Figure 20.1 Blood adjacent to the superior sagittal sinus. A, Axial NECT image shows SDHs in the left frontal region and within the interhemispheric fissure (arrow), which is difficult to differentiate from the superior sagittal sinus. B, Coronal reformatted image shows the high-density SDH (white arrow) outlining the normal-appearing superior sagittal sinus (black arrow).
Serial NECT (and/or MRI) of the head may increase the specificity of imaging findings, allowing the radiologist to more confidently establish the nature and age of a lesion by demonstrating interval change (15–17). This is particularly helpful in evaluating low density subdural collections (hygromas and hematohygromas), which on initial CT examinations can be mistaken for chronic SDHs.
Occasionally a brain contrast-enhanced CT (CECT) is indicated and may enable the radiologist to confidently differentiate between venous sinus thrombosis and a SDH abutting the dural sinuses or blood layering within the SAS on the surface of the brain adjacent to the dural sinus. In addition, a CECT can effectively outline the margin of a low-density or isodense SDH, which may be difficult to distinguish from CSF within the adjacent SAS (Fig. 20.2).
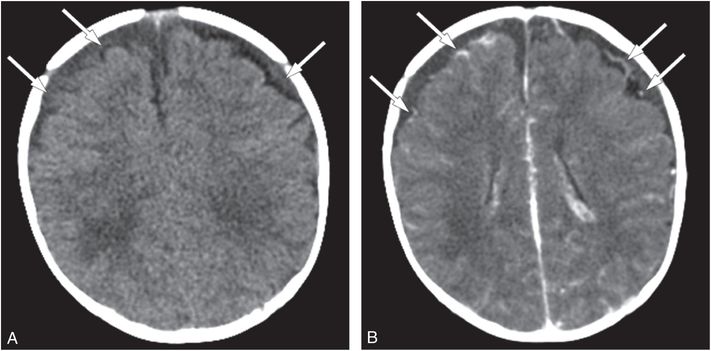
Figure 20.2 Isodense SDHs, value of CECT. Two-month-old boy presenting with new-onset seizures and no history of trauma. A, Axial NECT shows bifrontal low density SDHs (arrows). B, Axial CECT shows inward displacement of vessels (arrows) within the SAS by the space-occupying SDHs. The SS revealed multiple fractures of different ages including CMLs.
CECT may also reveal enhancing membranes within a subdural collection. If an enhancing membrane is identified within a SDH that contains acute blood in the context of an acute injury, then the hemorrhage is presumed to represent an acute on chronic bleed. In a large series by Walter et al. that examined histopathologic characteristics of SDHs, the earliest time point at which a membrane could be identified within a SDH was five days (18).
CT angiography or MR angiography is of value in assessing known or suspected neurovascular injury. A vascular study is indicated when there is evidence of hypoxic ischemic injury of the brain in an arterial distribution or if neck contusions are clinically noted and strangulation is suspected.
Since CT carries risks associated with ionizing radiation, departmental protocols should be structured accordingly. Dose reduction strategies should be employed that adjust CT acquisition parameters and scanning modes to optimally balance image noise with diagnostic efficiency (6). In our institution, we acquire head CTs in an axial mode for superior parenchymal detail, although many departments opt for the advantages of helical acquisitions, including MPRs, MIPs and 3D models.
Magnetic resonance imaging
MRI is the best imaging modality for detecting, characterizing, and dating brain injury, including parenchymal brain hemorrhage. In addition, MRI allows visualization of subtle clival and spinal subdural collections that would otherwise go undetected. MRI, however, is relatively insensitive in detecting skull fractures, particularly nondisplaced fractures, and SAH. Additional disadvantages of MRI are longer scan times, often necessitating sedation, as well as complex operational planning and consideration of safety issues needed to provide MRI to critically ill infants. In addition, scheduling an urgent or emergent MRI may be challenging due to limited availability of MRI at some sites.
