4 Spine
Approach
The aim of spinal imaging in acute trauma is to detect fractures, evaluate their stability, and identify associated soft tissue injuries. Other emergent indications include atraumatic pain (usually in the setting of an underlying malignancy or predisposition to infection) and acute myelopathy or radiculopathy.
CT with multiplanar reconstructions is significantly more sensitive and specific for detection and exclusion of cervical spine fractures than plain radiography and is now considered the standard of care in acute trauma. Five specific clinical criteria—validated by the National Emergency X-ray Utilization Study (NEXUS) and widely used in emergency departments and trauma centers—permit the emergency physician to exclude cervical spine fracture without imaging:
No midline tenderness
No focal neurologic deficit
Normal alertness
No intoxication
No painful distracting injuries
MRI is indicated for patients with neurological deficits, prolonged obtundation, or unstable spinal injuries. Flexion and extension radiographs are never indicated in the emergency setting, as muscle spasm can mask ligamentous instability and truly unstable injuries can be made worse.
Both the thoracic and lumbar spine are normally included on chest and abdomen CT in the setting of polytrauma, and as long as high-quality reformations are available, a separate dedicated spinal examination need not be performed. Plain radiographs are usually adequate to screen for thoracic and lumbar spine injuries in patients with low-energy mechanisms, with CT to follow if an abnormality is identified.
To avoid missing craniocervical dissociation injuries, which can be subtle, several measurements in the cervical spine are useful. The tip of the basion should be within 9.5 mm of the tip of the dens on CT (12 mm on lateral radiograph). The tip of the basion should be between 6 and 12 mm from a line drawn along the posterior cortex of C2 (the posterior axial line). This is referred to as the basion–axial interval. Injury to the transverse ligament, which affixes the dens to C1, increases the atlantodental interval, which should be less than 3 mm in adults and 5 mm in children on both CT and radiographs.
In the lower thoracic and lumbar spine, the interspinous distance and interpedicular distances on frontal radiographs and coronal CT increase by about 2 mm at each level from craniad to caudad. Isolated widening of either of these distances at a single spinal level should raise suspicion for fracture. Disk herniations should be identified when present and can be practically described as either (1) broad-based disk bulge, (2) central, paracentral, foraminal, or lateral protrusion, or (3) extruded or sequestered disk, in which disk material is separated from the body of the disk.
Anterior compression and burst fractures can be described by the ratio of the affected vertebral body height to the average height of the adjacent superior and inferior vertebral bodies; for example, if the adjacent vertebral body height average is 25 mm and the affected vertebra is 20 mm, it has a 20% loss of height. Canal compromise can similarly be expressed as a percentage of the expected canal diameter at the affected level.
Anatomic Checklist
Cervical Spine
Prevertebral soft tissues
Anterior spinal alignment
Posterior spinal alignment
Spinolaminar alignment
Basion–dens interval (< 12 mm on radiograph, < 9.5 mm on CT)
Basion–axial interval (< 12 mm on radiograph, 6–12 mm on CT)
Atlantodental interval (< 3 mm)
Occipital condyle–lateral mass relationship
Dens–lateral mass relationship
Facet articulations
Vertebral bodies
Vertebral artery canal
Intervertebral disks and uncovertebral articulations
Spinal canal
Spinal cord and epidural soft tissues
Neuroforamina
Thoracolumbar Spine
Paraspinous soft tissues
Anterior spinal alignment
Posterior spinal alignment
Intraspinous distance
Interpedicular distance
Vertebral bodies
Intervertebral disks and facet articulations
Transverse processes
Spinal canal
Spinal cord and epidural soft tissues
Neuroforamina
Imaging
Cervical Spine Radiographs
Indications: Pain, radiculopathy. Trauma significant enough to warrant imaging should be evaluated by CT.
AP
Lateral
Odontoid
Oblique
Thoracic Spine Radiographs
Indications: Minor trauma, some patients with pain.
AP
Lateral
Lumbar Spine Radiographs
Indications: Some patients with minor trauma and lower back pain. Generally not indicated for uncomplicated lower back pain in patients under 50 or without a history of underlying malignancy.
AP
Lateral
Sacrum
Oblique lumbar radiographs are rarely indicated
Spine CT
Indications: Trauma, radiculopathy.
Technique: 0.6-mm dataset with 2-mm axial, 2-mm sagittal, and 2-mm coronal reformations. Images localized to area of clinical concern. Postcontrast images can be obtained for osteomyelitis/diskitis or epidural abscess when MRI is contraindicated or not available.
Spine MRI
Indications: Neurologic deficit in trauma, radiculopathy, pain, and suspected ligamentous injury.
Sequences: Sagittal T1, sagittal T2, axial T1, axial T2.
Options: T1+gadolinium sequences can be obtained if there is concern for osteomyelitis/diskitis, epidural abscess, or tumor. Fat suppression techniques (STIR, T1 with fat saturation) are also commonly used.
Clinical Presentations and Differential Diagnosis
Clinical Presentations and Appropriate Initial Studies
Cervical Trauma
Cervical spine CT
MRI for suspected spinal cord injury, ligamentous injury or epidural hematoma
– Occipital condyle fracture
– Atlantoaxial dissociation
– Rotatory subluxation of C1 on C2
– Dens fractures
– Hangman fracture (C2)
– Flexion injury
– Extension injury
– Unilateral or bilateral jumped facets
Thoracolumbar Trauma
Thoracic or lumbar spine CT (or reconstructions/reformations from multidetector chest/abdomen CT)
MRI for suspected spinal cord injury, ligamentous injury, or epidural hematoma.
