5 Vascular Abnormalities
Table 5.1 Congenital and developmental vascular anomalies/variants |
Table 5.2 Acquired vascular disease |
Introduction
Arterial Anatomy
The intracranial arterial system is divided into the anterior and posterior circulations. The anterior circulation includes the internal carotid artery and its branches as well the anterior and middle cerebral arteries and anterior and posterior communicating arteries. The posterior circulation includes the vertebral arteries, basilar artery, and posterior cerebral arteries ( Fig. 5.1 ).
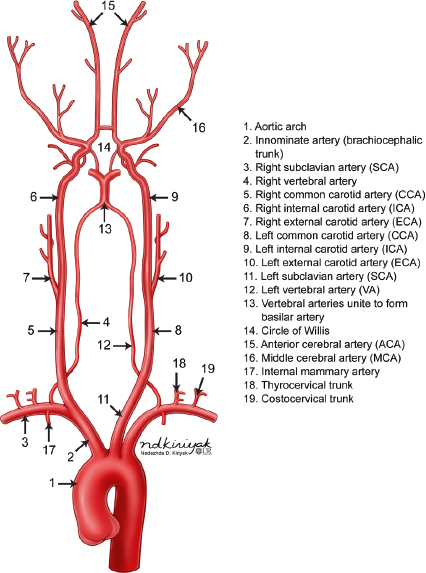
The internal carotid artery (ICA) is divided into seven segments corresponding to their embryonic precursor arteries ( Fig. 5.2 and Fig. 5.3 ). The first ICA segment extends from the bifurcation of the common carotid artery in the neck to the inferior level of the skull base. The second ICA segment is located within the petrous carotid canal of the temporal bone and has two small branches, the vidian artery (artery of the pterygoid canal), which anastomoses with branches of the external carotid artery (ECA), and the caroticotympanic artery to the middle ear. The third ICA segment is a short portion superior to the foramen lacerum that extends from the petrous apex to the cavernous sinus. The fourth ICA segment is located within the cavernous sinus and has two major branches (the meningohypophyseal trunk, which supplies the pituitary gland; clival dura; and tentorium via basal and marginal tentorial branch arteries, inferior hypophyseal artery, and trigeminal ganglion artery; and the inferolateral trunk, which supplies the cranial nerves and dura of the cavernous sinuses via cavernous sinus and meningeal branch arteries), which have anastomoses with branches of the ECA. The fifth ICA segment courses within the anterior portion of the cavernous sinus until it exits superiorly into the cranial cavity. The ophthalmic artery may occasionally arise from the fifth segment. The sixth ICA segment is the first segment in the intracranial subarachnoid space and has two branches (the ophthalmic artery and the superior hypophyseal artery, which supplies the adenohypophysis, pituitary stalk, and optic chiasm). The seventh ICA segment extends superiorly to the bifurcation of the ICA into the anterior cerebral artery (ACA) and middle cerebral artery (MCA) and includes the posterior communicating artery (PCOM) as a branch.
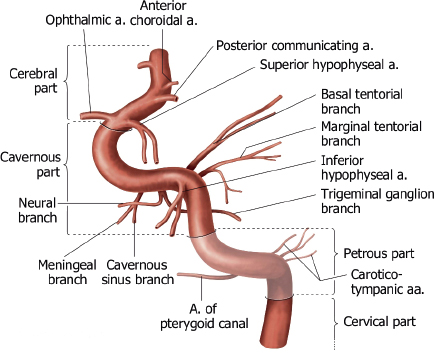
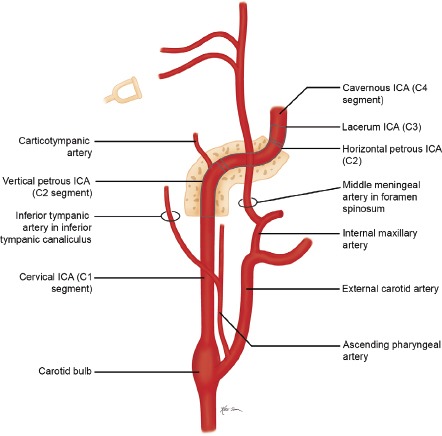
The ACA has three segments ( Fig. 5.4 and Fig. 5.5 ). The A1 segment extends medially from the ICA to the midline and the origin of the anterior communicating artery (ACOM), which connects to the contralateral ACA. The A1 segment has branch arteries, such as the medial lenticulostriate arteries, that provide blood supply to the basal ganglia. The A2 segment extends superiorly within the interhemispheric fissure from the ACOM to the level of the rostrum of the corpus callosum. Branch arteries from the A2 segment include the orbitofrontal and frontopolar arteries, which supply the inferomedial portions of the frontal lobes. The recurrent artery of Heubner can also arise from the proximal portion of A2 providing blood to the basal ganglia in addition to the lenticulostriate arteries from the A1 segment. In some cases, the recurrent artery of Heubner may arise from the A1 segment or anterior communicating artery. The A3 segment extends around the corpus callosum and divides into the pericallosal and callosomarginal arteries, which course posteriorly over the corpus callosum and cingulate gyrus, respectively. The A2 and A3 segments provide blood to the medial portions of the frontal and parietal lobes, corpus callosum, and anterior limbs of the internal capsules.
