4 Lungs
Introduction
The respiratory tract is divided into upper and lower parts. The upper respiratory tract includes the organs outside the thorax including the nose, pharynx, and larynx which are discussed in other chapters. The lower respiratory tract contains the organs within the thorax including the trachea, bronchi, bronchioles, alveolar duct, and alveoli. This chapter reviews the anatomy of the lung with emphasis on the lobes, segments, subsegments, and pulmonary lobules.
Embryology
During weeks 3 to 4 of gestation, the respiratory system develops from the ventral wall of the foregut. The epithelium of the trachea, bronchi, and alveoli originate from the endoderm, and the muscle, cartilage, and connective tissue originate from the mesoderm. 1 , 2 , 3 , 4 The tracheobronchial tree develops between days 24 to 36 of gestation during which a median bulge develops on the ventral wall of the pharynx at the laryngotracheal groove (Fig. 4‑1). By day 28, the bulge has formed into the right and left lung buds. These buds continue to elongate forming the primary and segmental bronchi. The human lung develops through multiple stages during gestation: the embryonic, pseudoglandular, canalicular, saccular, and alveolar. The alveoli continue to proliferate until 300 million (32 m2) have formed, usually by age 8. 1 All preacinar (conducting) airways will develop by week 17 which is the end of the pseudoglandular stage. Acinar contents develop mainly during the canalicular and saccular stages. Development of the pulmonary circulation occurs in parallel with lung development (see Chapter 2, Tracheobronchial System). The pulmonary artery develops from the sixth aortic arch. The distal right pulmonary artery loses its connection with the arch, whereas on the left it maintains connection by the ductus arteriosus. The pulmonary veins derive from the splanchnic mesoderm that overlies the foregut and their development is closely related to the development of the lung buds. Blood returning from the lung buds initially drains into the splanchnic plexus but later a primitive common pulmonary vein appears and connects the lung venous network to the left atrium along with obliteration of the majority of the splanchnic pulmonary connections. Four pulmonary venous tributaries of the primitive pulmonary vein subsequently develop and incorporate into the left atrium.
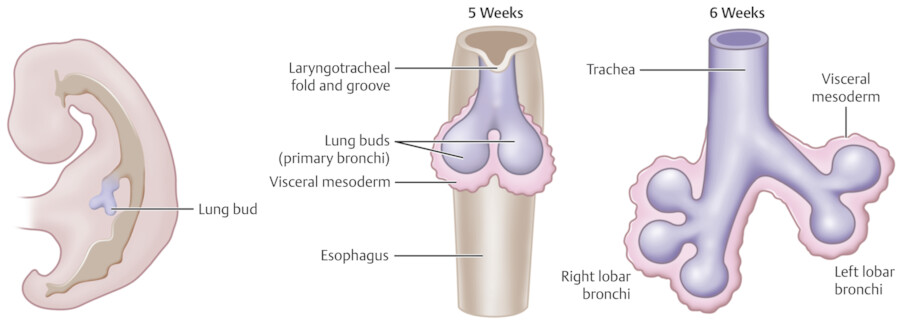
Anatomy
Lobes and Segmental Anatomy
Knowledge of the normal lobar and segmental anatomy is essential for understanding their variable appearances on chest radiography and CT imaging and for localizing various abnormal findings (Fig. 4‑2 , Fig. 4‑3). Typically, the right lung consists of three lobes: the upper, middle, and lower lobes. The right upper and middle lobes are separated by the minor or horizontal fissure, and the right middle and lower lobes are separated by the major or oblique fissure. The left lung typically has two lobes: the upper and lower lobes, which are separated by the major or oblique fissure. A fissure is made up of a double layer of visceral pleura that separates pulmonary lobes, however, it may be incomplete or absent. An incomplete major fissure may lead to disease spread because the lung parenchyma of the adjacent pulmonary lobes fuse. 5 The major fissures are usually incomplete toward the mediastinal side near the hilar area and in the upper parts.