Brain MRI should be performed in any child with an abnormal head CT, persistent neurologic signs or symptoms, and in children with a high index of suspicion for abuse. Additionally, a cervical spine MRI following brain imaging is important to assess for bony, ligamentous, and spinal cord injury, and complete spinal imaging will aid in detecting spinal SDHs (see Chapter 21) (19, 20). To better date intracranial blood products, MRI should be obtained as soon as possible and ideally within 1–2 days of admission. A repeat MRI performed within 5–10 days of admission is helpful in showing the evolution of intracranial abnormalities, which often increases the specificity of findings. Additional imaging should also be considered if the initial MRI findings are considered indeterminate in nature, if the neurologic status remains impaired, or if there is worsening of the neurologic condition (21). Imaging prior to discharge will document the type and extent of the injury associated with the current admission and in some cases confirms cerebral injury when the acute imaging was considered equivocal. In addition, a 3–6 month follow-up brain MRI will determine the full extent of brain injury as parenchymal volume loss from delayed cell death may continue for weeks. It is therefore prudent to image early and image often.
Given the critical role MRI can play both in clinical management and in medico-legal proceedings, we advocate thorough imaging on the highest-quality MRI scanner available. Ideally imaging should be performed at 3 Telsa using a high-density phased array brain coil and spine coil. Recommended pulse sequences are as follows:
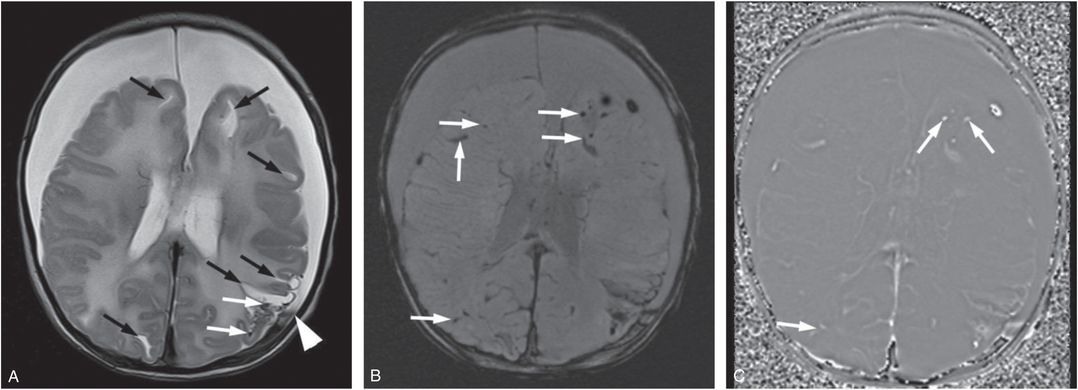