– Anterior compression fracture
– Burst fracture
– Chance-type fracture
– Flexion-distraction injury
– Fracture-dislocation
Pain
No imaging study for acute back pain in patients under 40 without trauma or other comorbid conditions.
MRI and CT can be considered for pain unresponsive to conservative measures or for patients with underlying malignancy or risk of infection.
– Muscle spasm
– Disk herniation
– Degenerative disk disease
– Primary or metastatic bone tumor
– Spondylolysis/spondylolisthesis
– Osteomyelitis diskitis
Myelopathy
MRI with or without gadolinium. CT with contrast may be considered if MRI is contraindicated.
– Acute or subacute cord compression
– Pathologic fracture
– Primary spine tumor
– Osteomyelitis/diskitis
– Epidural abscess
– Multiple sclerosis/demyelination
– Spinal cord ischemia
– Infectious myelitis
– Radiation myelitis
– Spinal cord neoplasm
Radiculopathy
MRI with or without gadolinium. CT with contrast may be considered if MRI is contraindicated.
– Herniated disk
– Degenerative disk/facet disease
– Spinal canal/neuroforaminal stenosis
– Schwannoma
– Meningioma
Differential Diagnosis
Collapsed Vertebra
Metastasis
Multiple myeloma
Lymphoma
Osteoporosis
Trauma
Osteomyelitis/diskitis
Enlarged Vertebra
Paget disease
Aneurysmal bone cyst
Hemangioma
Giant cell tumor
Extradural Mass
Extruded disk
Metastasis
Plasmacytoma
Lymphoma
Hematoma
Abscess
Meningioma
Intradural Mass
Meningioma
Metastasis
Intramedullary Mass
Astrocytoma
Ependymoma
Hematoma
Infarct
Intramedullary Signal Abnormality
Demyelinating disease/transverse myelitis
Contusion
Neoplasm
Ischemia
Craniocervical Junction Injuries
Atlantooccipital dislocation (AOD) is a consequence of very high-energy trauma; usually diagnosed at autopsy rather than on imaging studies. In AOD, the skull is dislocated with respect to the lateral masses of C1, and this can be directly assessed on CT by evaluating the congruity of the atlantooccipital articulations. Displacement can be along either the transverse or craniocaudal axis and is usually associated with marked prevertebral soft tissue swelling.
Complete AOD (dislocation) is a fatal injury, but partial AOD (subluxation) may be compatible with life. To ensure detection of an unstable but potentially survivable injury, the atlantooccipital articulation should be specifically evaluated on any lateral radiograph or cervical spine CT obtained for trauma. The most useful measurements are the basion–dens interval, the basion–axial interval, and the atlantodental interval, which assesses integrity of the transverse C1 ligament.
