Fig. 25.1
Graph illustrating the effect of fractionation on the cell survival of tumor cells (A) as well as normal cells (B). Since normal cells have intact repair mechanisms, whereas tumor cells do not, fractionation effectively separates the cell survival curves for tumor cells and normal cells, thereby sparing normal cells while killing tumor cells. Published with permission from Balagamwala EH, Chao ST, Suh JH. Principles of radiobiology of stereotactic radiosurgery and clinical applications in the central nervous system. Technol Cancer Res Treat. 2012;11(1):3–13. Epub 2011/12/21. http://www.tcrt.org
The radiobiology of high dose single fraction involves more than sublethal damage, and the major effects have been documented to involve both lethal DNA damage and endothelial damage. While favorable for tumor cell kill, these dual effects may have adverse effects on the normal neuropil and vasculature of the cochlear nerve.
To better understand an optimal dose regimen for the treatment of acoustic neuromas, it is important to review the concept of a biologically effective dose, or BED, which is useful for quantifying treatment expectations [49] and is an inherent part of the linear quadratic formula for radiation effect. For a review of the derivation and significance of α/β [alpha/beta] ratios and the linear quadratic equation, the reader is referred to an excellent recent review by Balagamwala et al. [48]. The radiation cell kill (or effect E) can be expressed as:
![$$ \mathrm{Cell}\kern0.24em \mathrm{kill}=E=n\left(\alpha \left[\mathrm{alpha}\right]d+\beta \left[\mathrm{beta}\right]{d}^2\right) $$](https://radiologykey.com/wp-content/uploads/2017/06/A83315_2_En_25_Chapter_Equ1.gif)
where α [alpha] and β [beta] are the radiosensitivity coefficients, n the number of fractions, and d the dose per fraction such that the total dose (D) = nd.
![$$ \mathrm{Cell}\kern0.24em \mathrm{kill}=E=n\left(\alpha \left[\mathrm{alpha}\right]d+\beta \left[\mathrm{beta}\right]{d}^2\right) $$](https://radiologykey.com/wp-content/uploads/2017/06/A83315_2_En_25_Chapter_Equ1.gif)
(25.1)
Historically, radiosurgical dose reductions for acoustic tumors have been performed empirically to improve hearing outcomes. Consistent with this exercise, consider a progressive reduction in d approaching but not reaching 0. Although the number of fractions n will then need to be increased to maintain the same effect, β[beta]d 2 will be very small in comparison to α[alpha]d, and when d is very small, the above equation can be simplified to:
![$$ E=n\kern0.24em \alpha \left[\mathrm{alpha}\right]d=\alpha \left[\mathrm{alpha}\right]D $$](https://radiologykey.com/wp-content/uploads/2017/06/A83315_2_En_25_Chapter_Equ2.gif)
This demonstrates that the total dose D of radiotherapy given at a very low dose per fraction represents the highest total dose required to obtain a specific effect, in this case simultaneously high rates of tumor control and hearing preservation. This total dose represents the biologically effective dose or BED in situations where neoplastic cellular repopulation can be ignored, which is the case for acoustic neuromas. Equation (25.2) above can be redefined as:
With this assumption, BED for any practical radiotherapy application can be derived by first dividing both sides of Eq. (25.1) by α [alpha] such that:
![$$ \mathrm{BED}=E/\alpha =\frac{n\left(\alpha d+\beta {d}^2\right)}{\alpha }=nd\left[1+\frac{d}{\alpha /\beta}\right] $$](https://radiologykey.com/wp-content/uploads/2017/06/A83315_2_En_25_Chapter_Equ3.gif)
It is perhaps difficult to understand that one BED can be obtained by different dose/fractionation schedules. Drawing from an analogy by Jones and colleagues [49], the height of a mountain could be viewed as a BED. BED represents the absolute height using the longest route (i.e., the lowest dose) to the summit, but it is possible to climb to the summit by a steeper and hence shorter route. Carrying the analogy forward, the steeper route represents a shorter distance, or in biological terms, the use of larger fractions results in a shorter time course with a smaller total dose.