Each lobe is divided into bronchopulmonary segments, based on the tertiary (segmental) bronchi supplying each segment (Fig. 4‑3). Each segment is supplied by its own artery and bronchus. The segmental anatomy is somewhat symmetric, even though the right lung consists of three lobes and the left consists of two lobes. There are 10 bronchopulmonary segments in each lung, but in left lung, some of these segments fuse to form 8 segments total. The bronchi continue to divide into smaller and smaller bronchi up to 23 generations of divisions from the main bronchus. On the right, the right upper lobe consists of the apical, posterior, and anterior segments, the right middle lobe consists of the lateral and medial segments, and the right lower lobe consists of the superior, medial, anterior, lateral, and posterior segments. The left upper lobe consists of the apicoposterior, anterior, superior lingula, and inferior lingula segments, and the left lower lobe consists of the superior, anteromedial, lateral, and posterior segments. 6
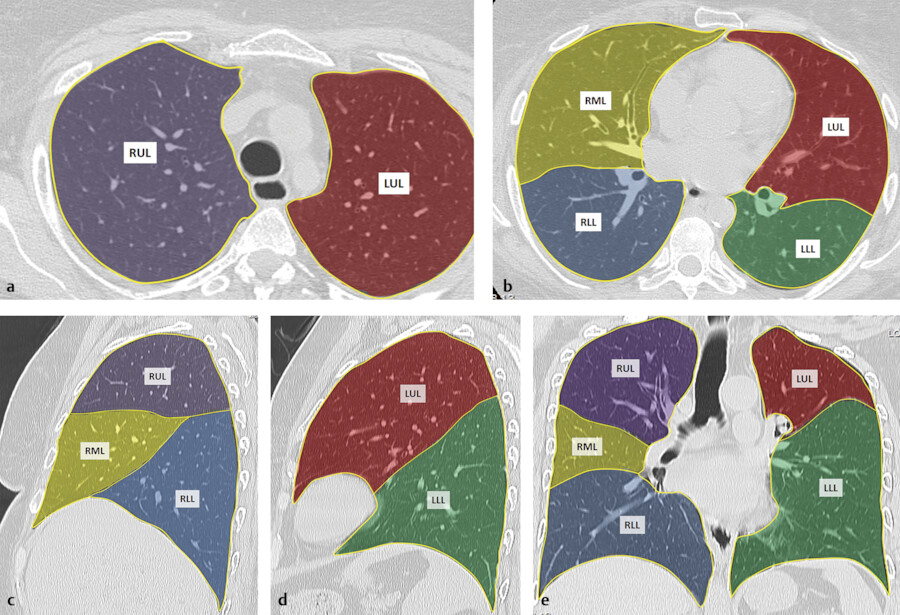
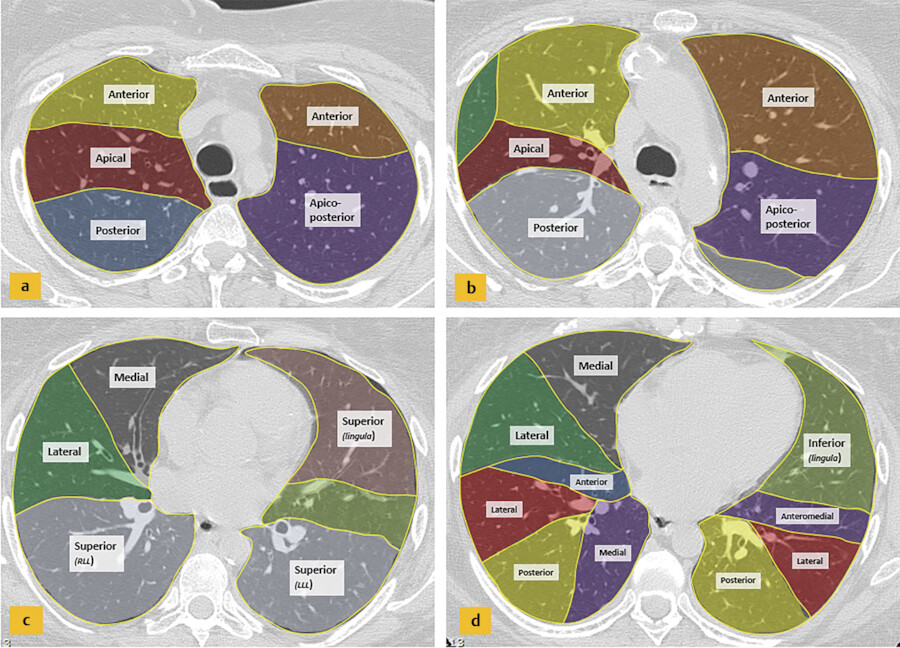
Secondary Pulmonary Lobule and Pulmonary Acini
The secondary pulmonary lobule (SPL) is a fundamental structure of the lung, and knowledge of its anatomy helps in the interpretation of both normal and abnormal CT scans. The tertiary bronchi subdivide into the bronchioles down to the level of the secondary lobules where the terminal bronchioles are located. The terminal bronchioles (final section of the conducting airway) are lined with simple cuboidal epithelium. The diameter of bronchioles is approximately 0.3 to 2 mm. The respiratory bronchioles are subdivisions of the terminal bronchioles which connect with the alveolar ducts and sacs that are responsible for gas exchange. The SPL is the smallest unit of lung structure marginated by connective tissue septa, 7 and each bronchopulmonary segment is made up of multiple SPLs. The SPL is generally polyhedral in shape and varies in size, from 1 to 2.5 cm. 7 At the center of the SPL, run a lobular (preterminal) centrilobular bronchiole and a small pulmonary artery branch. Within the connective tissue septa that marginate the SPL run the veins and the lymphatics 8 , 9 , 10 (Fig. 4‑4 , Fig. 4‑5). It should be noted that the size and shape of the SPL on CT images are affected by the orientation of the lobules in relation to axial slice.
