Luke R. Wilkins • Wael E. Saad
Gastric and esophageal varices can have multiple etiologies. Most (90%) patients have underlying cirrhosis and portal hypertension. This patient population has a 30% risk of developing varices, with gastric varices representing 10% to 20%. Although the bleeding risk associated with gastric varices is less than that associated with esophageal varices, gastric varices are accompanied with higher rates of morbidity and mortality.1 Treating patients with gastric variceal bleeding necessitates a multidisciplinary team approach, including hepatologists, endoscopists, diagnostic radiologists, and interventional radiologists.1,2
Although upper gastrointestinal (GI) endoscopy is the first-line diagnostic and management tool for upper GI bleeding secondary to varices, endovascular treatment is playing an ever-increasing role in definitive treatment.1,2 From the perspective of interventional radiology, there has been a significant amount of controversy regarding the optimal therapeutic management of patients with gastric variceal bleeding.3–7 Whereas the West (United States and Europe) typically prefers decompression of the portal circulation as the primary treatment approach (i.e., transjugular intrahepatic portosystemic shunt [TIPS]), the East (Japan and South Korea) focuses on the gastric varices themselves, sclerosing them via the balloon-occluded retrograde transvenous obliteration (BRTO) procedure.3,8
Although this chapter will focus on the obliteration (sclerotherapy and/or embolotherapy) of gastric varices, given the complexity and variability of gastric varices, the preprocedural clinical management, endoscopic management, and the preprocedural imaging will also be discussed.
DEVICE/MATERIAL DESCRIPTION
Embolization Agents/Sclerosants
Many agents are available for use in variceal obliteration. Examples include ethanolamine oleate (EO, Oldamin; Grelan Pharmaceutical Co., Ltd., Tokyo, Japan), sodium tetradecyl sulfate (Sotradecol; AngioDynamics, Queensbury, New York), polidocanol foam (Polidocasklerol; ZERIA Pharmaceutical Co., Ltd., Tokyo, Japan), and N-butyl cyanoacrylate. EO is the original agent used for BRTO and remains the agent of choice in Asia.9–11 Five percent EO is the most common concentration used and is made with 10% EO and the same dose of contrast medium.9,11 EO causes hemolysis with the release of free hemoglobin which may result in renal tubular disturbances and acute renal failure. The administration of 4,000 units of haptoglobin (Green Cross, Osaka, Japan) intravenously will prevent renal insufficiency by binding circulating free hemoglobin. Additional reported complications include pulmonary edema, disseminated intravascular coagulation, and cardiogenic shock.10,12,13 Consequently, some authors recommend limiting EO to a volume of 40 mL per procedure. Routine use of this agent in the United States is markedly limited as haptoglobin is not approved by the U.S. Food and Drug Administration (FDA).
Sodium tetradecyl sulfate (STS) has been extensively used for sclerotherapy of superficial lower extremity varicose veins in both liquid and foam forms.14,15 Additional uses include treatment of venous malformations, male varicoceles, and pelvic congestion syndrome.16–19 There are many potential advantages of STS foam, including minimal administered doses, contact with variceal wall, density of material, and efficiency of endothelial damage. A recent study demonstrated a marked decrease in amount of STS given when comparing the liquid mixture (34.1 mL, range 10 to 65 mL) to the foam mixture (10 mL, range 1 to 20 mL). This lower dose may correspond to decreased systemic effects of hemolysis and renal failure without significantly affecting technical success. Further, STS foam is thought to provide improved contact with the variceal wall in comparison to liquid sclerosing agents. This has been demonstrated in the treatment of lower extremity varicose veins where foam sclerotherapy was found to be far more effective than liquid sclerotherapy by improved displacement of the blood volume along with providing a larger surface area of the sclerosant to contact the venous endothelium.15 In vivo studies have demonstrated pathologic damage to the endothelium to be rapid and occur within the first 2 minutes of contact, followed by intimal edema, progressive intimal separation from the tunica media, and microthrombi formation in the following 30 minutes.20 In addition, the foam sclerosants have a tendency to ascend immediately into nondependent gastric varices when compared with more dense liquid sclerosants.