Figure 20.3 Five-week-old former 35-week preterm infant presenting with vomiting. (See same patient in Figs. 19.13, 19.14, 19.15, 19.16, 21.72.) Pyloric stenosis was ruled out and the infant returned two days later with continued vomiting and subsequent seizures. SS revealed multiple healing fractures. There were no retinal hemorrhages. A, Axial T2WI TSE. B, Axial SWI MIP. C, SWI phase image. There are multiple white matter lacerations (black arrows in A) and subdural collections. Note the layering blood products in the left SDH (white arrowhead in A). Although small foci of blood products are noted in the left posterior parietal lobe on the T2WI (white arrows), the SWI shows many more areas of hemorrhage (white arrows). On the SWI phase image these foci are bright (white arrows) consistent with blood products as opposed to calcifications.
Susceptibility-weighted sequences are particularly helpful in detecting small amounts of intraventricular hemorrhage that are inconspicuous on spin echo (SE) sequences (see Fig. 18.33). In addition, if SWI is available, indirect evidence of regional hyper- or hypo-perfusion can be obtained by relative differences in the prominence of venous deoxyhemoglobin. For instance, a regional decrease in cerebral perfusion will result in increased oxygen extraction and an increased deoxyhemoglobin-to-oxyhemoglobin ratio within venous blood. As a result, SWI can show asymmetric prominent hypointense vessels in areas of hypoperfused brain tissue. Conversely, if flow is increased, oxygen extraction within venous blood is decreased and veins become less apparent in areas of hyperperfused brain. Of note, it can be difficult to differentiate venous thrombosis from normal venous structures on SWI in cases with asymmetric prominence of draining veins or dural venous sinuses. In these cases, adding a T2 weighted GRE sequence may be helpful:
Additional sequences that may be useful include:
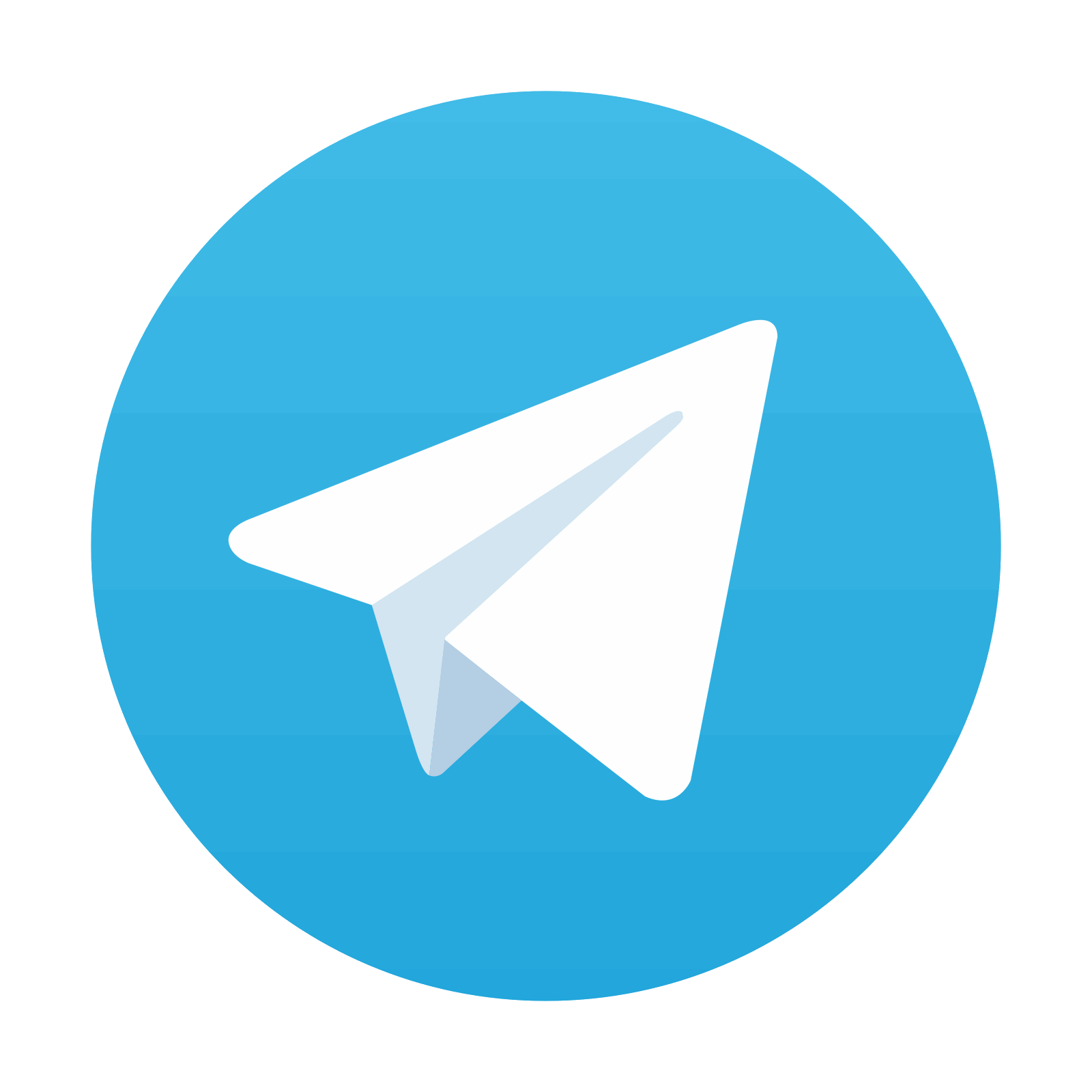
Stay updated, free articles. Join our Telegram channel

Full access? Get Clinical Tree
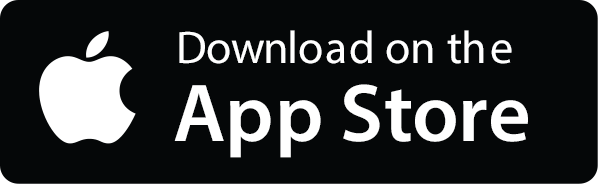
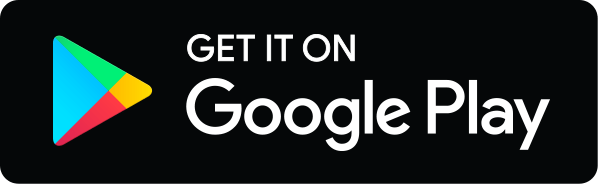