Major vascular complications
1. Any thoracic aortic dissection
2. Access site or access-related vascular injury (dissection, stenosis, perforation, rupture, arteriovenous fistula, pseudoaneurysm, hematoma, irreversible nerve injury, or compartment syndrome) leading to either death, need for significant blood transfusions (≥4 units), unplanned percutaneous or surgical intervention, or irreversible end-organ damage (e.g., hypogastric artery occlusion causing visceral ischemia or spinal artery injury causing neurological impairment)
3. Distal embolization (non-cerebral) from a vascular source requiring surgery or resulting in amputation or irreversible end-organ damage
Minor vascular complications
1. Access site or access-related vascular injury (dissection, stenosis, perforation, rupture, arteriovenous fistula or pseudoaneurysms requiring compression or thrombin injection therapy, or hematomas requiring transfusion of ≥2 but <4 units) not requiring unplanned percutaneous or surgical intervention and not resulting in irreversible end-organ damage
2. Distal embolization treated with embolectomy and/or thrombectomy and not resulting in amputation or irreversible end-organ damage
3. Failure of percutaneous access site closure resulting in interventional (e.g., stent graft) or surgical correction and not associated with death, need for significant blood transfusions (≥4 units), or irreversible end-organ damage
Complication Rates
Reported vascular complication rates associated with percutaneous aortic valve placement have ranged widely from 1.9 to 30.7 % [6, 9–19] that may be, in part, due to the lack of standardized definitions for vascular complications in TAVR in the past. For example, many studies have reported only complications requiring further surgical or percutaneous intervention. A meta-analysis of 16 outcome studies employing the VARC criteria published 15 months following their release showed an 11.9 % incidence of major vascular complications (range 5.0–23.3 %), a 9.7 % incidence of minor vascular complications (range 5.6–28.3 %), with a composite incidence of any vascular complication 18.8 % (range 9.5–51.6 %) [20].
Early percutaneous heart valve systems required 22–25 F sheaths to accommodate the large catheters used for valve delivery and were associated with rates of major bleeding as high as 50 % [21]. Several subsequent trials have demonstrated that patients with either a femoral or apical access site complication have 30-day mortality rates twice that of other individuals undergoing the procedure [9, 16]. Given these dangers, great effort has gone into identifying risk factors for vascular complication, and routine screening has been implemented with respect to iliofemoral vascular access. Newer low profile systems have been designed to be compatible with smaller 18 F sheaths in an effort to decrease the incidence of access vessel injury as well as the number of patients that may be precluded from having a transarterial TAVR due to small-caliber iliofemoral vessels [22].
Several large outcome studies suggest that vascular complications are the most common major adverse event seen in association with TAVR [9, 16, 23, 24]. These studies report vascular complication occurring in 10–23 % of procedures performed via femoral arterial approach with access site complications occurring in 13–18 % of such procedures after MDCT screening. Notably, procedures performed via the transapical approach demonstrate similar rates of access site complication.
It appears that with experience, and perhaps more stringent patient selection, vascular complication rates may decrease over time. The frequency of vascular complications decreases with operator experience, 12–4.5 % after 300 cases in one series [23]. This study also demonstrated that access site complication rates were significantly lower with a surgical cutdown of the femoral artery than when a percutaneous closure device was employed (5.9 % vs. 16.8 %) [23]. In contrast, when a fully percutaneous procedure is performed routinely, VARC vascular complication rates have been shown to decrease from 32 to 9 % with increased user experience [25]. This has been attributed to more careful patient selection based, in part on findings from MDCT, as well as increased experience with percutaneous vascular closure techniques.
Risk Factors for Vascular Injury
The experience from endovascular aneurysm repair (EVAR) would suggest that patients at the greatest risk of complication resulting in iliofemoral vessel injury are those with excessive iliac tortuosity, circumferential vessel wall calcification, significant occlusive disease, and small-caliber vessels [26]. Using these guidelines, it has been suggested that 32–35 % of patients at high surgical risk for surgical aortic valve repair (SAVR) have at least one criterion unsuitable for iliofemoral access by MDCT evaluation [24, 27].