Occipital condyle fractures, considered rare prior to the advent of CT, are diagnosed with increasing frequency. Depending on the mechanism of injury, several types of occipital condyle fracture have been identified.
Type I: impaction fracture of the occipital condyle due to axial loading
Type II: basilar skull fracture, usually due to direct impact, that extends to the occipital condyle
Type III: avulsion injury at alar ligament attachment due to bending and rotation
In Type I and II injuries the alar ligaments and tectorial membrane are intact, and these fractures are generally stable. Because the alar ligament is disrupted in Type III fractures, they are potentially unstable. Type III fractures are more likely to be associated with lower cranial nerve deficits ( Fig. 4.1 ).
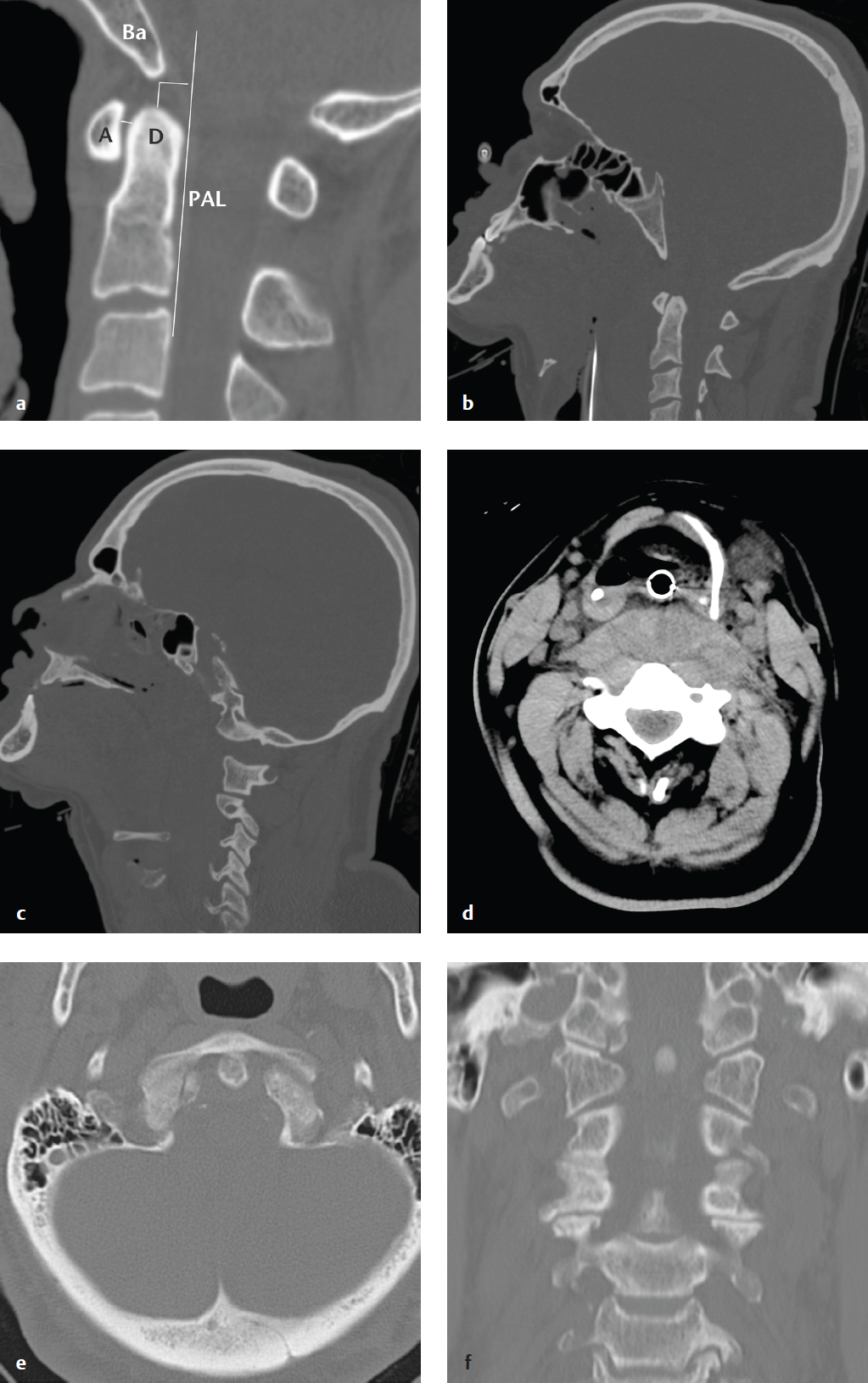
Atlantoaxial Rotatory Subluxation
Atlantoaxial rotatory subluxation is a consequence of motor vehicle accidents and sports-related injuries in older adolescents and adults but may be nontraumatic in children. In trauma, disruption of the alar ligament, atlantoaxial joint capsule, and transverse ligament permits hyperrotation of C1 relative to C2 with widening of the atlantodental interval. Patients present with their head tilted to one side and rotated toward the opposite side.
AP or odontoid radiographs will show asymmetry of the lateral atlantodental intervals. CT is diagnostic and demonstrates rotation of the C1 ring with respect to the C2 lateral masses. If not clinically obvious, dynamic imaging with scanning before and after voluntary head turning can establish whether or not subluxation is fixed.
Fixed subluxation can be reduced by cervical traction followed by active range of motion (ROM) exercises. Rarely, C1–C2 fusion can be considered for persistently fixed and painful rotation ( Fig. 4.2 ).
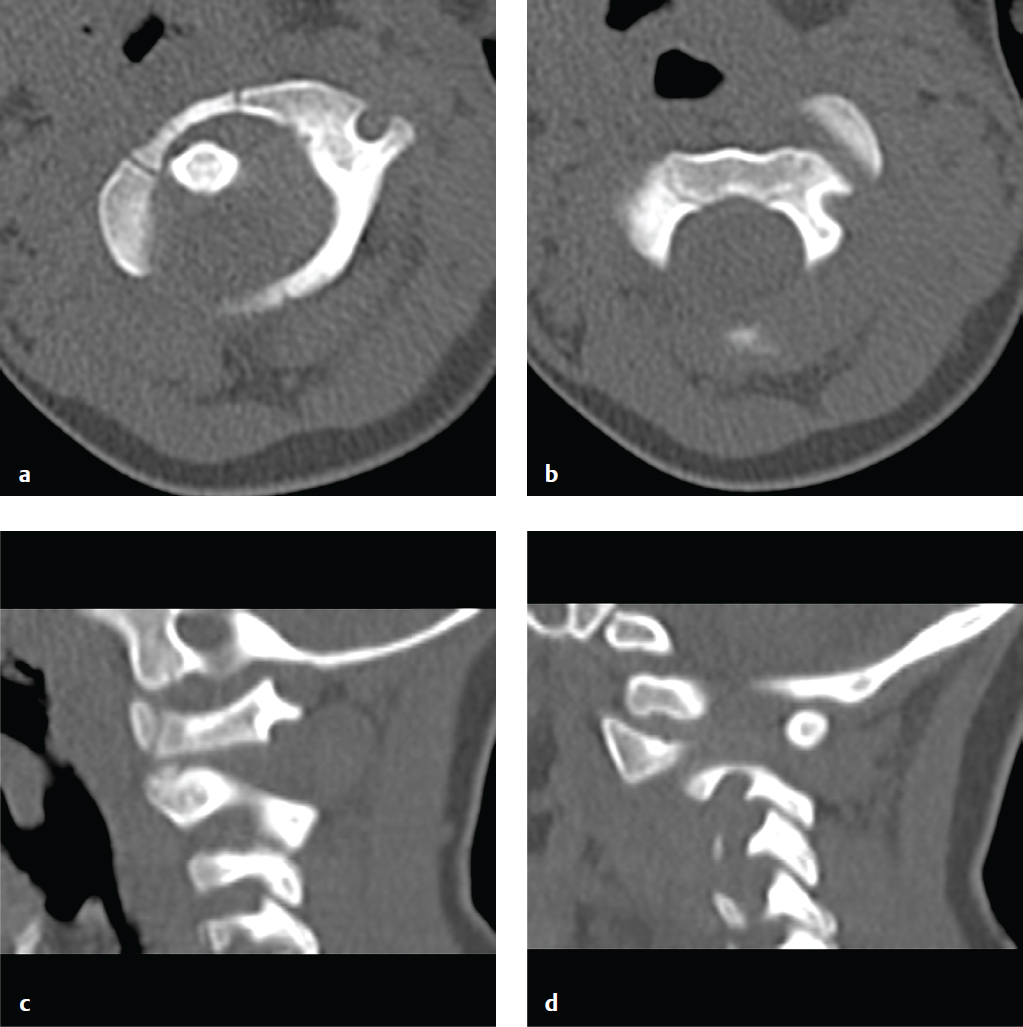
C1 Burst (Jefferson) Fracture
Axial forces transmitted through the occipital condyles to the C1 lateral masses result in multipart fractures of the C1 ring with radial displacement of the fragments away from the C2 odontoid. A common injury mechanism is diving headfirst into shallow water. Because the fracture widens rather than narrows the spinal canal, patients often present with pain but without neurologic dysfunction. Associated spinal cord injury is usually absent unless there is a retropulsed fragment. Stability of this fracture depends on whether or not the transverse atlantal ligament is intact.