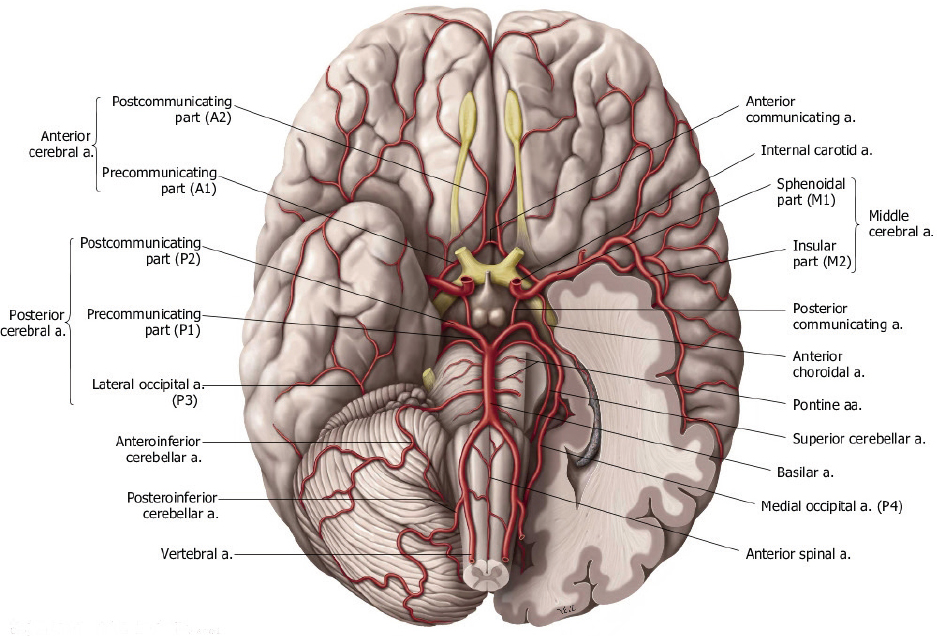
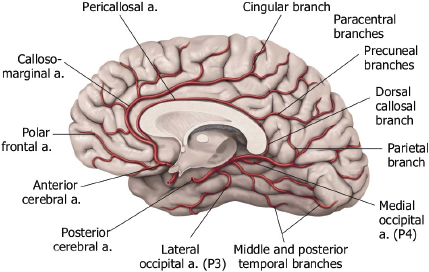
The MCA has four segments ( Fig. 5.6, Fig. 5.7 , and Fig. 5.8 ). The M1 (horizontal) segment extends laterally from the ICA to the sylvian fissure and has branch arteries, including the lateral lenticulostriate arteries, which supply the external capsule, caudate nucleus, and putamen, and the anterior temporal artery, which supplies the anterior portions of the temporal lobes. At the level of the sylvian fissure, the M1 segment bifurcates into the M2 (insular) segments, which extend upward and posteriorly along the insula. Branches from the M2 segments extend laterally along the overhanging or opercular portions of the cerebral hemispheres (M3 segments), providing blood to these locations. Continuation of the M3 segments after they exit the sylvian fissure are the M4 (cortical) segments, which supply the lateral portions of the cerebral hemispheres.
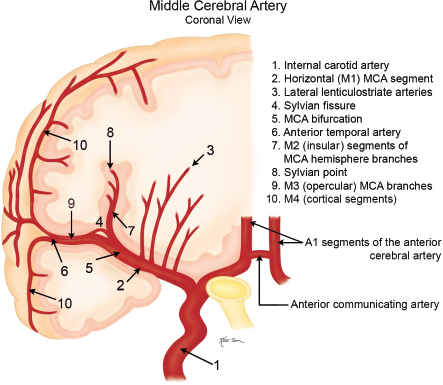
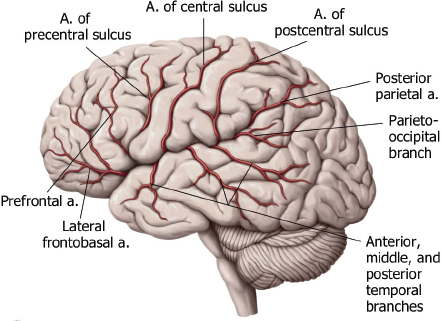
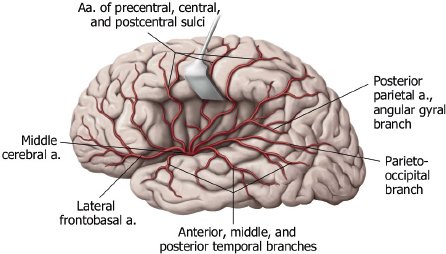
The upper terminal branches of the basilar artery are the posterior cerebral arteries (PCAs), which supply blood to the posteroinferior portions of the parietal lobes, occipital lobes, and thalami ( Fig. 5.9 and Fig. 5.10 ). The PCAs have four segments. The P1 segment extends laterally from the basilar artery to the level of the posterior communicating artery (PCOM). Branches from the P1 segment include the posterior thalamoperforating arteries, which supply the midbain and posterior portions of the thalami. The P2 segment extends posterolaterally from the P1 segment– PCOM junction around the midbrain above the tentorium. Arterial branches from the P2 segment include the anterior and posterior temporal arteries, which provide blood to the inferior portions of the temporal lobes not supplied by the anterior temporal artery from the M1 segment of the MCA. Other branches from the P2 segment include the medial and lateral posterior choroidal arteries, which supply the choroid plexus of the third and lateral ventricles, respectively; the thalamogeniculate arteries, which supply the posterior thalamus; and the peduncular perforating arteries, which supply the midbrain. The P3 segment is the portion of the PCA located in the quadrigeminal cistern posterior to the midbrain prior to its entry into the calcarine fissure. The P4 segment of the PCA is located within the calcarine fissure and its branches include the parieto-occipital artery, calcarine artery, lateral occipital artery, and posterior splenial arteries, which supply the occipital lobe, posterior medial portions of the temporal lobes, and posterior corpus callosum.
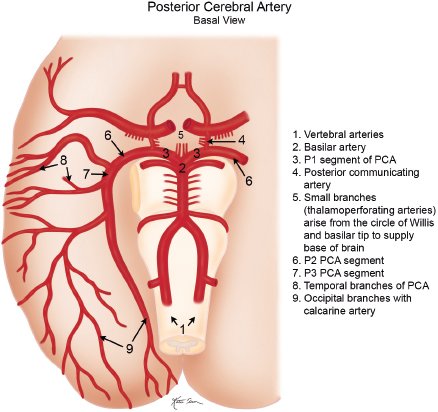
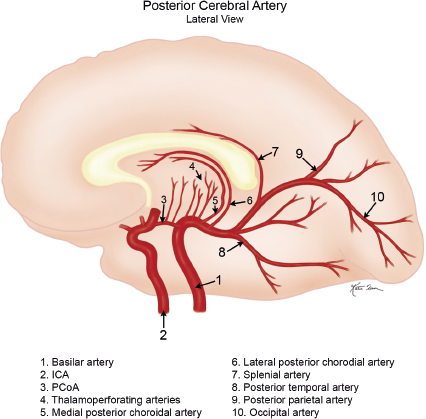
Single vertebral arteries (VAs) arise from each subclavian artery and extend superiorly in the neck to eventually fuse intracranially to form the basilar artery anterior to the brainstem near the pontomedullary junction ( Fig. 5.1 ). Each vertebral artery is divided into four segments. The V1 segment of the VA extends from its origin from the subclavian artery to its entry into the C6 foramen transversarium. The V1 segment supplies the lower portion of the cervical spinal cord and paraspinal musculature. The V2 segment extends upward through the C6 to C1 foramina tranversaria. The anterior meningeal artery is a branch from the V2 segment. The V3 segment is the portion of the VA from its exit from the C1 transverse foramen to the level of the outer dural margin at the foramen magnum. The posterior meningeal artery is a branch from the V3 segment. The V4 segment is the intradural portion of the VA from which the posterior inferior cerebellar artery arises. Other branches from the V4 segment include medullary perforating arteries and anterior and posterior spinal arteries. Both V4 segments fuse to form the basilar artery. Branches from the basilar artery include the anterior inferior cerebellar artery, superior cerebellar arteries, and basilar perforating arteries ( Fig. 5.11 and Fig. 5.12 ). The uppermost portion of the basilar artery terminates with a bifurcation into the right and left posterior cerebral arteries. The upper vertebral arteries and basilar artery provide blood supply to the upper spinal cord, brainstem, and cerebellum.