Sotradecol is available in 1% and 3% concentrations. Although a recent study suggested that there is no added benefit in using 3% versus 1% concentration, 3% Sotradecol is often favored as it provides the highest dose possible to the varix.15 Although there is no standard method of foam preparation, it is recommended that 3% Sotradecol be mixed with gas (air or carbon dioxide [CO2]) along with Lipiodol for added visualization in the following ratio: 2 mL STS : 1 mL Lipiodol : 3 mL air/CO2.13 Although many observe that room air remains in foam solution longer than CO2, there remains concern that room air may potentially embolize to the lungs or the systemic circulation via a patent foramen ovale as it comes out of solution.21,22 Alternative sclerosant mixtures include foam EO (10 mL of 10% EO : 10 mL iodinated contrast medium : 20 mL of air) and foam polidocanol (2 mL of 3% polidocanol : 8 mL of air).
With regard to occlusion balloons, there are several options available in the United States and include the Coda (Cook Medical, Inc., Bloomington, Indiana), Python occlusion balloon (Applied Medical, Rancho Santa Margarita, California), Equalizer (Boston Scientific Corporation, Natick, Massachusetts), and flow-directed occlusion balloon catheters (Cook Medical, Inc., Bloomington, Indiana).
Complete details on inventory (sclerosants and catheters) are beyond the scope of this chapter; however, they are discussed in detail by Saad et al.,23 including specifications for balloon occlusion catheters.
TECHNIQUE
Procedural Steps
Access is usually achieved via the right femoral or internal jugular vein using standard angiographic technique and placement of a 6-Fr to 12-Fr sheath. More commonly, access is through a right femoral vein approach. However, some institutions use the jugular vein approach exclusively. Preprocedure imaging may aid in deciding which approach provides the best angle for selecting the shunt.
During a conventional BRTO, the gastrorenal shunt (GRS) is catheterized via the left renal vein using a balloon occlusion catheter (a compliant balloon mounted on a catheter). Balloon occlusion catheters with a reverse curve are available in Asia and provide easier and more stable access into the shunt. These catheters are not readily available in the United States, and it is suggested that stable access into the inferior vena cava (IVC) or distal renal vein be secured with an appropriately sized sheath (6-Fr to 12-Fr). The renal vein may be selected with a 5-Fr diagnostic catheter (Cobra; AngioDynamics, Queensbury, New York). The diagnostic catheter can then be exchanged for an angled-tip catheter, which may be used to select the shunt. Alternatively, a 5-Fr Simmons 2 catheter (AngioDynamics, Queensbury, New York) may be used to select the renal vein and withdrawn until the tip engages the shunt. A 0.035-in stiff guidewire (TAD II [Mallinckrodt Inc., St. Louis, Missouri] or Rosen [AngioDynamics, Queensbury, New York]) is advanced into the shunt as far as possible and the angled-tip catheter is exchanged for an occlusion balloon that is sized to occlude the communicating GRS of interest. The diameter