![$$ E=n\kern0.24em \alpha \left[\mathrm{alpha}\right]d=\alpha \left[\mathrm{alpha}\right]D $$](https://radiologykey.com/wp-content/uploads/2017/06/A83315_2_En_25_Chapter_Equ2.gif)
(25.2)
![$$ \mathrm{BED}=D=E/\alpha \left[\mathrm{alpha}\right] $$](https://radiologykey.com/wp-content/uploads/2017/06/A83315_2_En_25_Chapter_Equa.gif)
![$$ \mathrm{BED}=E/\alpha =\frac{n\left(\alpha d+\beta {d}^2\right)}{\alpha }=nd\left[1+\frac{d}{\alpha /\beta}\right] $$](https://radiologykey.com/wp-content/uploads/2017/06/A83315_2_En_25_Chapter_Equ3.gif)
(25.3)
Currently, disparate focused radiation dose/fractionation schedules are practiced worldwide for acoustic tumors, including single fraction SRS, hyperfractionated FRS, and hypofractionated FSR. Doses of 12 Gy in a single fraction, 46.8 Gy in 1.8 Gy daily fractions, or 18 Gy in three fractions all achieve excellent tumor control rates with serviceable hearing preservation rates that vary roughly from 60 to 80 %. One might argue that these dose/fractionation schedules are not biologically equivalent because of variable outcomes in hearing preservation, but for purposes of this model, we will assume they are. We can correspondingly assign values of BED1, BED2, and BED3, respectively, to these dose/fraction schemes. Assuming BED1 = BED2 for tissue of an unknown α/β, or in terms of total dose D and fraction dose d:
D1[1 + d1/(α/β[alpha/beta])] = D2[1 + d2/(α/β[alpha/beta])] or α/β[alpha/beta] = (D1 × d1 − D2 × d2)/D2 − D1
For acoustic neuromas using the assumption of equivalent BED for 12 Gy in a single fraction (d1 = 12 Gy) and 46.8 Gy in 26 fractions (d2 = 1.8 Gy):
and the corresponding BED based on this α/β[alpha/beta] ratio is calculated as:
Now having derived an isoeffective BED for acoustic tumors, we can test an alternate dose-fractionation schedule by substituting actual values from Eq. (25.3) above for BED3, an actual dose-fractionation schedule recently reported [50] to see if our derived α/β [alpha/beta] ratio actually yields the same dose per fraction solving for d as the unknown:
which is rearranged to:
For a general quadratic equation such as ad 2 + bd + c = 0, the positive solution for d is given by:
where in this case a = 1.74, b = 3, and c = −95.7. The solution for d above is 6.6 Gy which is within a 10 % error of 6 Gy. As a working model, therefore, an α/β[alpha/beta] ratio of 1.72 yielding an isoeffective BED of 95.7 Gy is a reasonable derivation from current literature regarding focused radiation for acoustic neuromas.
![$$ \begin{array}{c}\alpha /\beta \left[\mathrm{alpha}/\mathrm{beta}\right]=\left[\left(12\times 12\right)-\left(46.8\times 1.8\right)\right]\;/\;\left(46.8-12\right)\\ {}=1.72\end{array} $$](https://radiologykey.com/wp-content/uploads/2017/06/A83315_2_En_25_Chapter_Equb.gif)

![$$ 3d\left[1+\frac{d}{1.72}\right]=95.7\kern0.24em {\mathrm{Gy}}_{1.72} $$](https://radiologykey.com/wp-content/uploads/2017/06/A83315_2_En_25_Chapter_Equd.gif)
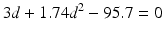
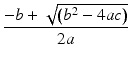
While an α/β[alpha/beta] ratio of 1.72 is low and consistent with late-responding normal CNS tissues [51], this model has potential pitfalls including an oversimplification of single Gamma Knife treatments which necessarily involve multiple isocenter hot spots, and a two-target model (DNA damage and endothelial cell apoptosis) may be necessary, especially at high doses per fraction. In the former case, a single one-point calculation will not be representative of the biological effect throughout a larger acoustic tumor with multiple hot spots, and multiple BED evaluations would be necessary [49]. In the latter case, a modification of the linear quadratic equation such as a generalized LQ (gLQ) [52] or a universal survival curve (USC) [53] might be more appropriate. As a starting point, however, we felt this model would be useful for dose-fractionation schedules designed for treatment of acoustic tumors. Its utility as a predictive model of serviceable hearing loss, however, remains unclear due to the significant variations in hearing outcomes mentioned above.
Derivation of a Hearing Ret Formula for Acoustic Neuromas
We felt a more systematic approach to serviceable hearing preservation would be the derivation and application of a hearing RET formula [42]. A prospective trial by Pan et al. [54] documented that for almost all cases in which significant hearing loss occurred in the affected ear receiving radiation, the dose was ≥45 Gy. Among published data available, we were able to plot the log of dose versus the log of the number of fractions drawing from Goldsmith’s model [3] for reports with hearing preservation rates of at least 70 % based on Gardner-Robertson criteria. The linear regression gave us a formula for dose/fraction size regimens with a high correlation coefficient (R2 = 0.9705). Drawing from Goldsmith’s optic RET derivation, we postulated that dose/fraction schemes with very high hearing preservation rates could be represented by a parallel line on the same plot, intersecting a point corresponding with the dosage/fraction scheme documented as safe. Data from Pan et al. [54] suggest that 45 Gy in 25 × 1.8 Gy fractions (or less) is such a point. By substitution to the linear regression equation:
So the line equation is:
where N is the number of fractions. This can be folded into: D = 1,120 × N0.43 or 1,120 hearing RET = D × N − 0.43 for dose/fraction schemes with high hearing preservation rates.