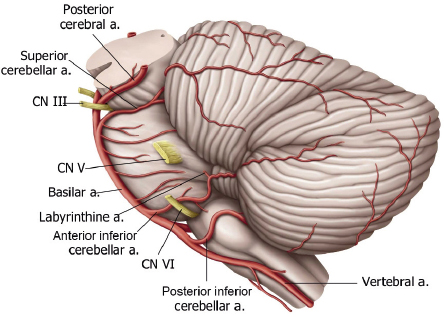
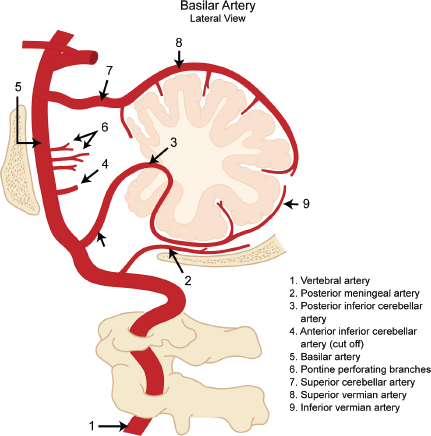
The circle of Willis is an important intracranial anastomotic arterial ring around the lower brain interconnecting the anterior and posterior circulations and includes the upper portions of both ICAs, the A1 segments of the ACAs, the anterior communicating artery (ACOM), the basilar artery, posterior communicating arteries (PCOMs), and P1 segments of both posterior cerebral arteries (PCA).
The external carotid artery (ECA) arises from the bifurcation of the cervical portion of the common carotid artery. The ECA has eight major branches ( Fig. 5.13 ). The superior thyroid artery extends inferiorly to supply the larynx and thyroid gland. The ascending pharyngeal artery extends superiorly to supply the nasopharynx, oropharynx, dura, middle ear, and cranial nerves IX, X, and XI. The lingual artery supplies the oral cavity, tongue, and submandibular gland. The facial artery supplies the face, cheek, lips, and palate. The occipital artery supplies the meninges of the posterior cranial fossa as well as the upper cervical paraspinal muscles and scalp. The posterior auricular artery supplies the scalp and outer ear. The superficial temporal artery supplies the scalp. The maxillary artery supplies the deep soft tissues of the face and nose. A branch from the maxillary artery extends superiorly through the foramen spinosum as the middle meningeal artery to supply the intracranial meninges. Anastomoses between ECA branches (except for the superior thyroid and lingual arteries) and intracranial branches of the ICA and/or vertebral arteries can occur.
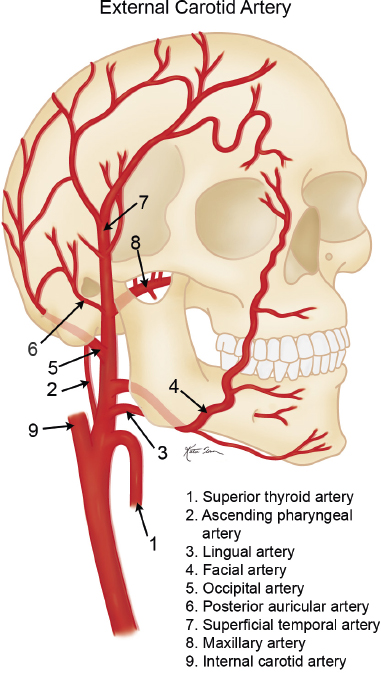
Intracranial Venous Anatomy
The intracranial venous system consists of a superficial system that drains blood from the cerebral cortex and superficial white matter into cortical veins and eventually into the dural venous sinuses, and a deep venous system that drains blood from the deep white matter and basal ganglia ( Fig. 5.14 , Fig. 5.15 , and Fig. 5.16 ). The deep venous system includes the paired internal cerebral veins in the roof of the third ventricle, the basal vein of Rosenthal, the vein of Galen, and the straight venous sinus. Also included in the deep venous system are transcerebral veins. The internal cerebral veins represent the confluence of septal, subependymal, ventricular, anterior caudate, thalomostriate, and choroidal veins at the foramen of Monro. The basal veins of Rosenthal are located at the medial aspects of the temporal lobes and drain blood from the adjacent temporal lobes, insula, and cerebral peduncles. These veins have anastomoses with the middle cerebral veins and petrosal veins. The basal veins course posteriorly around the cerebral peduncles, where they unite with internal cerebral veins to form the single midline great vein of Galen located below the splenium of the corpus callosum. The vein of Galen connects with the inferior sagittal sinus to drain venous blood into the straight venous sinus. Transcerebral veins are medullary veins that extend though the cerebral tissue and are not usually visualized on normal MRI or CT examinations.
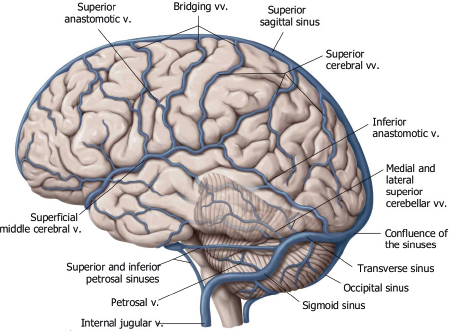
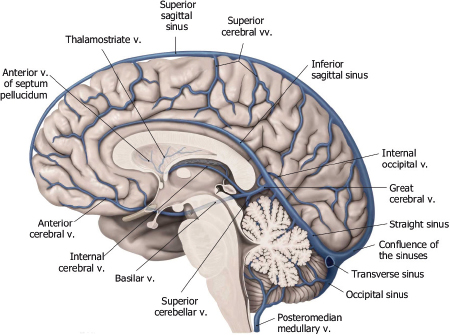
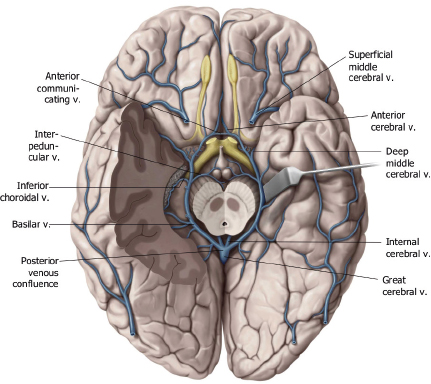
The superficial venous system includes superficial cerebral veins along the surface of the brain that drain blood from the adjacent cerebral cortex and subcortical white matter. The superficial veins can have various configurations, including approximately 12 that drain into the superior sagittal sinus. A prominent superficial anastomotic vein of Trolard can be observed that connects to the superior sagittal sinus. A prominent inferior anastomotic superficial vein of Labbé can also be seen that connects the transverse venous sinus. The superficial middle cerebral vein is another prominent vein that courses anteriorly in the sylvian fissure and drains blood from the adjacent lateral brain into the cavernous sinuses and/or pterygoid venous plexus.