of occlusion balloons ranges from 8.5 to 32 mm. Selection of the proper occlusion balloon would optimally occur at the time of preprocedure imaging (as detailed earlier). A new technique for selection of the shunt from the left renal vein via a femoral approach using a straight reinforced sheath is called the PRESS technique (Pullback RE-inforced Straight Sheath).24,25 The left renal vein is selected with a catheter (usually Cobra-shaped) and a 0.035-in Rosen wire is advanced into the left renal vein into the left renal hilum. The Cobra catheter is exchanged for a reinforced sheath. Pullback venography through the sheath as it is withdrawn over the anchoring Rosen wire is performed. The anchoring wire and the shape of the reinforced sheath allows the sheath to “scrape” the superior aspect of the left renal vein. The sheath will then “pop” into the shunt.24,25
Balloon occlusion venography may then be performed to define the type of varix/varices and the anatomy of the venous drainage. As will be discussed in greater detail in the section entitled “Preprocedure Imaging,” venous drainage patterns may be classified into type A, B, or C (Fig. 32.1). Type D drainage patterns lack a definable shunt and are unable to be treated via the BRTO procedure.5,26
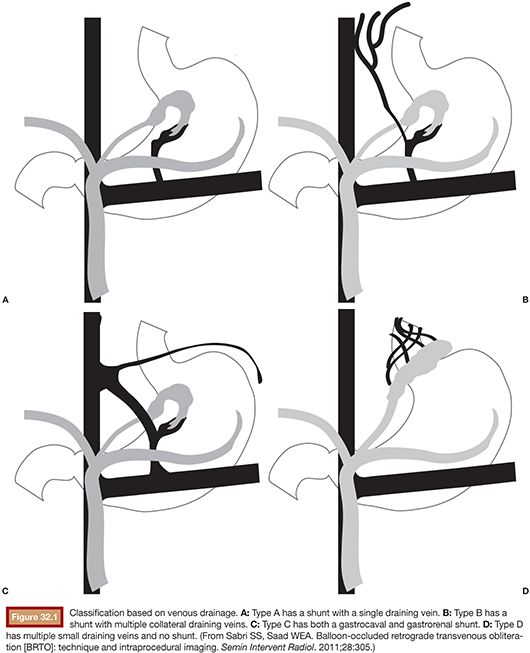
Following balloon occlusion venography, the sclerosant may be infused. The goal of sclerosant infusion is filling of the extent of the varix with the embolization end point of minimal filling of the afferent portal vein branch(s). Although the injection of the sclerosing agent can be performed with or without the use of a microcatheter, it is recommended that a microcatheter be advanced as deeply as possible into the varix and sclerosing agent administered through the microcatheter. This allows the advantage of selective delivery of the sclerosant into the varix while minimizing the amount of volume (optimizing distribution of the sclerosant) used as well as minimizing the risk of balloon rupture when the sclerosant comes in contact with the balloon. Full opacification of gastric varices may be prevented by leaking collateral veins such as the inferior phrenic or paravertebral veins. Occlusion with coils or Gelfoam pledgets (Pfizer, New York, New York) through the microcatheter can help occlude these veins, allowing concentration of the sclerosant at the varix and minimize nontarget efflux of sclerosant into the portal system or systemic vasculature.
Following infusion of chosen sclerosant, the occlusion balloon(s) remain inflated for a minimum of 4 hours and a maximum of 24 hours. Although the balloon(s) may be left in place overnight and deflated under fluoroscopy the following day, this will likely increase access site bleeding, infection rates, and patient inconvenience. Research suggests that there is no observable change in obliteration rate or complication rate when leaving inflated for 4 hours as opposed to 24 hours.13
CLINICAL APPLICATIONS
Preprocedure Evaluation and Management
Clinical Management