The “classical” Jefferson fracture is a four-part fracture with bilateral anterior and posterior arch fractures. Two- and three-part fractures are common variants reflecting eccentric loading and usually involve one lateral mass and the adjacent occipital condyle.
Coronal CT reformations will show displacement of the C1 lateral masses away from the odontoid. If the combined lateral atlantodental intervals are > 7 mm, transverse ligament rupture is possible. Sagittal CT reconstructions will show prevertebral soft tissue swelling as well as a widened atlantodental interval. The normal atlanto-dental interval is ≤ 3 mm in adults and ≤ 5 mm in children. An atlantodental interval > 6 mm indicates definite transverse ligament disruption. Associated cervical spine fractures, especially of C2, should be excluded.
Multidetector CT more precisely delineates the C1 ring fractures, their extent of displacement, and any other synchronous fractures of the cervical spine. Minimally displaced fractures may be treated conservatively with hard collar immobilization. If the transverse atlantal ligament is disrupted, the injury will likely require halo placement or C1–C2 surgical fixation ( Fig. 4.3 ).
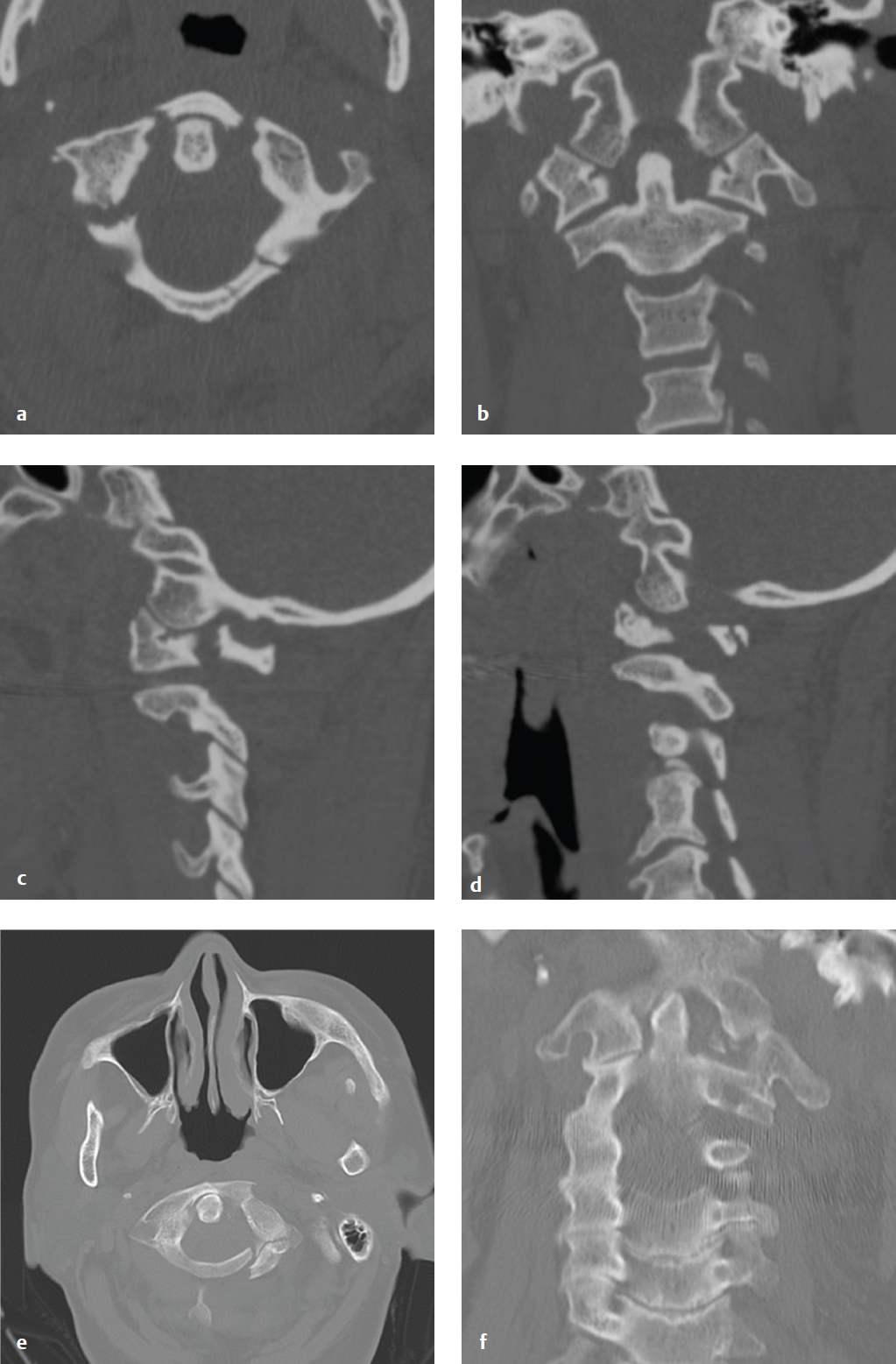
Odontoid (Dens) Fracture
C2 fractures are common and are frequently seen in elderly patients who fall from standing or sitting. Hyperflexion, hyper-extension, and lateral flexion mechanisms can all result in fractures that separate the odontoid from the vertebral body.