For one fraction: D = 11.2 Gy. This implies that the radiosurgical dose should not exceed a threshold around 11.2 Gy to obtain high rates of hearing preservation. Based on this hearing RET formula, single fraction doses above 11.2 Gy or a cumulative FSR dose above 45 Gy in a 25 fraction regimen could result in higher rates of hearing loss. For SRS, any dose below 13 Gy results in loss of tumor control [23], so even with an approximate 60 % hearing preservation rate, 13 Gy may represent the most optimal dose achievable with SRS. Based on our previous literature review, a median value of 13 Gy has, in fact, resulted in reported serviceable hearing losses ranging from 17 to 67 % [42]. A median FSR dose of 47.5 Gy, while maintaining excellent tumor control, has resulted in reported serviceable hearing losses ranging from 29 to 43 % [42]. As noted in Fig. 25.2b below, the dose prescription schemes with the highest yield of hearing preservation fall within the box that includes the 10 % variance of the α/β [alpha/beta] ratio we derived. The single point represents a recently published paper which describes a high probability of hearing loss with a dose-fractionation scheme for acoustic neuromas described as a total dose of 54 Gy in 27 fractions [55]. Applying an α/β [alpha/beta] ratio of 1.63 for tumor control and the hearing RET formula as a guide for hearing preservation, the total dose should not exceed 46.2 Gy for 27 fractions to maximize the chance of hearing preservation while maintaining excellent tumor control.
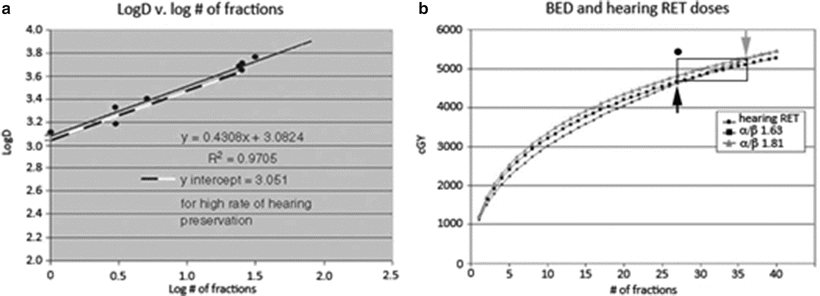
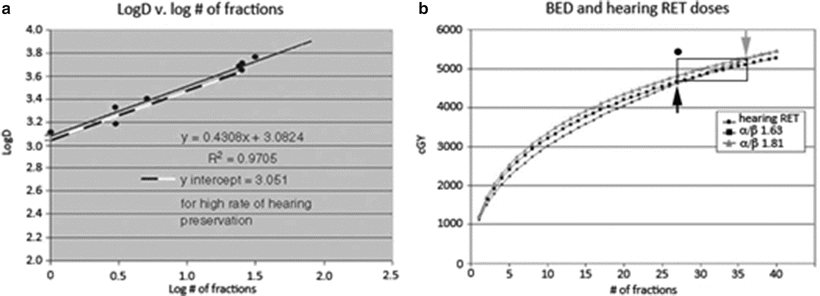
Fig. 25.2
(a) Logarithmic plot of total dose versus number of fractions for cases reporting preservation of serviceable hearing; (b) plots of biologically equivalent doses utilizing the linear quadratic formula with α/β [alpha/beta] ratios as +10 % of the calculated α/β [alpha/beta] value, in comparison with the hearing RET plot. The dose prescription schemes predicting the highest yield of hearing preservation fall within the box at or below the hearing RET curve depending on which α/β [alpha/beta] ratio is utilized. The black arrow represents the point beyond which dose-fractionation schemes predict a higher likelihood of hearing preservation for a lower α/β [alpha/beta] ratio, the gray arrow for a higher α/β [alpha/beta] ratio. The black point represents a recent study which documented a high rate of hearing loss [56] and falls above the hearing RET range
Further Radiobiological Advantages: The Example of Large Tumors
With the progressive shift in treatment patterns toward use of radiation therapies for acoustic neuromas, some authors have suggested that relative indications for microsurgical resection include the presence of medically refractory trigeminal neuralgia, hydrocephalus, symptomatic mass effect including disabling ataxia or hemiparesis, intractable headache, and large tumor size. Although the definition of large tumor size remains somewhat arbitrary, maximal tumor diameter >3 cm has conventionally been used as a cutoff for SRS treatment based on studies demonstrating rates of effective tumor control as low as 57 % and increased risk of complication compared to SRS treatment of small- and medium-sized tumors [56]. Milligan et al. described their experience using SRS for tumors with volumes more than one standard deviation above the mean during a 9-year period and demonstrated a 5-year actuarial tumor control rate of 83 % [57]. Impairment of facial nerve function was observed in 14 % of patients and 15 % required prolonged corticosteroid administration for symptomatic radiation-induced brainstem or cerebellum edema. Based on their results, the authors recommended consideration of surgical resection for tumors larger than 2.7 cm in posterior fossa greatest dimension. A group from the Netherlands recently reported primary SRS treatment of 33 patients with large tumors defined as tumor volume >6 cm3 with indentation of brainstem (Koos Grade III & IV). Patients with NF2, symptomatic mass effect, or maximum extracanalicular dimension ≥4 cm were excluded from primary SRS treatment. A radiographic tumor control rate of 88 % was reported over a median follow-up time of 30 months, although 7 of 33 patients required further microsurgical or SRS treatment for development of either progressive neurologic symptoms or radiographic enlargement. Transient facial and trigeminal neuropathy occurred in 9 % and 14 % of patients, respectively, with good facial nerve function (HB Grades I and II) preserved in all patients at 1 year posttreatment. Interestingly, of the 12 patients included in the study who reported pretreatment symptoms of trigeminal hypoesthesia, 11 (92 %) experienced resolution of their condition following SRS likely related to the 67 % incidence of tumor volume shrinkage observed on radiographic follow-up (Table 25.1).