Recent work employing MDCT screening prior to retrograde iliofemoral access confirmed that moderate to severe arterial calcification is associated with a threefold increase in vascular complications (29 % vs. 9 %), and the presence of a minimal arterial lumen diameter less than that of the external sheath is associated with a fourfold increase in vascular complications (23 % vs. 5 %) [28]. A systematic analysis assessing arterial minimum lumen diameter in relation to the external sheath diameter has revealed that a sheath to femoral artery ratio (SFAR) of 1.05 or greater is predictive of VARC vascular complications and 30-day mortality [8]. Subgroup analysis of the sample population further demonstrated that this threshold may be more lenient (SFAR = 1.10) if there is no calcification of the iliofemoral vessels and conversely, stricter (SFAR = 1.00) in the presence of mild to severe calcification.
When vascular access is established for a TAVR procedure, the femoral arteries are sequentially dilated to allow passage of the sheath through the iliac arteries and into the aorta. Experience has shown that a long sheath extending directly from the femoral artery to the aorta is preferred over a short sheath as catheter introduction into the iliac arteries has been associated with iliac arterial dissection [3].
The minimum diameter of the iliofemoral arteries used for vascular access should ideally exceed that of the introducer sheath. However, short segments of narrowing in relatively compliant arteries (those without extensive calcification or atheroma) can usually be safely cannulated if they are no more than 1–2 mm smaller in diameter than the delivery system [22, 27]. Occlusive femoral sheaths have been associated with adherence of the vascular endothelium to the sheath itself resulting in complete arterial avulsion upon withdrawal of the sheath. Heavy circumferential (>60 %) calcification of the common iliac arteries has also been shown to be associated with arterial hemorrhage. If the introduction sheath is occlusive, this may not become apparent until the time of sheath removal [3].
Device Sizes
In order to evaluate iliofemoral access, it is of fundamental importance to understand the delivery devices used, including a working knowledge of their materials and diameters. Conventional nomenclature for the sizing of catheters uses the French system (Fr) to describe their outer diameter. Access sheaths are also described using the French system, although the French value assigned to a sheath refers to the maximum catheter size that will fit within its lumen. It does not relate to the outer diameter of the sheath, which is the diameter of interest when evaluating the size of the iliofemoral vessels. To predict size mismatch between the external device diameter and the arterial luminal diameter requires familiarity with the sheath systems employed. This in turn may depend on the implanted valve prosthesis size. Delivery system profiles have decreased since the first transcatheter valve was placed in 2002 (Table 21.2) and it is expected that this trend will continue as newer systems are developed. The newest generation of sheaths employed with Edwards Sapien XT system is a 16 F expandable sheath, which is smaller than the balloon-mounted valve. The sheath expands transiently as the valve is passed through it, recoiling afterwards so as to not become adherent to narrow access vessels. However, decreasing sheath diameters may come at the expense of stiffness of the delivery system. Similar to vessel size, a mismatch between system stiffness and vessel tortuosity may represent a quantifiable risk.