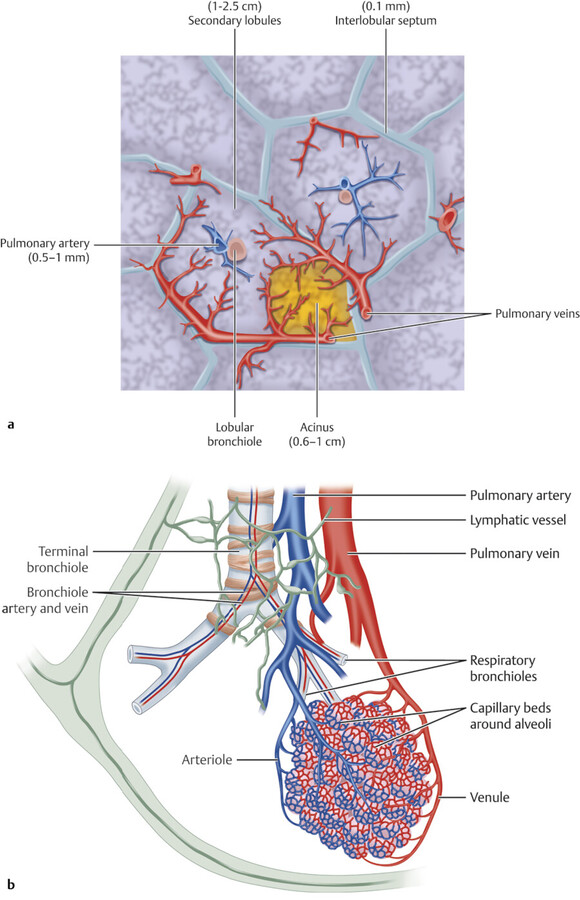
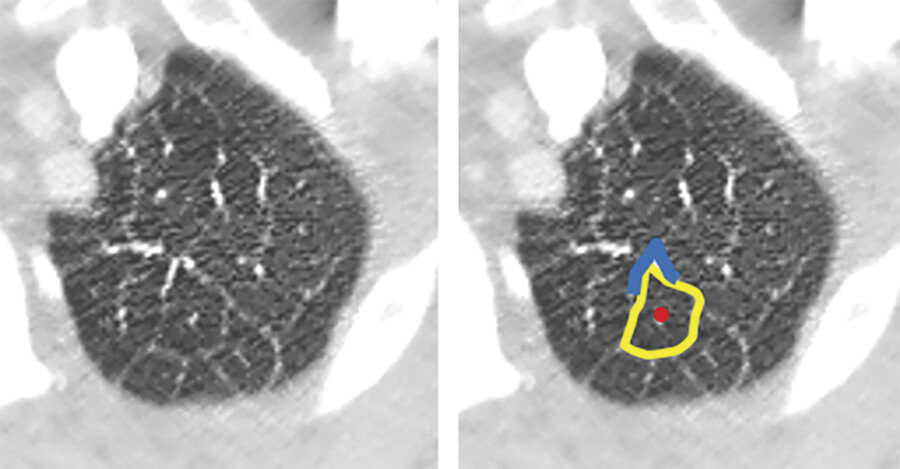
A SPL is made up of smaller structural units called acini. The pulmonary acinus is defined as the structure distal to a terminal bronchiole. It is supplied by a first-order respiratory bronchiole. The number of acini forming the lobules ranges from 3 to 24. 10 Since respiratory bronchioles contain alveoli in their walls, an acinus is described as a unit of lung structure in which all airways participate in gas exchange. Acini can range in size from 6 to 10 mm with a mean acinar volume of 187 mm3. 11 , 12 The size of vessels supplying this unit is less than 150 µm.