Table 25.1
Results of the ANA surveys over three decades
Treatment reported by respondents | 2007–2008 (%) | 1998 (%) | 1983 (%) |
---|---|---|---|
Translabyrinthine approach | 33 | 51 | 72 |
RETrosigmoid approach | 17 | 28 | 11 |
Middle fossa approach | 10 | 6 | 3 |
Don’t know approach | 0 | 0 | 14 |
Total, microsurgical resection | 60 | 85 | 100 |
Stereotactic radiosurgery (SRS) | 12 | NA | NA |
Fractionated stereotactic radiotherapy (FSR) | 8 | NA | NA |
Total SRS/FSR | 20 | 5 | 0 |
Watch and wait | 20 | 4 | 0 |
Don’t know what type of treatment | NA | 6 | 0 |
Total | 100 | 100 | 100 |
The use of FSR has been recommended as an alternative radiation modality for the treatment of larger tumors adjacent to the brainstem although minimal data exists to suggest improved outcome relative to SRS for this population of tumors. Mandl et al. reported a cohort of patients with tumors >3 cm in diameter treated primarily with multisession SRS using a maximum dose of 25 Gy delivered in 5 Gy fractions [58]. The actuarial 5-year tumor control and facial nerve function rates were 82 % and 80 %, respectively. We have updated our review of FSR treatment for acoustic neuromas, and of 154 tumors treated in the 46.8 Gy cohort, 15 were >6 cc in size. In this subgroup, we have documented no treatment failures, and of 6 patients with serviceable hearing, 3 have maintained hearing in a serviceable range at a median follow-up of 35 months. At conventional fraction sizes, we have also noted no other cranial neuropathies in this subgroup of patients. See Fig. 25.3 as an example.