Table 21.2
Component profile and desired minimum arterial diameter by MDCT measurement of the most common current and historic balloon-mounted and self-expandable stents used for retrograde femoral access
– | Valve size (mm) | Delivery system | Nominal sheath diameter (F) | Outer diameter (mm) | Desired mean arterial diameter (mm)b |
---|---|---|---|---|---|
Cribier Edwards (2002) | 23 | – | 22 | 8.4 | 8.0 |
26 | 24 | 9.2 | 8.8 | ||
Edwards SAPIEN (2005) | 23 | RetroFlex 3 | 22 | 8.4 | 8.0 |
26 | 24 | 9.2 | 8.8 | ||
Edwards SAPIEN XT (2009) | 23 | NovaFlex | 18 | 7.2 | 6.9 |
26 | 19 | 7.5 | 7.1 | ||
Edwards SAPIEN XT (2011) | 23 | NovaFlex + (eSheath) | 16 | 6.7 | 6.4 |
26 | 18 | 7.2 | 6.9 | ||
20 | 8.0 | 7.6 | |||
CoreValve 1st Generation (2004) | 21 | – | 25 | 9–10 | – |
CoreValve 2nd Generation (2005) | 21 | – | 21 | ~8 | – |
CoreValve 3rd Generation (2006) | 26 | Large Check Flow Introducer Systema | 18 | 7.3 | 6.9 |
29 | 18 | 7.3 | 6.9 | ||
31 (2011) | 18 | 7.3 | 6.9 |
Assessment of Iliofemoral Vessels
Early series utilizing both the balloon expandable Edwards valve (Edwards Lifesciences, Irvine, California) and the self-expandable CoreValve ReValving system (Medtronic Core Valve, Irvine, California) were performed in 2005–2006 [3, 21]. These initial series used single plane angiography as the primary modality to assess luminal patency of the iliofemoral access vessels. There are inherent limitations to this method which include the inability to adequately assess the 3D nature of vascular tortuosity and the eccentricity of occlusive disease [29]. The burden of atherosclerotic plaque and vascular calcification may also be underestimated. These limitations may have contributed to high complication rates in early series.
Current angiographic practice employs dual-plane angiography and MDCT, or MDCT alone, for iliofemoral evaluation. MDCT is increasingly being recognized as the de facto gold standard as it is the only technique capable of accurately quantifying minimum vessel diameter, calcification, and tortuosity. Angiographic evaluation of iliac vessels is a useful adjunct to coronary angiography in patients for whom only non-contrast MDCT can be obtained. It has been shown that a standardized approach to patient screening and selection with MDCT reduces morbidity and mortality rates from vascular injury [25].
Intravascular ultrasound (IVUS) could also possibly be used to gauge luminal diameter and plaque burden; however, it is unable to accurately gauge the degree of vascular calcification or provide any practical information on vessel tortuosity. IVUS and magnetic resonance angiography for iliofemoral evaluation is discussed in later chapters.
Multidetector Row CT Angiography
MDCT has evolved considerably since its first inception in 1991 [30] and has had a tremendous impact on vascular imaging. The introduction of multiple-detector-row CT with increasing numbers of detector channels and faster gantry rotation speeds has virtually replaced diagnostic catheter angiography in almost all vascular territories. The synchronization of CT data acquisition with the patient’s electrocardiogram (ECG) – first conceived in the 1970s – became a clinical reality with MDCT. This capability is now widely available on 64-detector-row or higher CT systems for imaging the coronary arteries but has been used extensively for preoperative and postoperative imaging of the aortic valve and root as well [31, 32].
CT Angiography for TAVR Planning
The three essential components for cardiovascular CT and CT angiography (CTA) are (a) a high-resolution volumetric CT acquisition, (b) synchronized with contrast medium enhancement of the target vasculature, and (c) 3D (or 4D) image post-processing. If the ascending aorta, aortic root, and heart are of particular diagnostic importance, the thoracic portion of the CT angiographic acquisition is acquired using ECG gating to suppress cardiac motion and pulsation artifacts. This technique – which also used for the preoperative assessment of patients with aortic root aneurysms and acute aortic syndromes – is described in greater detail elsewhere [31, 32].
CT Protocol
Our own institution’s acquisition protocol for evaluation of patients being considered for TAVR consists of a non-contrast-enhanced acquisition through the chest, abdomen, and pelvis, followed by a contrast-enhanced CT angiographic acquisition of the chest, abdomen, and pelvis using a single injection of contrast medium and a single breath-hold (Fig. 21.1). Depending on the scanner used, there is a brief interscan delay of up to 4 s between the EKG-gated thoracic and non-gated abdominal and pelvic acquisitions. The total scan time with a 64-channel CT system is in the order of 15–20 s.