Interalveolar Air Drift
In 1931 Van Allen and Lindskog showed intercommunication at the periphery of the bronchial tree allowing transfer of gases and fluid between alveoli. 13 These collateral channels are called pores of Kohn which connect alveoli to adjacent alveoli and the canals of Lambert which connect terminal bronchioles to the alveoli (Fig. 4‑6). Collateral channels prevent collapse of the alveoli in case of proximal bronchial obstruction and lung can preserve its ventilation function. Collateral air drift can also occur in the fused lungs at the areas with defective fissures. 14
Interfissural collateral air drift becomes evident if one lobe is collapsed due to obstruction of the lobar bronchus. The portion of the collapsed lobe fused with the unobstructed lobe remains aerated by the airflow across the incomplete major fissure (Fig. 4‑6). The middle lobe has reduced collateral ventilation which predisposes it to complete atelectasis and disease 15 (Fig. 4‑7).
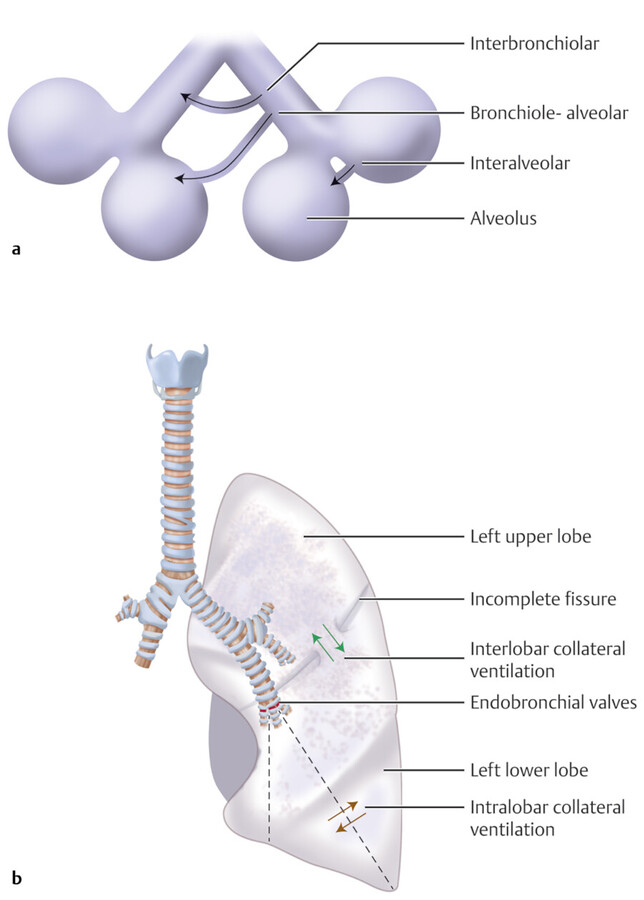
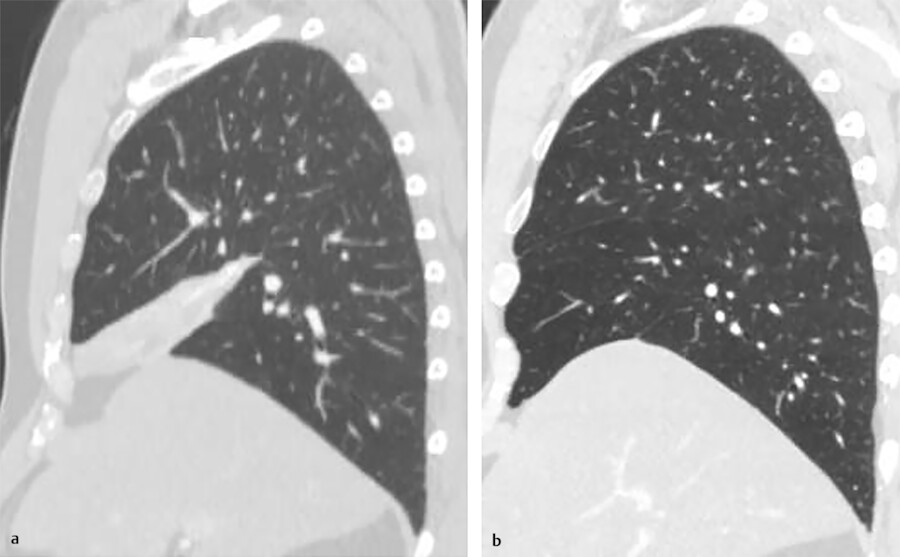
Collateral ventilation does not substantially contribute to lung function in the normal lung because of the considerably high resistance to collateral ventilation in the normal lung. 16 On the contrary, the resistance to collateral ventilation will be diminished when bronchial obstruction exists, which facilitates collateral ventilation to the diseased lung. 17 In patients with bronchiolitis obliterans, collateral aeration can persist over a period of years causing emphysema and hyperlucent lung. 18 Collateral ventilation through an incomplete fissure may cause problems during bronchoscopic lung volume reduction by hampering the collapse of a nonfunctioning lung segment. 17 Recognition of an accessory fissure may alter the surgical strategies for a lung lesion, because an incomplete fissure may contribute to postoperative air leakage.