Type I fractures are avulsions of the odontoid tip at the attachment of the alar ligaments. These are rare but sometimes seen in patients with atlantooccipital dislocation. Type II fractures traverse the odontoid base. Because the dens is mainly composed of dense cortical bone, they are prone to non-union, and patients often require operative repair. Type III fractures separate a fragment that includes both the dens and a portion of the C2 body. Thanks to the larger area of cancellous bone-to-bone contact, type III fractures tend to heal with immobilization and do not usually require surgical fixation. Types II and III are also referred to as “high” and “low” dens fractures, respectively ( Fig. 4.4 ).
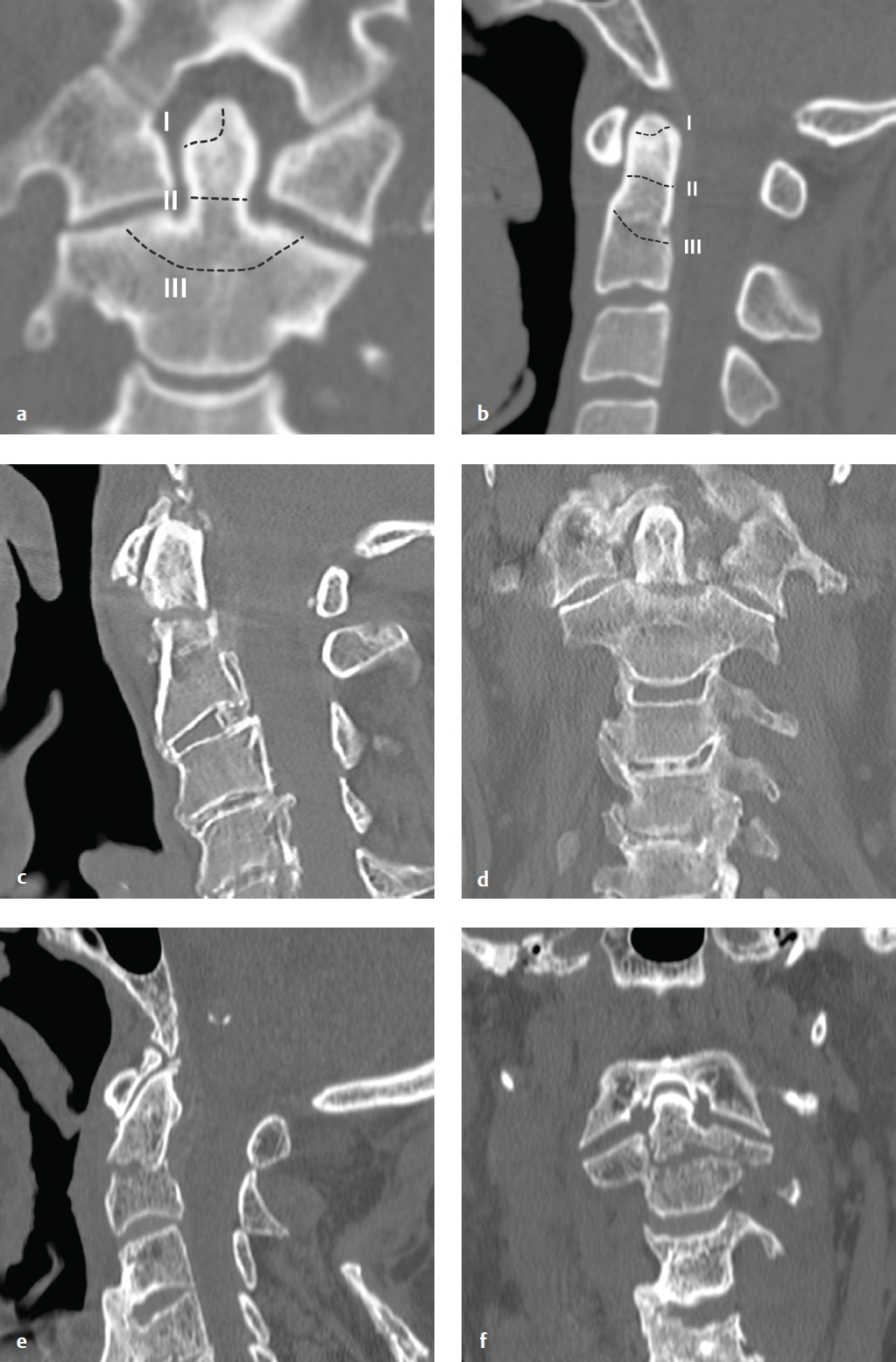
Traumatic C2 Spondylolisthesis
Traumatic C2 spondylolisthesis results from the combined effects of axial loading and either hyperextension or hyperflexion. Both C2 pedicles fracture with varying degrees of angulation and anterolisthesis of C2 on C3. Historically referred to as a “hanged man′s” fracture or “hangman” fracture because of its similarity to the injury caused by judicial hangings, traumatic C2 spondylolisthesis most frequently results from high-speed motor vehicle accidents. Neurologic sequelae are uncommon. Because the fracture tends to widen, rather than narrow, the spinal canal, the spinal cord and nerve roots are not usually damaged. The “atypical” hangman fracture is a variant in which the coronal fracture traverses the posterior C2 body, rather than the pedicles.