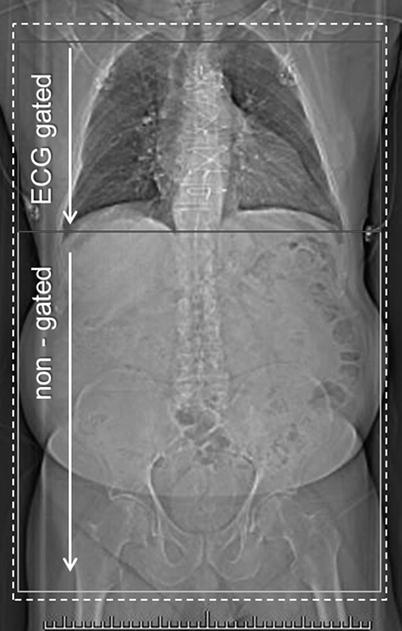
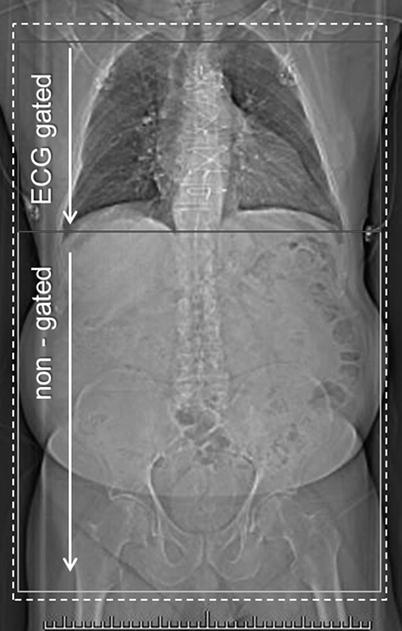
Fig. 21.1
CT angiography for transcatheter aortic valve replacement. Scanning range, thoracic portion (indicated by red box) acquired with ECG gating, abdominal portion non-gated (yellow box). Both acquisitions are obtained with a single contrast bolus. Total scan time (breath-hold) ~15–20 s
Non-contrast images are reconstructed with a section thickness of 2.5–3.0 mm. The contrast-enhanced data are typically reconstructed with a section thickness of 1 mm (0.5–1.25 mm) and at a section spacing of 1 mm or less. In-plane resolution is 512 × 512. An example of scanning parameters for a 64-channel MDCT scanner is shown in Table 21.3.
Table 21.3
Sample MDCT scanning parametersa for TAVR candidate screening
Scan I – non-contrast | Scan II – angiogram | |
---|---|---|
Section thickness (mm) | 3 | 1.0 |
Reconstruction interval (mm) | 3 | 0.7 |
Tube voltage/current (eff. mAs) | 120 kV/200 | Gated: 120 kV/320 |
Non-gated: 120 kV/219 | ||
Detector configuration (mm) | 2 × 32 × 0.6 | 2 × 32 × 0.6 |
Scan range | Above aortic arch to the lesser trochanter | Gated: Above the aortic arch to diaphragm |
Non-gated: Diaphragm to lesser trochanter | ||
Total scan time (s) | ~12 | Gated chest: ~15 |
Interscan delay: 4 | ||
Abdomen: ~7 | ||
Scanning delay | 6 s (automated breathing instructions) | Minimum (automated bolus triggering from region of interest in ascending aorta) |
Contrast medium | – | 370 mg I/mL preferred |
300 mg I/mL if azotemic | ||
Injection parameters | – | Biphasic – See Table 21.4 |
Reconstruction phases (gated portion) | – | Best diastolic (automated selection, usually ~65–70 % RR): entire scan range
![]() Stay updated, free articles. Join our Telegram channel![]() Full access? Get Clinical Tree![]() ![]() ![]() |