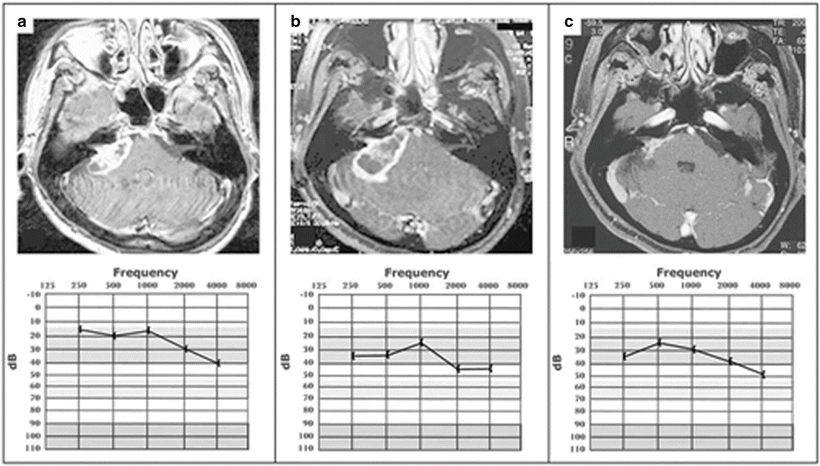
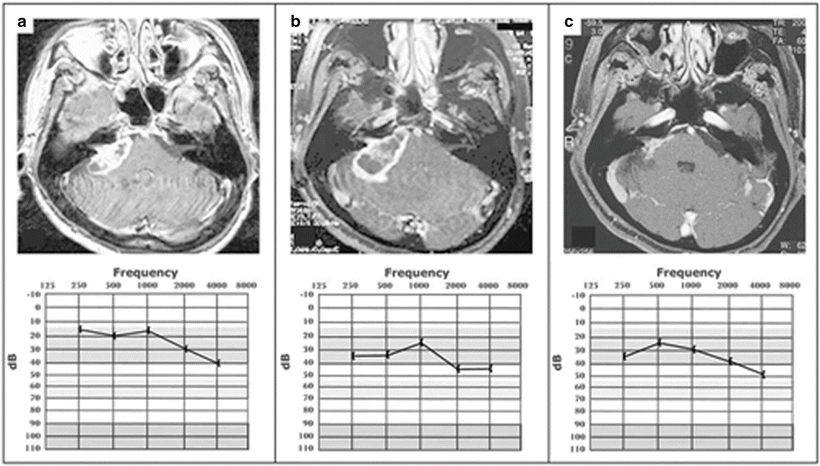
Fig. 25.3
Serial magnetic resonance imaging scans (upper) with associated audiograms obtained at same time (lower) in patient treated with FSR to 46.8 Gy. (a) Pretreatment T1-weighted gadolinium-enhanced axial image featuring right acoustic neuroma. Audiogram reflects pure tone average of 22 and speech discrimination score of 95 %, representing Gardner-Robertson level 1 pretreatment serviceable hearing. (b) Posttreatment T1-weighted gadolinium-enhanced axial image at 6 months featuring some enlargement and extensive central necrosis of tumor. Audiogram at 6 months after treatment reflects some audiometric decay with pure tone average of 35 and speech discrimination score of 100 %, representing a decrease to Gardner-Robertson level 2 serviceable hearing. (c) Posttreatment T1-weighted gadolinium-enhanced axial image at 42 months featuring marked diminution in tumor size. Audiogram at 42 months after treatment reflects stable audiogram with pure tone average of 30 and speech discrimination score of 92 %, back to lowest range of Gardner-Robertson level 1 serviceable hearing. From Andrews DW, Werner-Wasik M, Den RB, Paek SH, Downes-Phillips B, Willcox TO, et al. Toward dose optimization for FSR for acoustic neuromas: comparison of two dose cohorts. Int J Radiat Oncol Biol Phys. 2009;74(2):419–26