A patient presenting with underlying liver disease and upper GI bleeding should be appropriately evaluated and closely monitored. When the patient is clinically stable, upper endoscopy is typically performed for first-line diagnostic and therapeutic purposes.2 It is advised that aggressive volume resuscitation be avoided as variceal bleeding may be exacerbated by fluid overload. After identification of bleeding gastric varices, the management involves diagnostic and therapeutic considerations necessitating a multidisciplinary approach. A management protocol flowchart may be found in Figures 32.2 and 32.3. Although this will briefly be discussed in the following pages, a complete discussion regarding management of gastric varices is outside the scope of this chapter. The reader is referred to the current literature regarding indications and management of gastric varices.27
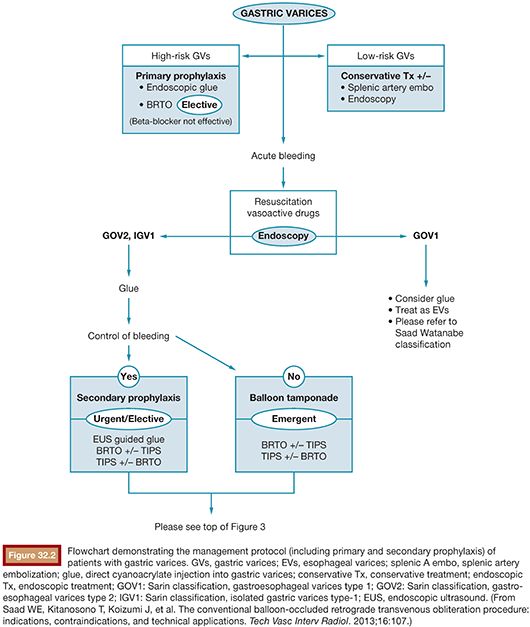
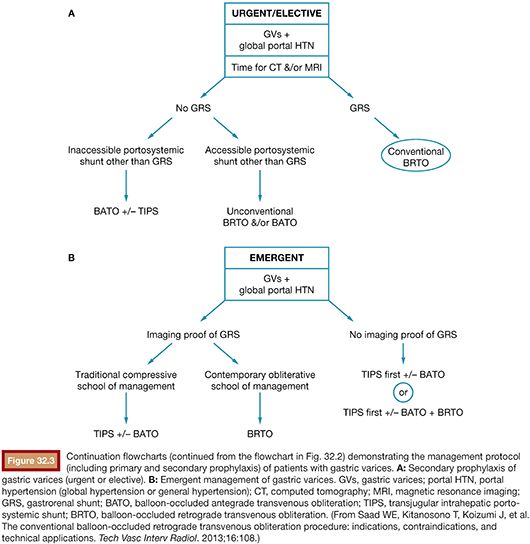
Endoscopic Evaluation and Treatment
As mentioned earlier, routine upper endoscopy is performed early in the clinical course of a patient presenting with melena or hematemesis. The endoscopic evaluation is essential for identification of which varices (if any) are bleeding in addition to identifying the types of underlying gastric varices. This is usually classified according to the Sarin endoscopic classification (Fig. 32.4). This classification helps to differentiate and triage the bleeding varices so that optimal therapy may be considered. Esophageal varices can often be controlled effectively by endoscopic-guided banding and sclerotherapy. Bleeding esophageal varices that cannot be controlled medically and endoscopically would warrant the creation of a TIPS. If gastric varices are identified on evaluation, then a different endoscopic and endovascular approach is warranted. In addition to etiologic identification, the stigmata of “high-risk gastric varices/impending bleeding” and/or stigmata of recent bleeding may be identified by endoscopy. If high-risk gastric varices with recent bleeding or actively bleeding cardiofundal varix is encountered in the course of that endoscopic evaluation, several clinical avenues may be considered. Conventional endoscopic therapy involves attempts at sclerosants and/or banding. However, studies have demonstrated relatively high failure rates for acute control and early rates of rebleeding.28 Recent comparative trials of sclerotherapy versus banding demonstrate high rebleeding rates of 30% to 45% in both groups, underscoring the significant morbidity associated with such endoscopic methods and enforcing the need for alternative treatment techniques.29 Cyanoacrylate injection may also be considered as a hemostatic agent for gastric variceal bleeding. Although its use in the United States has been limited, several trials have emerged as its use has been established as a primary means of achieving gastric varix obliteration. Although demonstrating improvement when compared with conventional techniques, cyanoacrylate maintains rebleeding rates from 15% to 27%.30,31 The elevated rebleeding rate of both conventional TIPS and endoscopic treatments have only served to increase interest in alternative treatment techniques such as BRTO.