Venous Sinuses
Veins from the superficial and deep venous systems drain into the dural venous sinuses ( Fig. 5.17 ). The dural venous sinuses are endothelial channels located between the periosteal (outer) and meningeal (inner) layers of the dura. The dural venous sinuses can be subdivided into two groups: posterosuperior and anteroinferior. The posterosuperior group includes the superior and inferior sagittal sinuses, straight venous sinus, torcular herophili, transverse and sigmoid sinuses, and jugular bulbs. Arachnoid granulations can occur in all of the dural venous sinuses, although they are most frequently present in the superior sagittal and transverse venous sinuses.
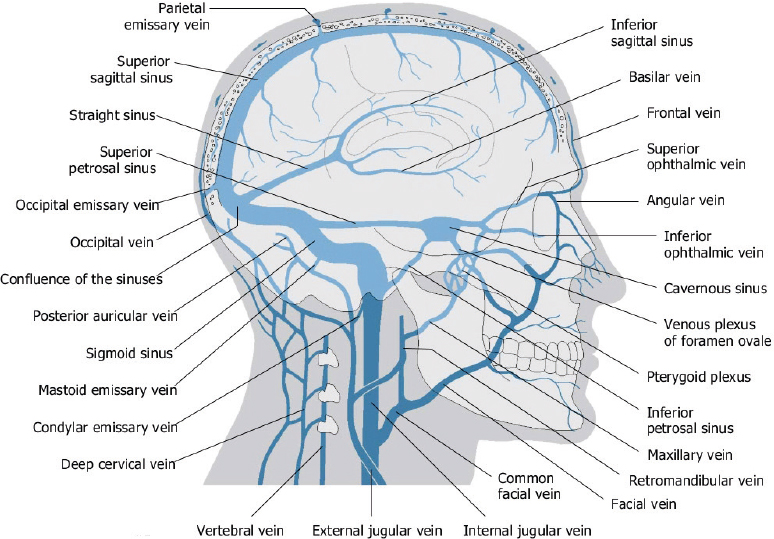
The anteroinferior group of dural venous sinuses includes the cavernous sinus, superior and inferior petrosal sinuses, clival venous sinus, and sphenoparietal sinus. Blood from the cavernous sinuses drain into pterygoid venous plexuses via the foramen ovale or into the superior and/or inferior petrosal sinuses. Blood from the superior petrosal sinus drains into the sigmoid sinus, whereas blood from the inferior petrosal sinus drains into the jugular bulb. The clival venous plexus connects the cavernous sinus with the petrosal sinuses. The sphenoparietal sinus at the anterior portion of the middle cranial fossa connects the superficial veins adjacent to the temporal lobes to the inferior petrosal sinus ( Fig. 5.17 ).
The veins in the posterior cranial fossa can drain upward into the vein of Galen (superior vermian vein, precentral cerebellar vein, and anterior pontomesencephalic vein), anteriorly into the petrosal sinuses (petrosal veins), or posterolaterally into the transverse or sigmoid venous sinuses (inferior vermian veins) ( Fig. 5.18 ).
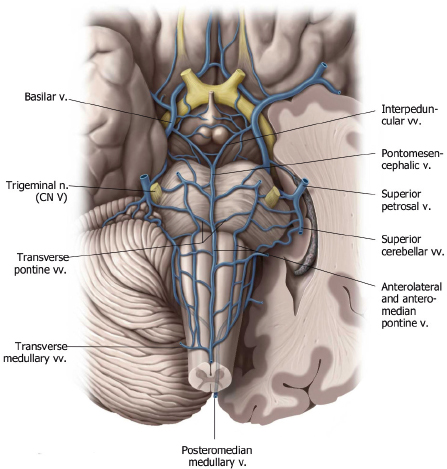
Cerebral Arterial and Venous Development
Arterial Development
There are six branchial arches during embryonic development. Derivatives of the third branchial arches form the internal carotid arteries. A portion of the left fourth branchial arch forms most the aortic arch, and the right fourth branchial arch forms the proximal segment of the right subclavian artery. Derivatives of the first arch form the vidian artery. Derivatives of the second arch form the pharyngeal artery, external carotid artery, stapedial artery, and caroticotympanic artery.
At 4 weeks of gestation, primitive, paired internal carotid arteries connected to the dorsal aorta and third aortic arch provide blood to the developing vesicles of the forebrain, midbrain, and hindbrain. The mature pattern of the cerebral arterial system develops by 8 weeks of gestation.
At 4 weeks of gestation, the developing hindbrain is supplied by paired longitudinal arteries that have transient anastomoses with the internal carotid arteries. These paired arteries progressively fuse in the midline to form the basilar artery around 5 weeks of gestation. Lack of normal fusion of the longitudinal arteries can result in arterial fenestrations. Lack of normal involution of some of the anastomoses between the internal carotid arteries and longitudinal arteries can result in anomalous connections between the internal carotid arteries and basilar artery, such as a persistent trigeminal artery ( Fig. 5.19 ).
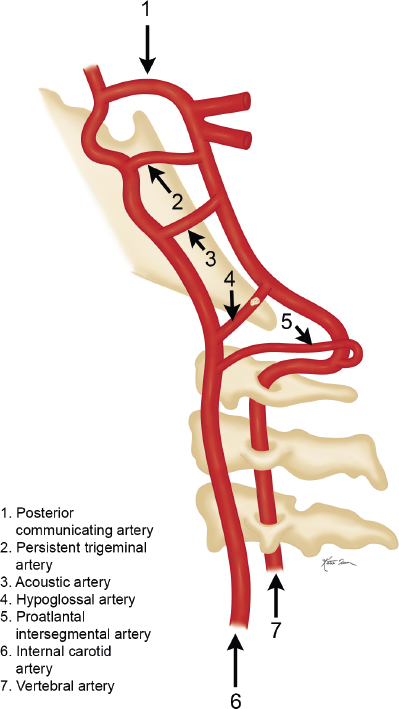
Venous Development
At the fifth week of gestation, three venous plexuses form around the posterolateral aspects of the developing brain. Progressive growth and connections between these plexuses result in eventual formation of the major dural venous sinuses over the next 6 months.
Development of cortical veins occurs after normal involution of early fetal transcerebral veins, and the primitive pial venous plexus develops by 11 weeks of gestation.
