resolution of diagnostic (nonmammography) radiology monitors 3 megapixels (MP). Most standard computer monitors are on the order of only 2 MP. However, the monitors used clinically by nonradiologists are rarely diagnostic-quality monitors, with resolution on the order of 2 MP. Consequently, the hardware requirements for an educational computer laboratory would likely be satisfied by monitors already in many, if not most, educational laboratories. However, it is important to note that monitors for many laptop and tablet computers may not be of sufficient resolution for adequate simulation fidelity.
The software needed for simulation is something that is not currently routinely available, at least in radiology. The option exists for institutions to develop their own simulation software and case database files. However, such an undertaking would be rather laborious and would be more feasible for larger programs with significant resources at their disposal. Smaller programs would probably not be as well situated to develop their own simulation software and case databases, and may be more reliant on commercial solutions. Consequently, the availability of commercial software for perceptual training may be a limiting factor to widespread adoption.
31.11.3 Acceptance of Perceptual Training via Simulation
Medicine is a rather conservative discipline that is slow to adopt new ideas, and with good reason. Rapid acceptance of new ideas without appropriate validation may result in patient harm. Consequently, healthcare practitioners are cautious about making changes. As a result, changes in healthcare tend to be evolutionary, not revolutionary.
Many physicians stress the importance of experience acquired during the clinical work day as being the core aspect of medical training. This may be especially true of radiologists who believe, with good reason, that there is no substitute for real-world experience. Deploying these tools using a simulated radiology workstation may lead some more traditional educators to question the value of such an intervention. The goal of simulated perceptual training is to complement traditional educational tools in specific high-yield areas, not supplant them. Consequently, it is probable that, even if there is resistance to adoption within the radiology community, this is a barrier that can be overcome.
Nonradiologists who receive relatively little radiology training are less likely to find simulation an obstacle. Given that the amount of hands-on radiology training for nonradiologists is often limited, perceptual training deployed via simulation is more likely to be seen as a useful and helpful adjunct to training in a much-needed area.
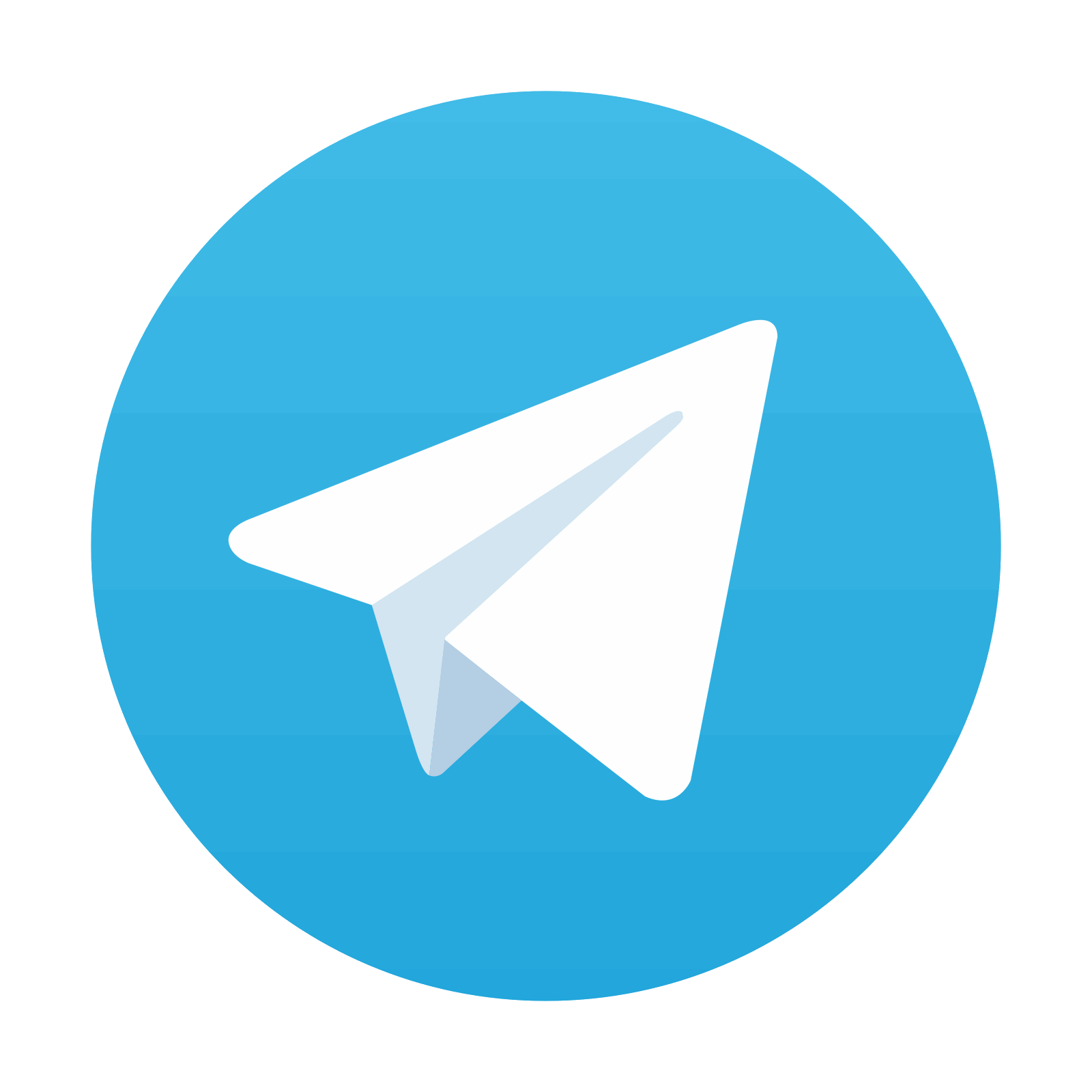
Stay updated, free articles. Join our Telegram channel

Full access? Get Clinical Tree
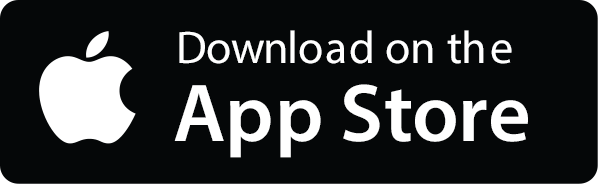
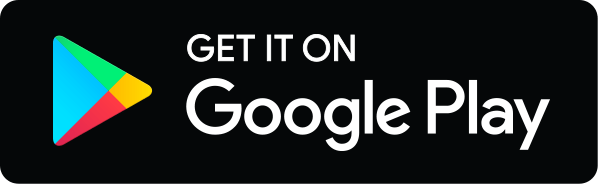