CT is necessary for accurate evaluation of displacement and angulation, which impact classification and treatment decisions, as well as identification of vertebral foraminal fractures and potential vascular injury. As in all cervical spine fractures, CTA should be considered to exclude vertebral or carotid artery injury.
Fractures that do not show disk widening, angulation, or subluxation are considered stable (Type I) ( Fig. 4.5 ).
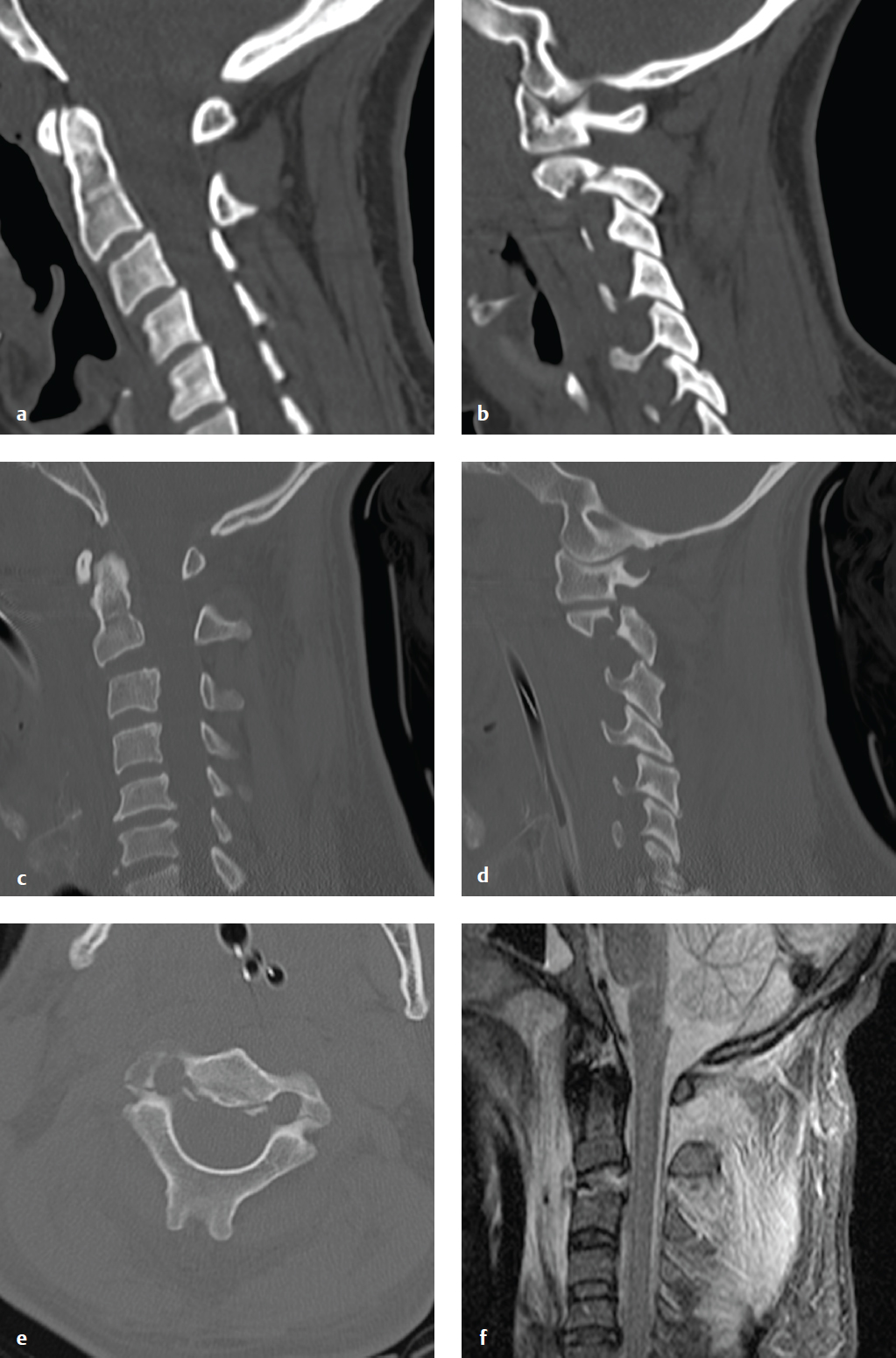
Effendi/Levine and Edwards Classification
Type I: Bilateral pedicle fractures with intact disk and ligaments. No angulation or translation
Type II: C2/3 disk disruption with angulation but no translation
Type III: C2/3 disk disruption with angulation, translation, and facet subluxation or dislocation
Type I fractures are stable, types II and III are unstable ( Fig. 4.6 ).