The theoretical advantages of LINAC-based fractionation for large tumors are depicted in Figs. 25.4 and 25.5 below. For larger treatment volumes, the penumbra affecting contiguous normal tissues is often judged to be prohibitive for single fraction SRS that is substantiated in a broad literature. In a review of 149 patients with posterior fossa tumors treated with SRS, for example, factors that predicted cranial nerve palsy included D max of >17.5 Gy, a prescribed isosurface dose of >12.5 Gy, length of cranial nerve of >16 mm, and tumor volume of 1.7 cc [59]. Particularly for larger acoustic tumors, both the dose prescription convention and the design of multiple isocenters to achieve conformality increase the likelihood of overdosing the cochlear nerve.
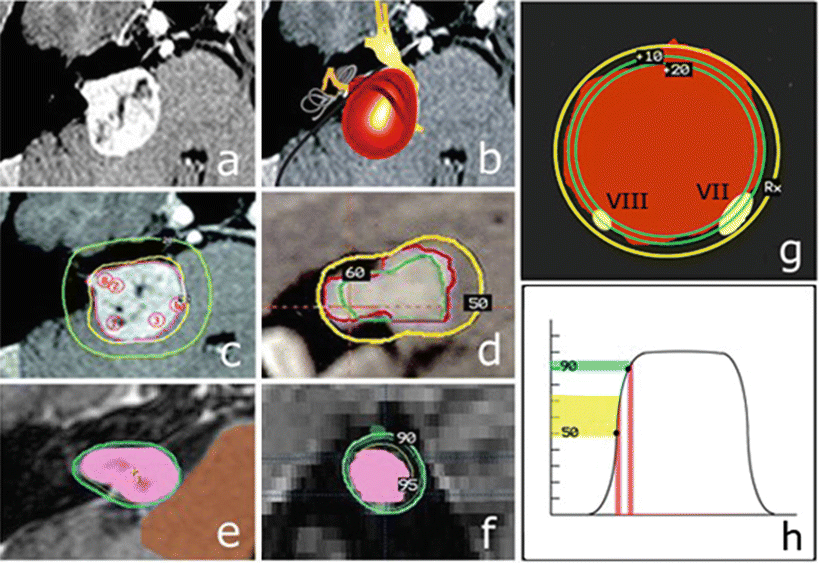
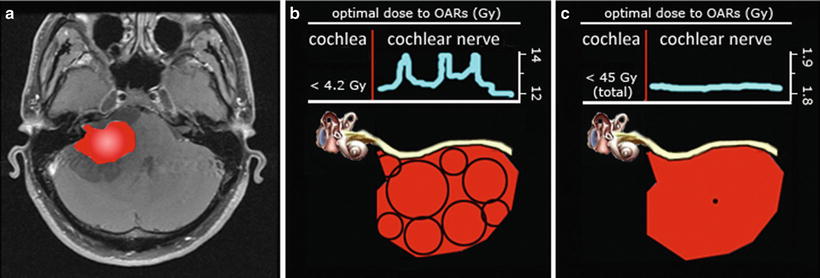
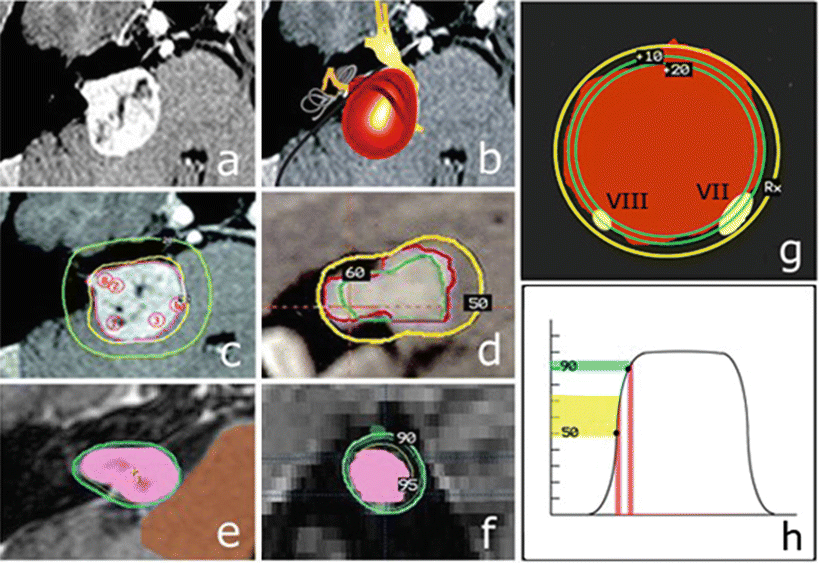
Fig. 25.4
Comparison of dose distribution to the cochlear nerve with Gamma Knife and FSR treatments based on isosurface prescription conventions. (a) Axial T1 gadolinium-enhanced MRI scan of right acoustic neuroma. (b) Artist’s rendering of translucent acoustic tumor with cranial nerves VII and VIII adherent to the anterior and caudal surface of the tumor, coursing to internal auditory canal. (c) Eight-shot Gamma Knife radiosurgery treatment plan with a 12 Gy prescription to the 50 % isodose line (yellow) for right acoustic neuroma. (d) Magnified