CT Angiography and CT Perfusion
CT angiography (CTA) is a powerful imaging modality for evaluating normal and abnormal blood vessels. CTA has proven to be clinically useful in the evaluation of intracranial arteries, veins, and dural venous sinuses. Pathologic processes involving intracranial blood vessels, such as aneurysms, arteriovenous malformations, arterial occlusions, and dural venous sinus thrombosis, can be seen with CTA.
CT perfusion is a relatively new technique using dynamic intravenous infusion of contrast to measure cerebral blood flow (CBF), cerebral blood volume (CBV), and mean transit time of contrast enhancement (MTT) in selected volumes of interest in the brain. CT perfusion has major clinical application in the evaluation of cerebral infarcts and adjacent zones of decreased perfusion (penumbra and oligemic areas) at risk for progression to infarction. Maintenance of CBF is critical for neuronal function. With arterial occlusion, loss of normal neuronal electrical activity occurs within seconds after arterial occlusion. Cellular death is dependent on the duration and magnitude of ischemia, metabolic vulnerability of specific anatomic sites, and the oxygen content of blood. Normal CBF ranges from 50 to 60 mL/100 g/min. When CBF is reduced to 15 to 20 mL/100 g/min for several hours (mild–moderate hypoxia), spontaneous and evoked neuronal electrical activity decreases significantly secondary to ischemia, although it can be reversed by reperfusion with CBF above 50 mL/100 g/min. With severe hypoxia/anoxia resulting from CBF below 10 mL/100 g/min, cellular membrane depolarization and ischemia leading to brain infarction may occur within several minutes.
When thrombotic or embolic arterial occlusions occur, CBF in the involved brain tissue is usually heterogeneous, with a central core showing the greatest reductions in CBF that cause irreversible cell damage and infarction, and a surrounding zone (referred to as the salvageable penumbra) that may have moderate reduction in CBF, resulting in ischemia that may be reversible with reperfusion. The penumbra typically shows loss of neuronal electrical activity without immediate anoxic depolarization, as well as loss of autoregulation. If reperfusion does not occur, the penumbra will progress to infarction. An oligemic zone of mildly reduced CBF may also be seen surrounding the penumbra, and this zone is less vulnerable to infarction than the penumbra. Thrombolytic medication can be useful and beneficial when it results in timely reperfusion to the penumbra and oligemic zone. Estimating the sizes of the penumbra and oligemic zone can be done in the acute setting with dynamic contrast-enhanced CT. CT perfusion, utilizing iodinated contrast delivered as an intravenous bolus, can use the linear relationship between contrast concentration and attenuation to directly calculate and quantify CBF, CBV, and MTT for sites of ischemia and infarction in the brain prior to thrombolytic treatment.
Magnetic Resonance Angiography
Magnetic resonance imaging (MRI) is a powerful modality for evaluating normal and abnormal blood vessels. The appearance of blood vessels on MRI depends on various factors, such as the type of MRI pulse sequence, pulsatility and range of velocities in the vessels of interest, and size, shape, and orientation of the vessels relative to the image plane. Useful anatomic information about blood vessels can be gained by using spin echo pulse sequences, which can display patent vessels as zones of signal void (blackblood images), or gradient recalled echo (GRE) pulse sequences, which display the moving hydrogen atomic nuclei (protons) in blood as zones of high signal (brightblood images).
The GRE technique is used to generate MR angiograms (MRA). The high signal from flowing blood on GRE images reflects movement patterns and velocities of hydrogen atomic nuclei rather than direct anatomic displays of the blood vessels. The operator of the MRI equipment can choose parameters to optimize the imaging of various arteries and veins. Two main types of GRE techniques are used for MRA. One is based on hydrogen signal amplitude and is referred to as the time-of-flight (TOF) method. The other method is based on the phase differences of the moving protons (hydrogen) in blood compared with stationary tissue and is referred to as phase-contrast (PC) MRA.
In TOF MRA, the GRE pulse sequence is optimized for demonstrating the inflow enhancement (high signal) of moving protons (hydrogen nuclei) in blood relative to the low signal of protons in stationary tissue. Phase-contrast (PC) MRA is a technique that differentiates flowing and stationary protons through the use of bipolar flow-encoding gradients. If the flow velocity is known, the flow sensitivity of the sequence can be selected to emphasize the vessels of interest. PC MRA can be optimized for detecting slow flow in veins and at areas of high-grade arterial stenosis.
The individual GRE images can be acquired in a sequential mode, also referred to as two-dimensional (2D) TOF or PC MRA, or as an entire volume of covered tissue, which is referred to as three-dimensional (3D) TOF or PC MRA. The acquired image data from either of these two methods are post-processed with computer algorithms to generate the MRA images in a display format similar to conventional arteriograms. Two commercially available types of post-processing are the maximum intensity projection (MIP) technique and surface rendering (SR). The former technique is more common, and the MIP MRA images can be displayed in any plane of obliquity on film or as a movie loop. Surface rendering is another post-processing method for MRA that shows 3D relationships by giving the displayed vessels shadowing and perspective. The MRA images are projected in a fashion similar to that used for the MIP method. Surface rendering has been demonstrated to be useful in showing spatial relationships between vessels on a single coronal image, allowing differentiation of adjacent and overlapping vessels.
MRA has proven to be clinically useful in the evaluation of the carotid arteries in the neck, intracranial arteries, intracranial veins, and dural venous sinuses. Disorders like aneurysms, arteriovenous malformations, arterial occlusions, dural venous sinus thrombosis, etc., can be seen with MRA.