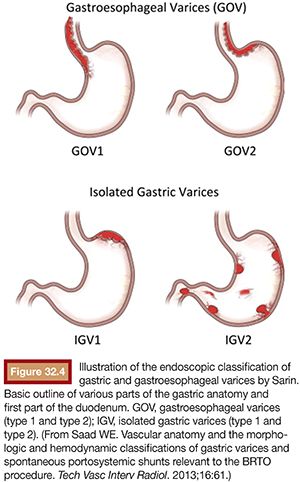
Preprocedure Imaging
Both three-phase contrast-enhanced computed tomography (CECT) and contrast-enhanced magnetic resonance angiography/venography (MRA/MRV) may be used for preprocedural evaluation and are equally effective at providing optimal visualization of the main portal vein and its tributaries.2 Advantages of CECT include availability, multiplanar reconstructions, and speed. When protocoling a CECT for BRTO, it is important to not give oral contrast as this may obscure visualization of the varices. Water may be used as it provides gastric distension and acts as a negative contrast agent for the contrast-enhanced gastric varices. In addition, it is recommended that images be acquired from the level of the midchest through the pelvis so as to fully evaluate the extent of existing portosystemic shunts.32 Advantages of MRA/MRV include lack of ionizing radiation, decreased nephrotoxicity, and evaluation of portal hemodynamics. It is important that images be acquired in the coronal plane and include dynamic postcontrast sequences. In addition, rapid sequences, such as the vastly undersampled isotropic projection reconstruction (VIPR) sequence (GE Healthcare, Milwaukee, Wisconsin), are promising in evaluating portal hemodynamics.2
Following image acquisition, many variables must be weighed when evaluating a patient for a possible BRTO and technical planning of the procedure. Several anatomic and hemodynamic factors to consider before performing a BRTO include splenic vein (SpV) patency, portal vein patency, and presence and size of the portosystemic shunt. In addition, for unconventional BRTO, one should note the presence and size of alternative portosystemic shunts and leading supradiaphragmatic and infradiaphragmatic systemic veins that can be seen traversing the diaphragm to reach the subphrenic gastric variceal complex. These include the left phrenic, left pericardiac vein, and the azygo-esophago venous/variceal axis (Fig. 32.5).2
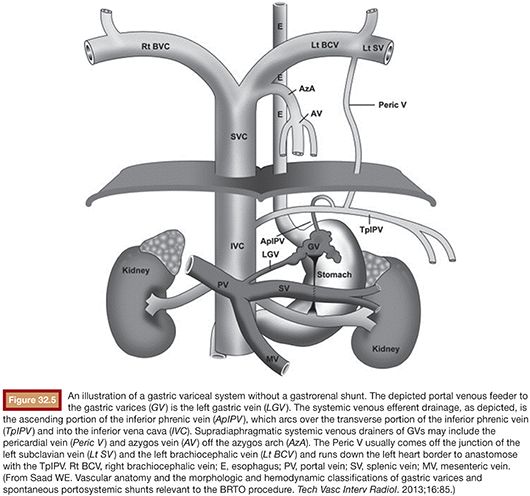
Assessing SpV patency allows differentiation between segmental (sentinel) and generalized (global) portal hypertension. This differentiation is critical for the management of gastric varices. Segmental portal hypertension involves thrombosis of the entirety of the SpV in the absence of portal vein thrombosis. Alternatively, segmental portal hypertension may be caused by acute or chronic pancreatitis with focal occlusion of the distal SpV. Generalized portal hypertension may be differentiated by classically showing secondary signs of a hepatic etiology (i.e., cirrhosis). Radiographic signs of portal hypertension include portal vein diameter greater than 14 mm, occasional portal vein thrombosis (partial or complete), cavernous transformation of the portal vein, and the presence of right-sided portosystemic collaterals (e.g., recanalized paraumbilical vein and esophageal varices along the left gastric and hemiazygous venous axis).2
The presence or absence of portal vein thrombosis, although a potential sequelae of a GRS, should also be noted in preprocedural imaging. GRSs and splenorenal shunts are portosystemic collaterals that have hepatofugal flow and may promote hepatofugal flow in the SpV and even the main portal vein. A complex set of hemodynamic variables will determine to the extent that the portal circulation is affected by a GRS, including size of the GRS, presence of TIPS, patency of the main portal vein, resistance of the sinusoidal bed, etc.26,33 As one would imagine, in the presence of main portal vein thrombosis, the GRS will act as a main outflow for the splenic and mesenteric veins. Occlusion of the GRS through a BRTO procedure would potentially cause mesenteric venous hypertension and mesenteric ischemia with possible thrombosis of the entire splanchnic portal venous circulation.2 However, the hemodynamics of the portal circulation is incompletely understood and the risk may be somewhat diminished in the presence of cavernous transformation of the portal vein. These risks should be carefully weighed against any potential benefits before a proposed BRTO.32
Last, for a conventional BRTO procedure, one must assess for the presence of a large infradiaphragmatic (i.e., left-sided) portosystemic collateral/shunt. These shunts include the splenorenal, gastrorenal (combine gastrosplenorenal), gastrocaval, gastrophrenic, and gastrogonadal portosystemic shunts.26 The most common shunt that is found and occluded during a conventional BRTO is a GRS, which provides portal venous outflow to the gastric varices in 90% of patients with gastric varices. The shunts may be classified according to the venous drainage pattern into A, B, C, or D (see Fig. 32.1).12,25 In addition, the diameter of the shunt is usually measured to plan for the BRTO and select for the appropriate equipment. The diameter of the shunt is typically measured at the distal shunt near the renal vein and is frequently the location where the interventional radiologist will attempt to occlude the shunt with the occlusive balloon for the BRTO procedure. It should be noted that the distal shunt may not correspond to the narrowest point of the shunt and it is for this reason that a thorough evaluation throughout the extent of the shunt is warranted. Further, attention must be paid to the presence of venous webs that may cause additional narrowing within the shunt and may aid in occlusion balloon catheter placement. On occasion, the size of a GRS on cross-sectional imaging will preclude a BRTO procedure; however, one may be attempted as computed tomography (CT) and magnetic resonance imaging (MRI) frequently underestimate the presence of webs. Only after cannulation and balloon occlusion can the true diameter and ability to safely occlude the GRS be tested with the balloon occlusion catheter available.