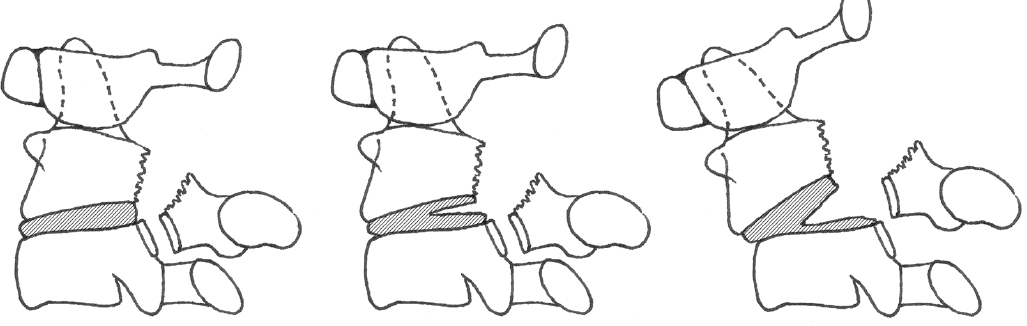
Cervical Hyperflexion Injury 1
Cervical spine hyperflexion injuries result from whiplash mechanisms in which abrupt extension is followed by flexion or from axial loading forces acting upon a flexed neck. The latter are seen in head-on motor vehicle collisions and diving accidents. Hyperflexion injuries range, with increasing severity, from the stable hyperflexion sprain, in which only the posterior ligamentous complex is disrupted, to anterior vertebral subluxation, bilateral facet dislocation, and flexion “teardrop” or burst fractures.
Hyperflexion sprains may only show subtle spinous process distraction on lateral radiographs or sagittal CT reformations. If the injury is limited to the interspinous and nuchal ligaments, it is considered stable. Any degree of anterior vertebral subluxation, facet subluxation, or facet dislocation, however, indicates instability. MRI and/or delayed flexion and extension radiographs (after muscle spasm related to the acute injury has resolved) can be used to assess ligamentous injury and stability better.