References
1. Johnson MH, Thorisson HM, Diluna ML. Vascular anatomy: the head, neck, and skull base. Neurosurg Clin N Am 2009;20(3):239–258 2. Kathuria S, Chen J, Gregg L, Parmar HA, Gandhi D. Congenital arterial and venous anomalies of the brain and skull base. Neuroimaging Clin N Am 2011;21(3):545–562, vii 3. Kathuria S, Gregg L, Chen J, Gandhi D. Normal cerebral arterial development and variations. Semin Ultrasound CT MR 2011;32(3):242–251 4. Raybaud C. Normal and abnormal embryology and development of the intracranial vascular system. Neurosurg Clin N Am 2010;21(3):399–426 5. Scott JN, Farb RI. Imaging and anatomy of the normal intracranial venous system. Neuroimaging Clin N Am 2003;13(1):1–12Table 5.1 Congenital and developmental vascular anomalies/variants
Persistent fetal origin of posterior cerebral artery
Hypoplasia of the A1 segment of the anterior cerebral artery
Persistent trigeminal artery (PTA)
Persistent otic artery
Persistent hypoglossal artery
Proatlantal artery
Duplications of cerebral, carotid, vertebral, or basilar arteries
Azygous anterior cerebral artery
Hemiazygous anterior cerebral artery
Arterial fenestration
Aberrant position of the internal carotid artery
Persistent stapedial artery (PSA)
Unilateral agenesis, aplasia, and hypoplasia of the internal carotid artery
Vein of Galen aneurysm
Sturge-Weber syndrome
Moyamoya disease
ACTA2 mutations with dolichoectasia of the proximal internal carotid arteries and stenosis of the upper internal carotid arteries
Menkes’ syndrome
PHACES syndrome (posterior fossa malformations, facial hemangiomas, arterial anomalies, cardiac anomalies and aortic coarctation, eye abnormalities, and sternal clefts or supraumbilical raphe)
Thoracic outlet syndrome
Venous angioma (developmental venous anomaly)
Dehiscence of the jugular bulb
High position of the jugular bulb
Sinus pericranii
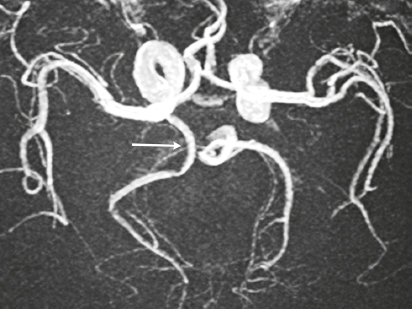
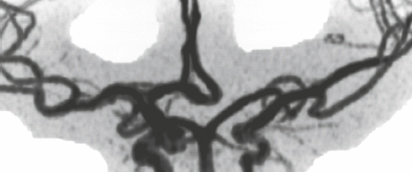
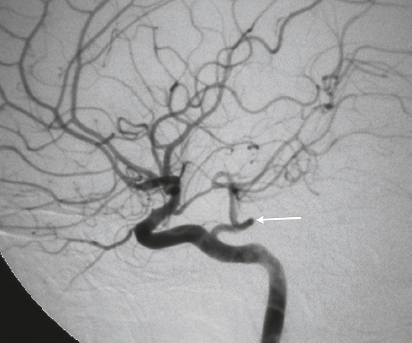
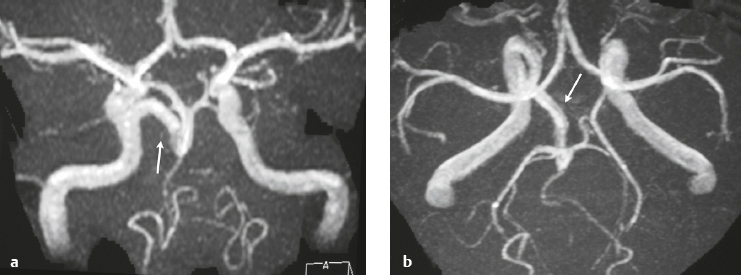