In the absence of a GRS or gastrocaval shunt (required for a conventional BRTO), alternative portosystemic collaterals/routes may be evaluated for the possibility of an unconventional BRTO. These routes may include gastrophrenic, pericardiogastric (left pericardiac vein), and azygous/hemiazygous to left gastric vein axis. Additionally, these routes may be present with a coexisting GRS, which may hinder the BRTO and allow escape of sclerosant into the systemic circulation.
Treatment Modifications According to Draining Venous Pattern
As detailed earlier and outlined in Figure 32.1, shunts may be classified according to the venous drainage pattern into A, B, C, or D.5 Treatment of gastric varices may require modification on the basis of the draining venous pattern.
Type A varices are contiguous with a single draining shunt. This is most commonly a GRS and less commonly a gastrocaval shunt draining through an enlarged inferior phrenic vein directly into the IVC. This type of drainage pattern is the easiest to treat given the absence of leaking collateral veins or additional shunts. Further, the entire varix can be visualized during balloon-occluded retrograde venography. As detailed earlier, the microcatheter can then be advanced through the balloon occlusion catheter as deep as possible into the varix and sclerosant administered to embolization end point of minimal filling of the afferent vein/portal vasculature (Fig. 32.6).34
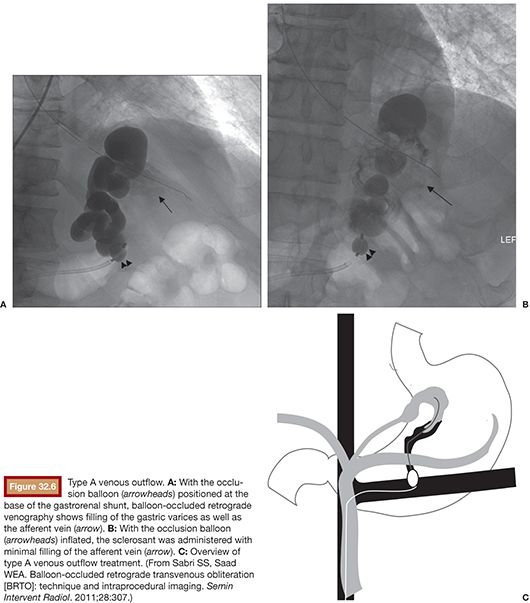
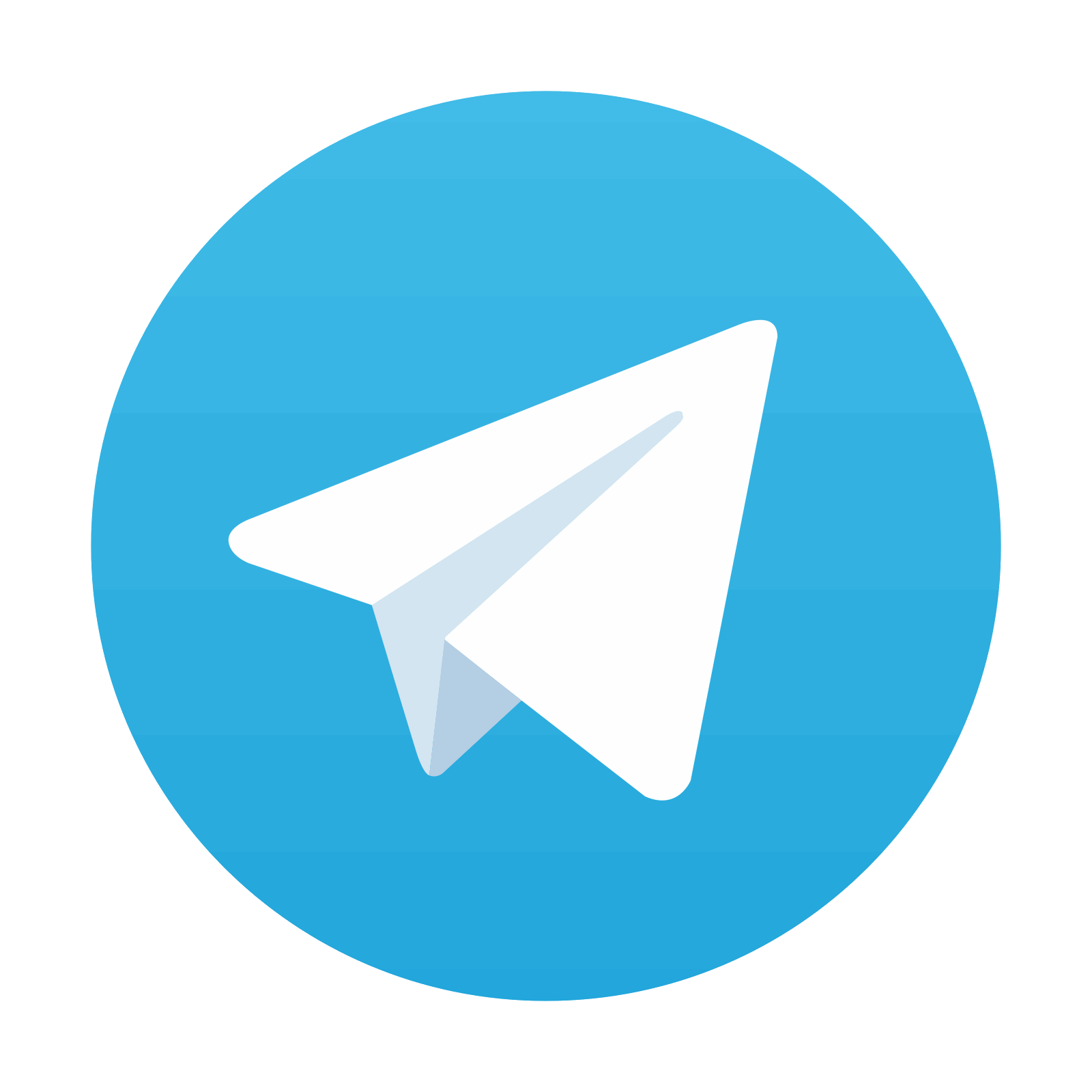
Stay updated, free articles. Join our Telegram channel

Full access? Get Clinical Tree
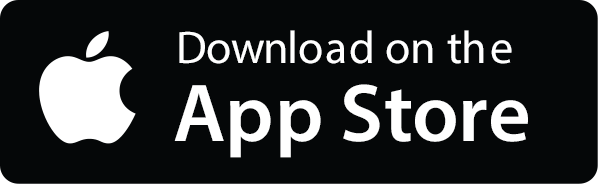
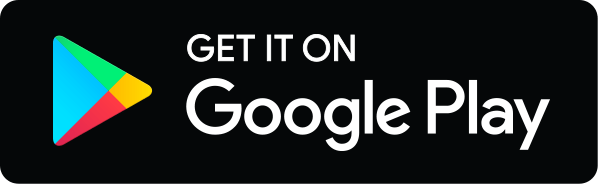