sagittal view of actual treatment plan in the distal porus acusticus [outer line (yellow) is 50 % isodose prescription line; middle line (magenta) is tumor surface; inner line (green) is 60 % isodose line]. Assuming cochlear nerve is around the 7 o’clock position, the nerve is within a 10 % dose gradient above isodose prescription. (e) Single-shot Novalis FSR treatment plan with a 1.8 Gy prescription to the 90 % isodose line for a right acoustic neuroma (tumor is shaded; outer line is 90 % isodose prescription line). (f) Magnified sagittal view of actual treatment plan in the distal porus acusticus (tumor is shaded; outer line is 90 % isodose 10 prescription line; inner line is 95 % isodose line). Assuming cochlear nerve is around the 7 o’clock position, the nerve is within a 5 % dose gradient above isodose prescription. (g) Artist’s caricature of (d) (magnified sagittal cross section of intracanalicular portion of tumor treated with Gamma Knife). The VIII nerve at the 7 0’clock position is within the prescribed isodose line and exposed to higher dose gradients. (h) Profile of a focused radiation beam with typical isodose prescriptions at 50 % (Gamma Knife) and 90 % (FSR). Vertical columns in either scenario could represent narrow location range of cochlear nerve relative to tumor (e.g., within 1 mm of tumor surface). Broad inferior horizontal bar represents potential actual dose range delivered to cochlear nerve with 50 % isodose