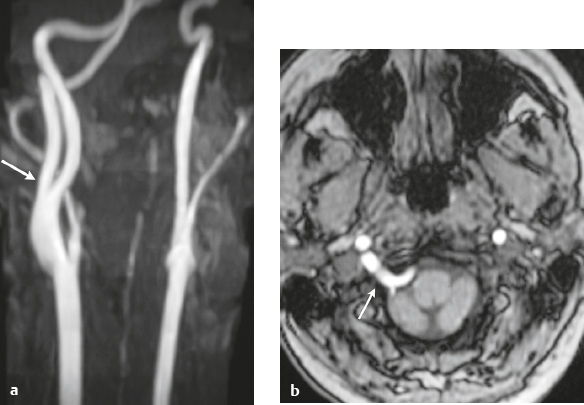
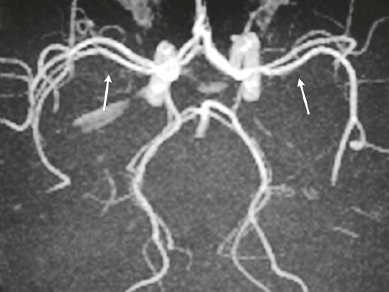
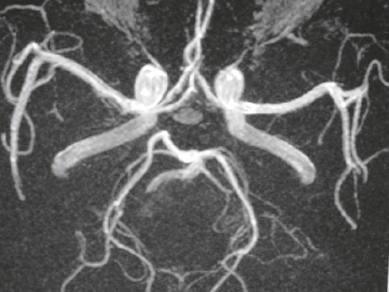
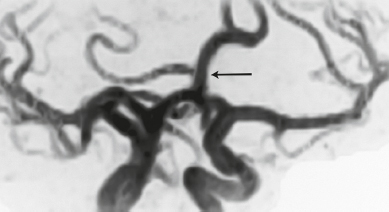
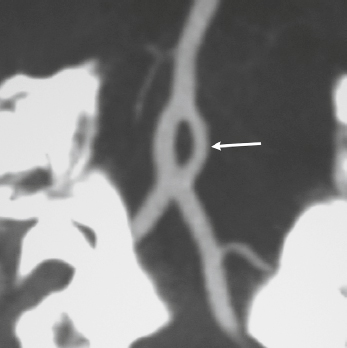
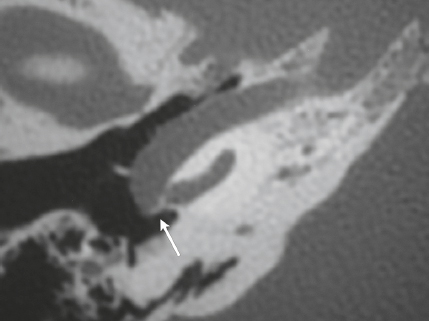
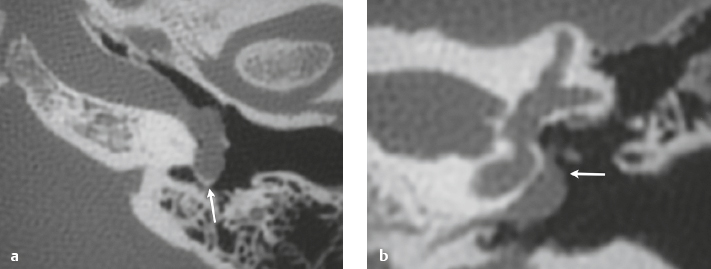
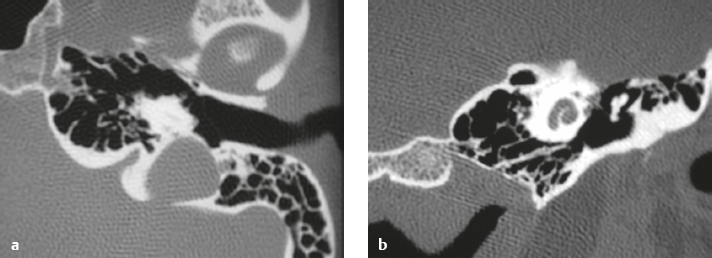
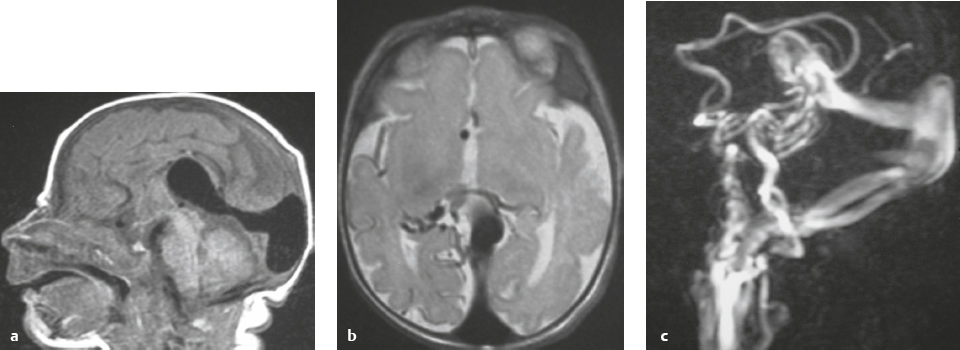
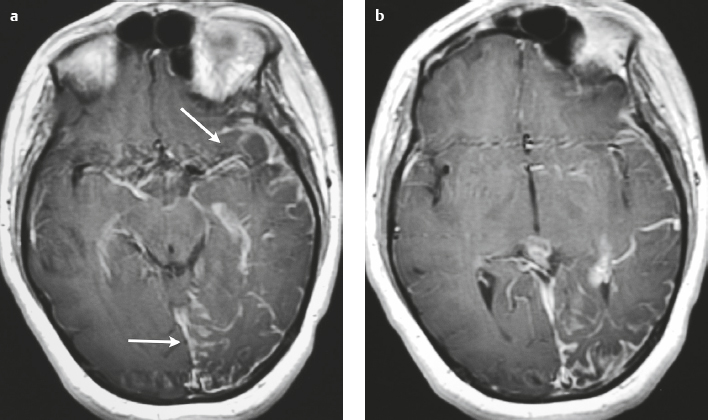
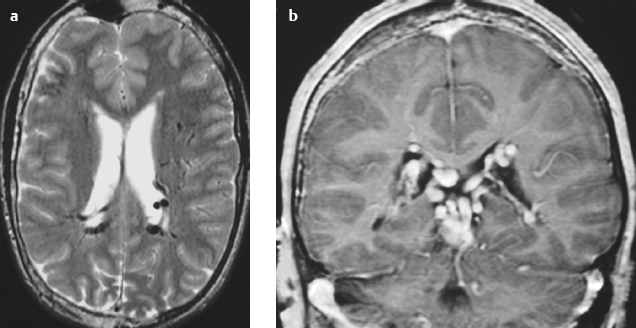
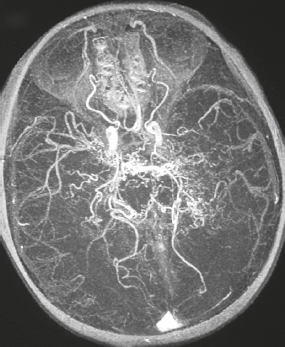
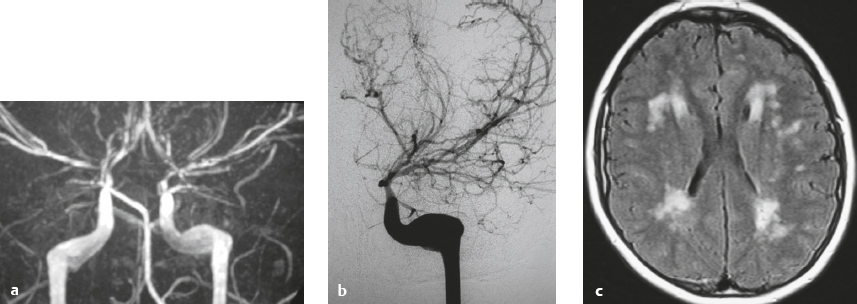
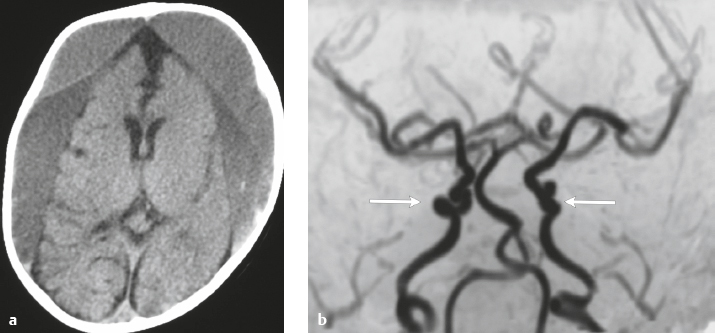
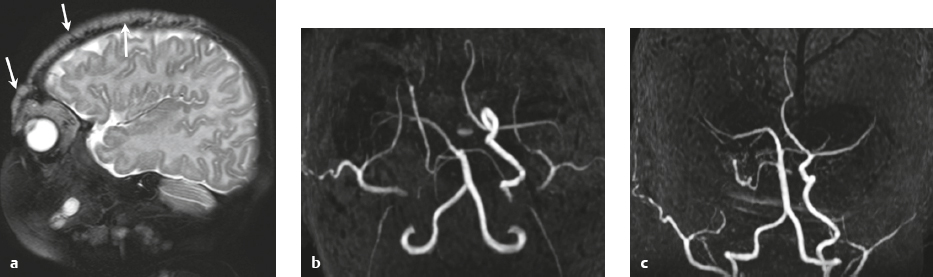
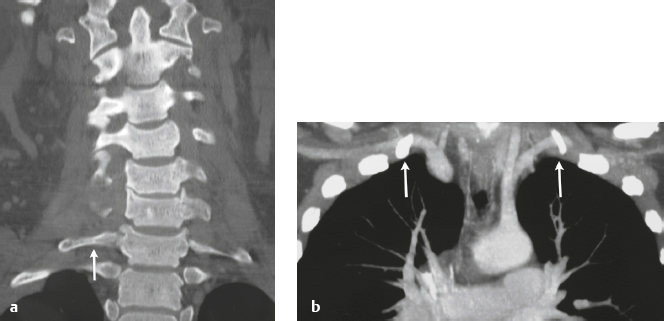
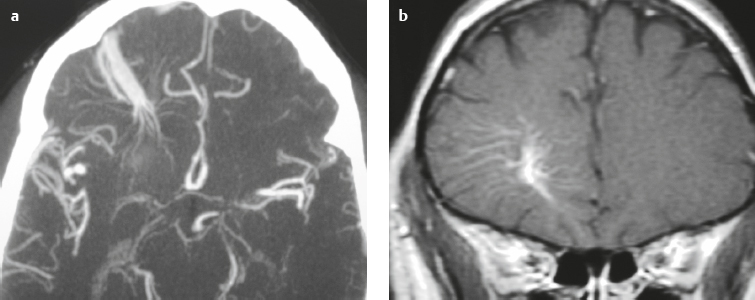
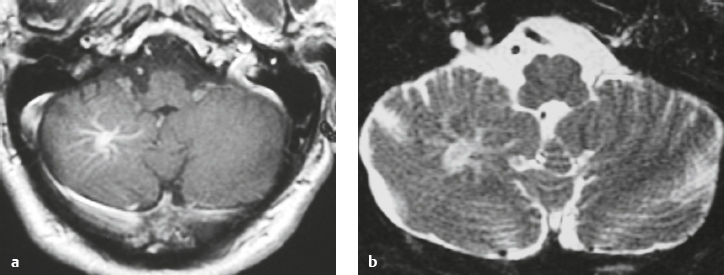
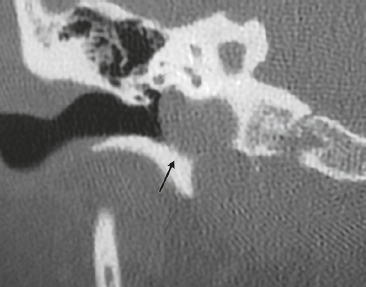
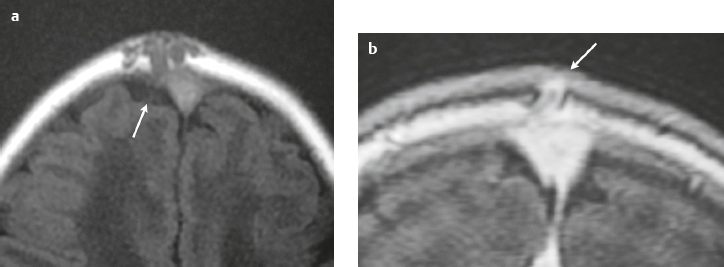
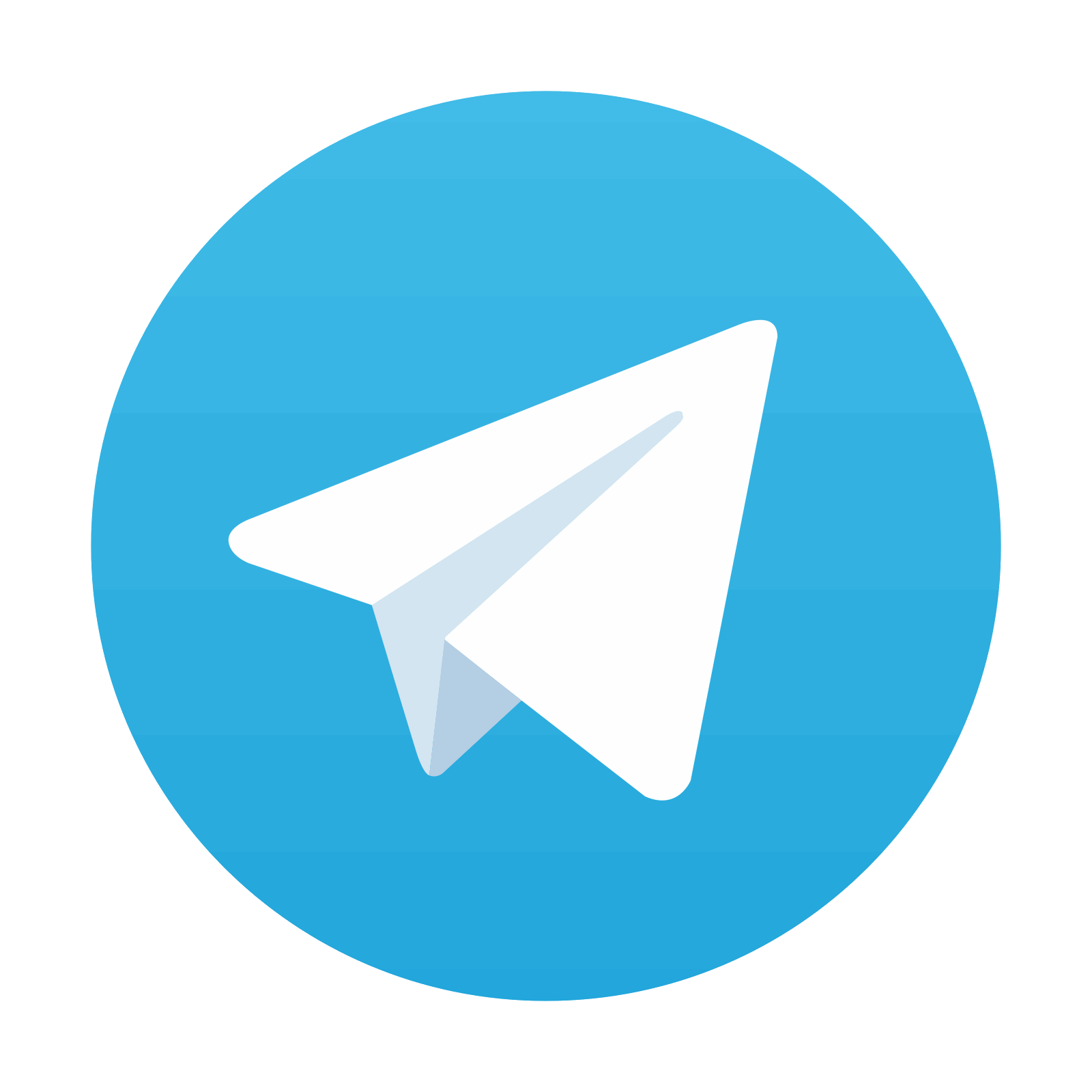
Stay updated, free articles. Join our Telegram channel

Full access? Get Clinical Tree
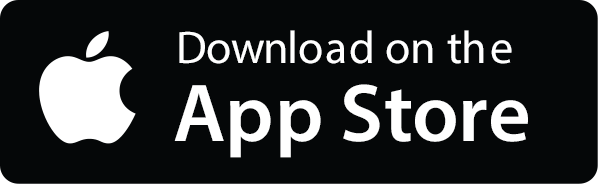
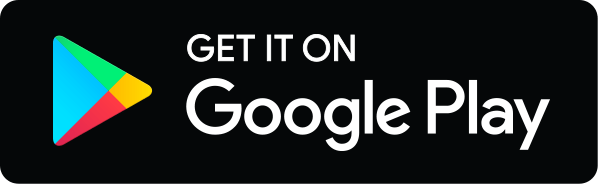