The clay shoveler fracture is a horizontal fracture of a lower cervical spinous process originally described in workers who would abruptly contract their cervical muscles and flex the neck while shoveling heavy clay. The fracture may also result from direct impact and other complex mechanisms. Clay shoveler fractures are stable, as they do not involve the vertebral ring and are often incidentally discovered years after a forgotten injury.
Bilateral interfacet dislocation (BID) is a hyperflexion injury characterized by disruption of the anterior, middle, and posterior spinal ligaments. It is uncommon, extremely unstable, and associated with a high risk of spinal cord damage. On lateral cervical spine radiograph or sagittal CT reformation, the inferior articulating facet of the upper vertebra is displaced and locked anterior to the superior articulating facet of the lower vertebra. The upper vertebral body is anteriorly displaced by up to 50% relative to the lower one. Disk space and interspinous widening reflect the ligamentous nature of this injury. Small facet fractures may be seen ( Fig. 4.7 ).
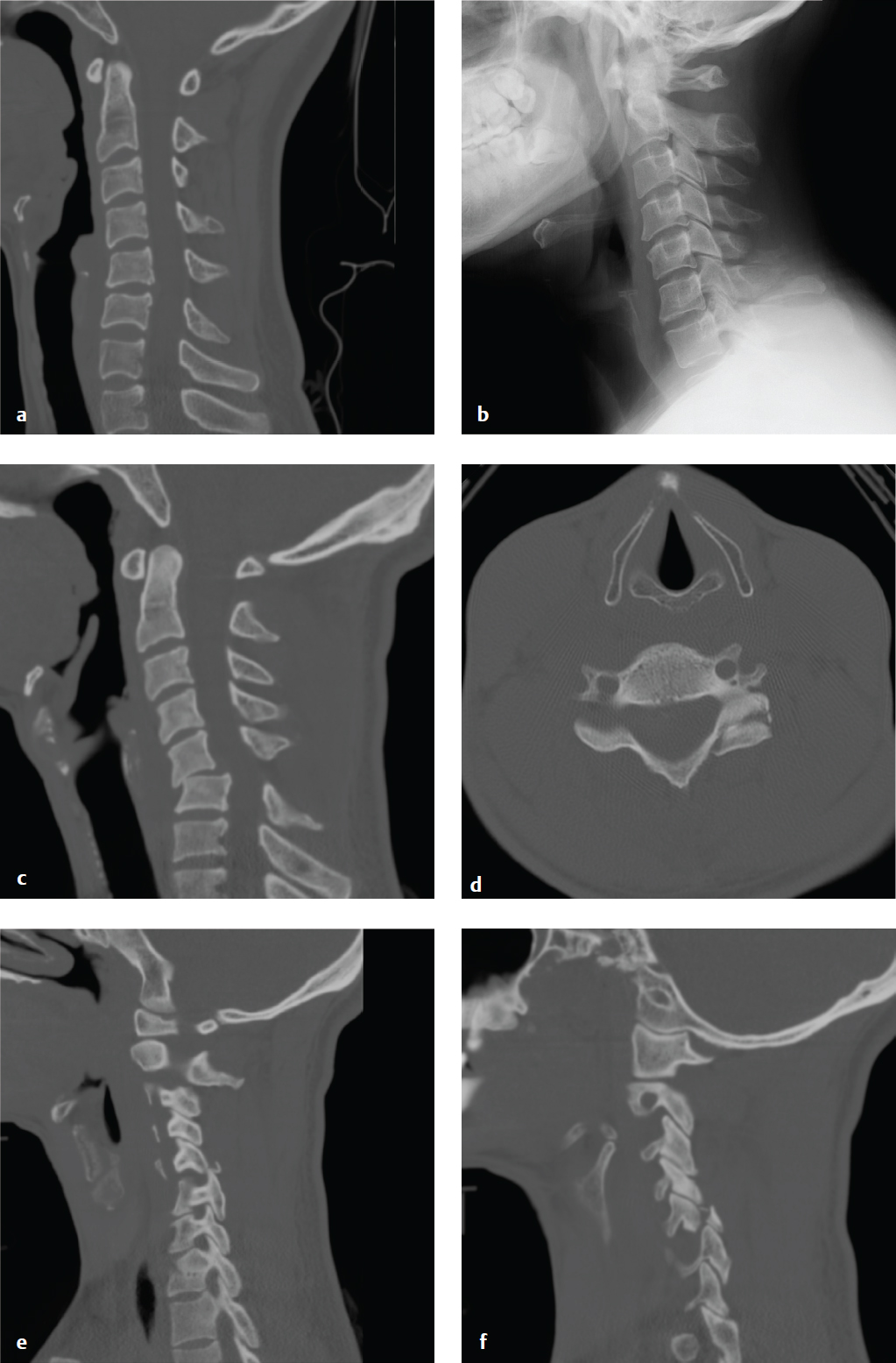
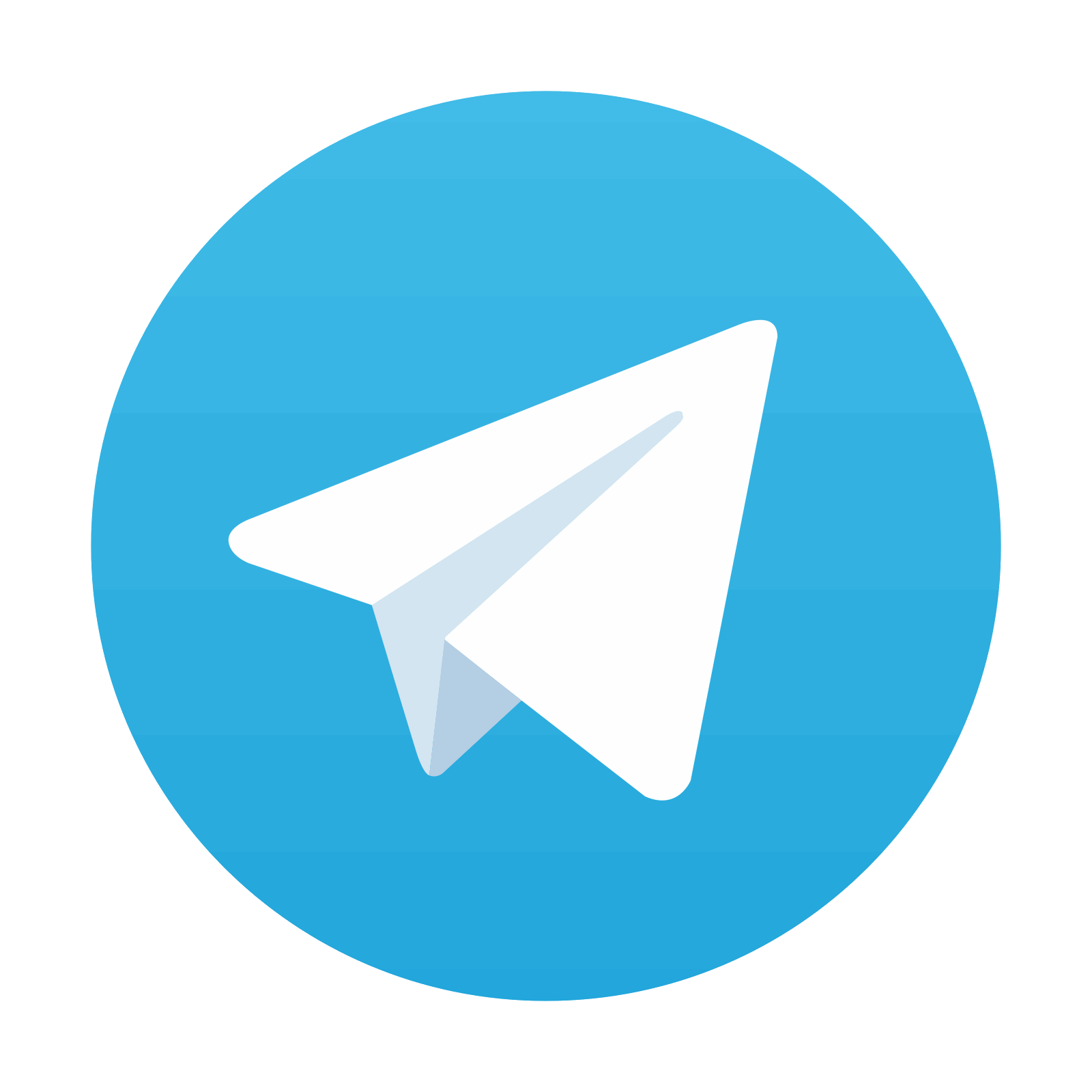
Stay updated, free articles. Join our Telegram channel

Full access? Get Clinical Tree
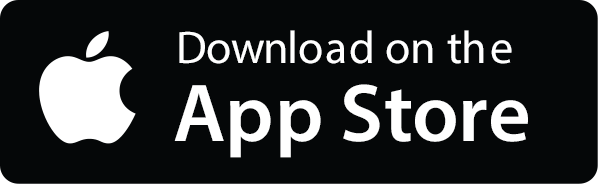
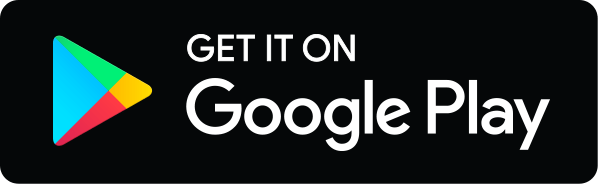