Pulmonary Function
Understanding of lung volumes, lung compliance, pulmonary perfusion, microcirculation, and ventilation–perfusion relationship are essential for clinical application of respiratory physiology. The main function of the lung is establishing a highly effective gas exchange. For this purpose, ventilation and perfusion of the lung need to match. The portion of ventilation which reaches the alveoli and participates in the gas exchange is called alveolar ventilation. Normal value of alveolar ventilation matches the cardiac output and both are approximately 5 L/min.
Lung Ventilation
Gas moves from the outside to the acinus by convection and diffusion. Convective transportation of gas occurs within the conducting airways. At the acinus level, conductive gas transport stops as gas transport is primarily by diffusion and alveolar ventilation. The location of this transition is called the diffusive front. 19
Normal tidal ventilation which is approximately 4-8 ml/kg-m is required for normal gas exchange. 20 , 21 Vital capacity of the lung is defined as a full inspiratory breath followed by expiration to reserve volume which is approximately 4 to 5 L in an average 70-kg individual. The residual volume of air remaining in the alveoli after vital capacity breath is called residual volume. Functional residual capacity (FRC) is the amount of air in the lungs after a normal expiration which is the residual volume plus expiratory reserve volume and measures between 2.8 and 3.1 L in standing position. Gases remaining in the lungs at the end of expiration not only prevent alveolar collapse but also continue to oxygenate the pulmonary blood.
Compliance is the ability of lung to distend. It is expressed as the distension of lung for a given level of transpulmonary pressure. In normal respiration, negative pleural pressure is sufficient to distend the lungs during inspiratory phase. Compliance depends on the volume of the lung. Compliance is minimum at extremes of FRC. In other words, an expanded lung or a completely deflated lung has the lower capacity to distend to a given pressure. In the upright lung, the intrapleural pressure becomes positive from apex to base of lung. It is –8 cm of H2O at the apex and –1.5 cm H2O at the base. As a result, in upright position apical lungs are less compliant and therefore less ventilated. Ventilation pattern changes with the position of individual because of the change of pleural pressure with gravity. Change in body position from upright to supine, lateral, or prone reduces FRC in dependent lungs which promotes early airway closure and decreases ventilation in the dependent regions. Since lung blood flow passes preferentially to dependent regions, ventilation and perfusion mismatch occurs in these regions. 21
Lung Circulation
The flow of blood between the heart and the lungs is achieved by two separate pathways, the pulmonary circulation and the bronchial circulation. 22 , 23 Pulmonary circulation develops early. By day 34 in embryogenesis, circulation exists between the primitive heart and the lung buds. The pulmonary circulation primarily provides blood oxygenation, whereas the systemic (bronchial) circulation is the major supply to nourish the airways and lungs. This dual input perfusion concept is important for imaging analysis of pulmonary perfusion and characterization of intrapulmonary pathologies especially lung masses. Malignant tumors, especially larger ones, may be supplied by both bronchial and pulmonary circulations. It is therefore suggested that including both arterial flows in perfusion measurements of lung tumors may be more accurate. Many conditions, including pulmonary embolism, pulmonary hypertension, chronic obstructive disease and tumors, alter pulmonary perfusion. Furthermore, pulmonary perfusion changes after surgical lung resection and during physiological processes, such as change of body position or during physical activity. Therefore, assessment of the pulmonary perfusion is of physiological and clinical importance. It is important to know that large vessels serve to conduct blood flow throughout the lung, do not participate in gas exchange, and therefore do not represent pulmonary perfusion. Multiple approaches to assess pulmonary perfusion have been used. Computed tomography (CT), magnetic resonance imaging (MRI), and single-photon emission computed tomography (SPECT) all have been successfully used to measure lung perfusion.