prescription; narrow superior horizontal bar represents potential actual dose range delivered to cochlear nerve with 90 % isodose prescription. The potential dose gradient at a 50 % isodose prescription is more than three times greater than 90 % prescription at the same distance
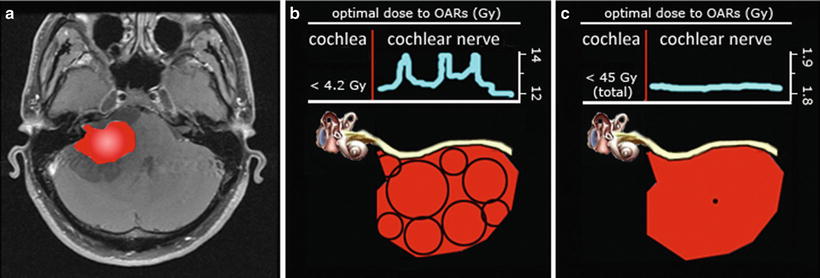
Fig. 25.5
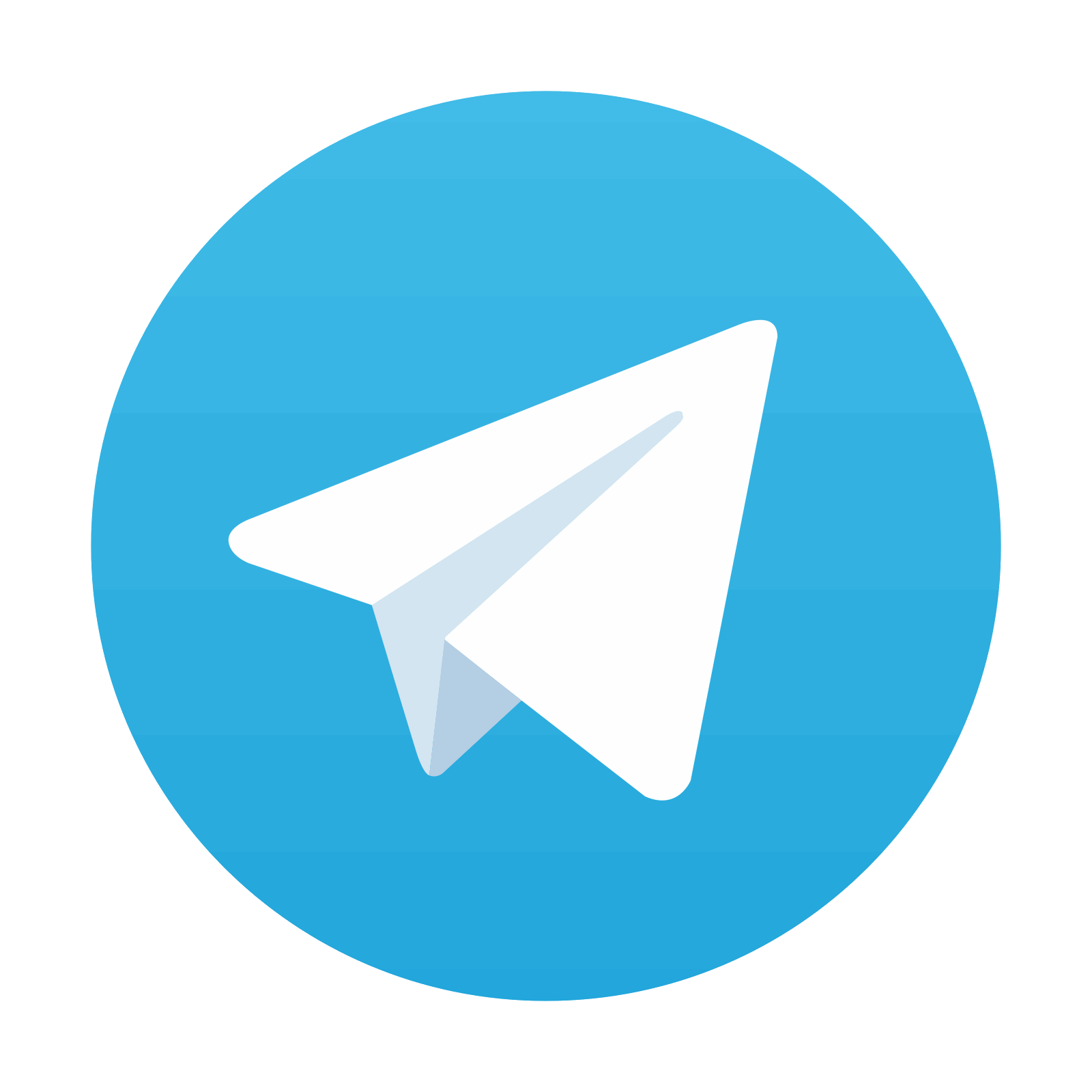
Comparison of dose distribution to the cochlear nerve with Gamma Knife and FSR treatments based on dose delivery conventions. (a) Axial T1 gadolinium-enhanced right acoustic neuroma, highlighted in red. (b) Cartoon of the same tumor now featuring the cochlear nerve and cochlea (OARs are organs at risk). After a multiple-shot, high conformality treatment, the dose penumbra including the cochlear nerve necessarily includes hot spots from overlapping shots. 4.2 Gy is threshold beyond which injury to the cochlea results in hearing loss [31]. (c) After a single isocenter dynamic arc treatment with mini-multileaf collimation treatment, the dose penumbra including the cochlear nerve is now much more homogeneous and close to the isosurface prescription dose. A total dose of 45 Gy with conventional fraction sizes is a threshold beyond which injury to the cochlea results in hearing loss [55]
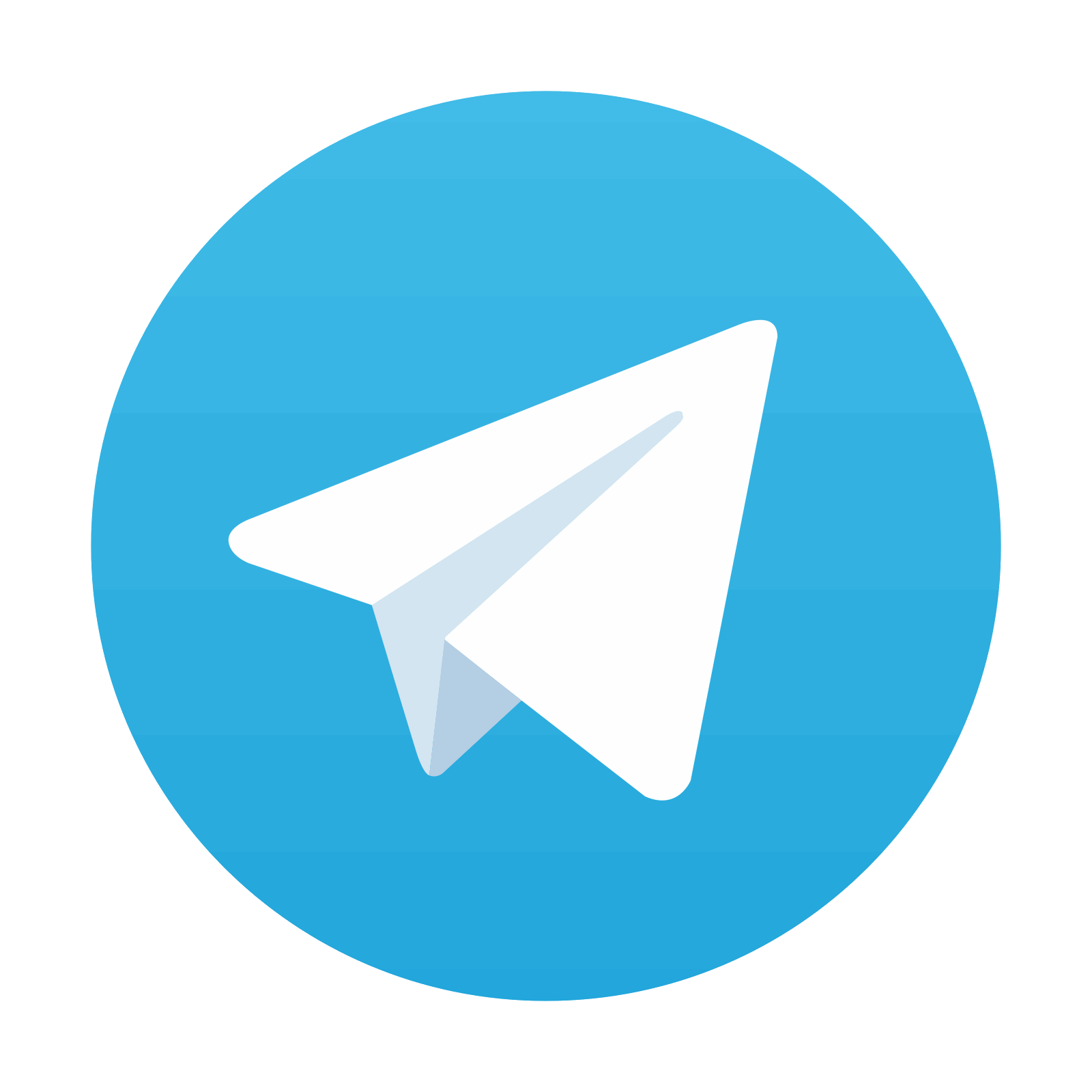
Stay updated, free articles. Join our Telegram channel

Full access? Get Clinical Tree
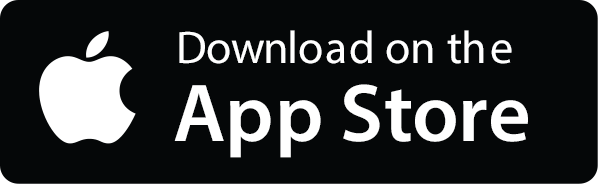
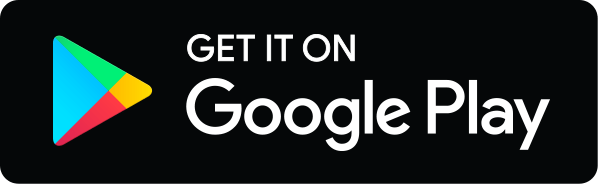