The pulmonary vascular circuit runs in parallel to the systemic arterial circuit but in much lower pressure than the systemic circuit. The pulmonary vessels are thin-walled and have less musculature to assist fast diffusion of gases. Despite their low pressure, pulmonary circuit is a high-flow circuit, receiving the entire volume of cardiac output with each stroke. The low pressure of the pulmonary circuit is the effect of low resistance of the pulmonary microcirculation, thanks to its denser vascular network, offering a larger surface area compared to systemic circulation. Because of low pressure and structural differences of pulmonary vasculature, they change with gravity.
The distribution of blood flow in the pulmonary arteries depends on three factors: alveolar pressure, pulmonary arterial pressure, and pulmonary venous pressure. In apical regions, alveolar pressure is higher and arterial blood flow is lower, and this zone is considered as physiological dead space. The patients on positive pressure ventilation with positive end-expiratory pressure (PEEP) may have larger apical zone due to high alveolar pressure. Pulmonary artery pressure is high during exercise, eliminating any existing dead space in the apical regions. Hypoxic pulmonary vasoconstriction is a compensatory mechanism that shifts blood flow away from hypoxic lung regions to better oxygenated regions.
The relative contribution of blood within the lung tissue is high. The lung density at functional residual capacity is approximately one-third of that of the brain and the blood volume itself contributes to more than one-half of the weight of the lung. The lung density is even higher (more capillaries) in dependent lungs compared to nondependent regions.
Pulmonary Artery Microcirculation
Pulmonary microcirculation consists of small arterioles and capillaries (Fig. 4‑4). This microcirculation network comprises approximately 25% of lung volume and is designed to handle the entire cardiac stroke volume which permits proper gas exchange. 24
Small pulmonary arterioles contain smooth muscle fibers and thus have a level of control over blood distribution, acting as resistance vessels and allowing for the shunting of blood within the microcirculation. Even smaller capillaries arise from the branching arterioles, which then form a network of vessels surrounding the alveoli. These capillaries have very thin walls, less than 0.1-µm thick, permitting gas exchange and oxygenation of blood. In addition to gas exchange, the capillaries play a vital role in chemical substance exchange, such as water and electrolytes, as well as biochemical destruction and synthesis. For example, the vasoconstrictive hormone angiotensin I is converted to the active angiotensin II at the pulmonary capillary level. After gas exchange occurs, blood then continues within the pulmonary venous system for ultimate return to the heart.
Bronchial Artery Circulation
Bronchial circulation differs from pulmonary circulation in that it carries oxygenated blood on the arterial side, arising from the aorta. Bronchial circulation supplies the trachea and bronchi, bronchovascular bundles, pulmonary interstitium, lymph nodes, visceral pleura and the vasa vasorum of the aorta, pulmonary artery, and pulmonary vein. 25
Bronchopulmonary Arterial Collateral Flow
Several studies have demonstrated the presence of collateral circulatory pathways between the pulmonary arterial system and bronchial arterial system early in life, but in the normal adult lung, this system recedes. 26 While likely still present, there is no demonstrable flow within the collateral vessels.
However, anastomotic flow has been observed in cases of diseased lung, such as in cases of fibrotic interstitial lung disease, 25 atypical mycobacterial infection, or lung cancer. The result is much higher-pressure systemic blood flows (bronchial) entering the lower-pressure pulmonary circuit. The result of which is vascular breakdown and ultimate hemoptysis. In cases of pulmonary embolism, flow through these channels can compensate for lack of pulmonary flow and explains why pulmonary infarction is seen in only a minority of cases.
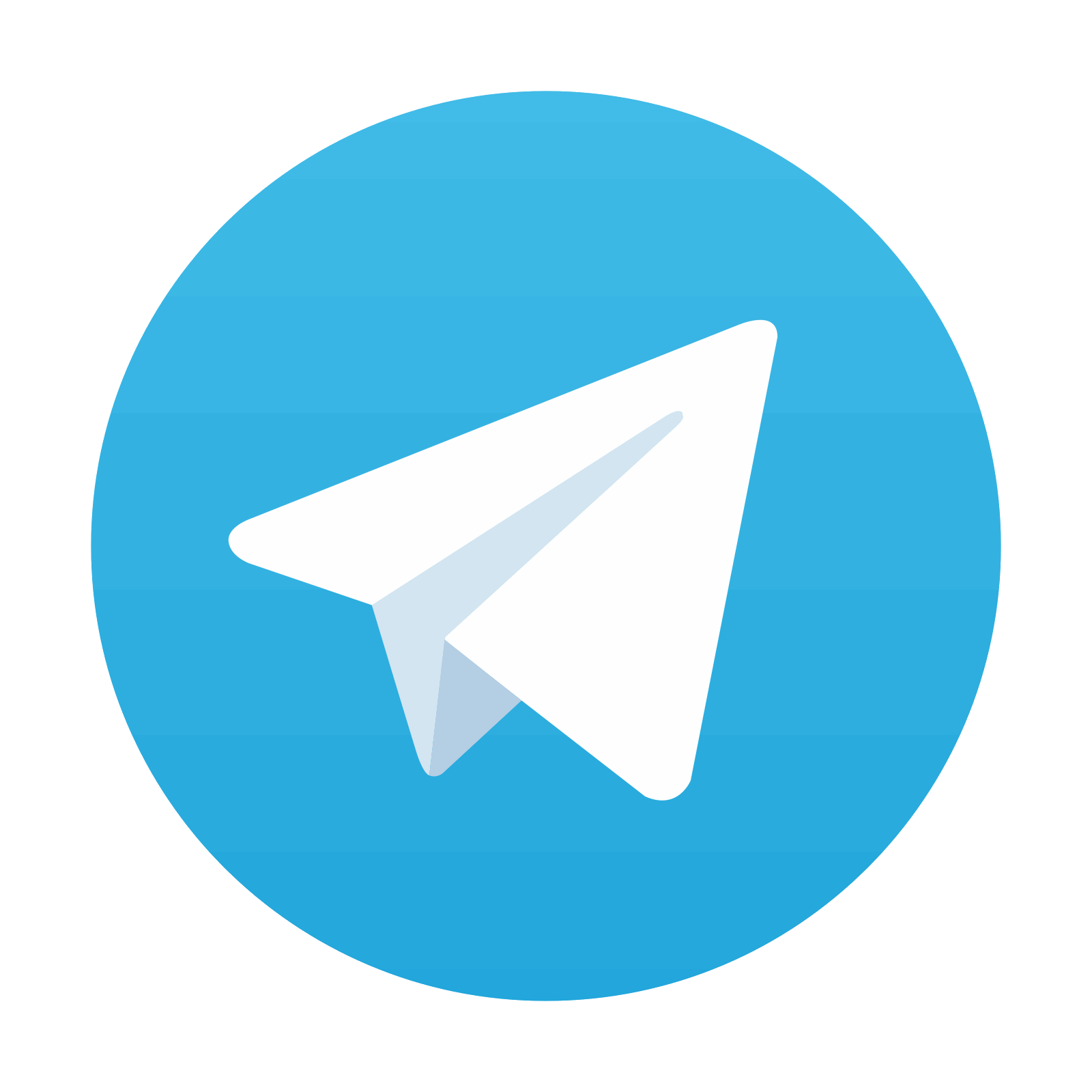
Stay updated, free articles. Join our Telegram channel

Full access? Get Clinical Tree
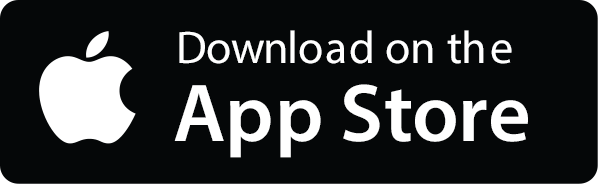
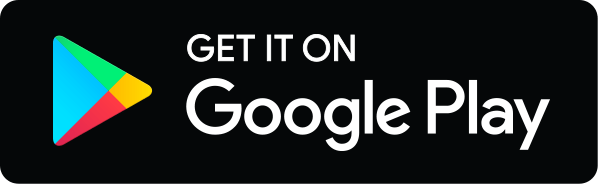
