Fig. 3.1
Testicular cyst . The tissue distal to this testicular cyst (gridded area) is prone to more tissue heating because sound waves passing through the cyst are less attenuated than those passing through adjacent testicular parenchyma
Tissue heating of up to 1 °C may occur during diagnostic imaging at tissue/bone interfaces. Localized tissue heating seems to be safe up to about 2–5 °C [2]. In most diagnostic scanning applications, the amount of tissue heating is negligible. The beneficial effects of tissue heating are utilized in therapeutic ultrasound for joints and muscles. At high intensity levels (e.g., with high-intensity focused ultrasound (HIFU)), the biological effects of heating and cavitation are used to destroy tissue [3].
Standard gray-scale imaging generates the least tissue heating because the pulse duration is short and pulse-repetition frequencies (PRFs) are low. Color flow Doppler and power Doppler produce intermediate tissue heating. Spectral Doppler imaging is an unscanned mode which uses longer pulse durations and higher PRFs (to avoid artifacts such as aliasing) and therefore has an increased potential for heating. Spectral Doppler also involves longer duration times as individual vessels are interrogated in a fixed scanning position (Fig. 3.2) [4]. Tissue heating associated with routine diagnostic ultrasound is not known to produce tissue damage or to cause denaturation of proteins or to have teratogenic effects as long as scanning times are not prolonged and acoustic output restrictions are observed [5].
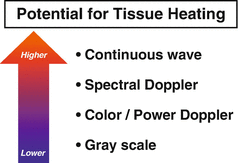
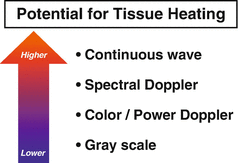
Fig. 3.2
Potential for tissue heating. Relative likelihood of tissue heating by mode of ultrasound is based in part on pulse-repetition frequency and duration of tissue exposure
Mechanical Effects
Ultrasound energy generates an acoustic field in tissue. The acoustic field generates mechanical forces which affect tissue at the microscopic and macroscopic levels. Pressure exerted by the field creates torque and induces motion called streaming. These forces may potentially damage cells or tissues and also contribute secondarily to tissue heating.
One phenomenon associated with acoustical fields , cavitation , deserves special attention since it has been shown to cause tissue damage in animal models [1]. Cavitation occurs when gas bubbles within tissue begin to first oscillate or vibrate in response to ultrasound and then to collapse. This collapse causes a violent movement of individual adjacent particles within the tissue which, in turn, causes tissue damage. This damage may take the form of ruptured cells and blood vessels or may cause chromosomal (ultrastructural) damage. In addition, the heat generated by the rapid collapse of gas bubbles may result in the formation of toxic chemical by-products (Fig. 3.3) [6].
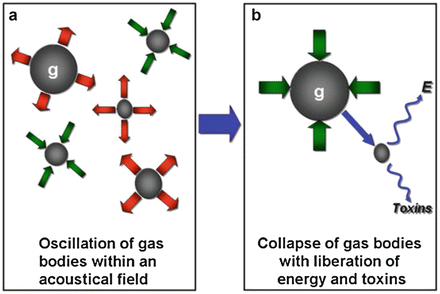
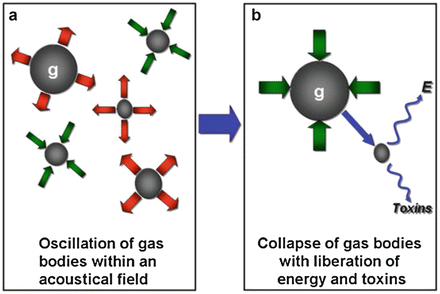
Fig. 3.3
Cavitation . (a) The oscillation and collapse of gas bodies (g) is cavitation. (b) The result of the collapse of gas bodies is the release of energy (E) and chemical toxins which may cause tissue damage
The detrimental mechanical effects of acoustic fields are most likely to occur at gas/fluid interfaces. Thus, cavitation effects (such as petechia formation) would most likely be seen in the lung or bowel. The threshold for cavitation is approximately 1 kW/cm2 (far higher than the average intensity of 100 mW/cm2 generated by most clinical scanners) [6].
Patient Safety
For most urologic applications, ultrasound produces energies in human tissues which are within recognized safe limits. The single most important factor in minimizing potential adverse effects of ultrasound is the well-informed ultrasound operator [7].
The proper selection of acoustic output, transducer frequency, and mode of ultrasound for a specific indication will mitigate risks. Expeditious and efficient scanning technique is critical to limiting overall exposure. To assist the sonographer in monitoring the bioeffects of ultrasound, the ultrasound community has adopted the output display standard (ODS) [8]. Two values are typically displayed, the mechanical index (MI) and the thermal index ( TI ) (Fig. 3.4). These indices are calculated estimates of the potential for bioeffects of ultrasound based on the mode of ultrasound being used, frequency, power output, and time of insonation. The MI and TI are typically displayed on the monitor during ultrasound examinations and all practitioners should be familiar with the location. It is important to understand that these indices are not safety limits and are not direct measurements .
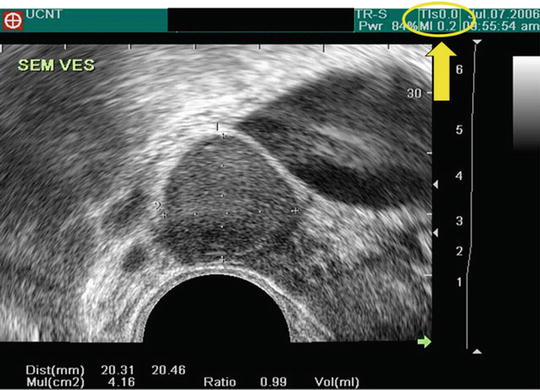
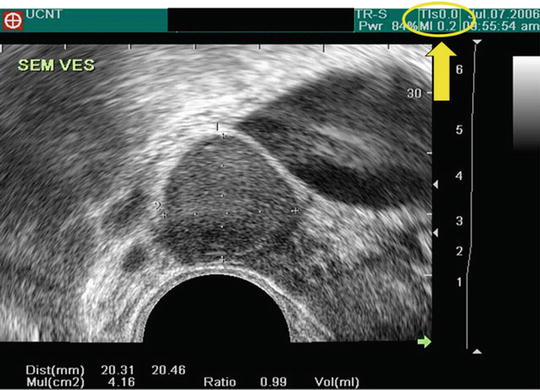
Fig. 3.4
Mechanical and thermal index. The MI and TI (yellow cycle) are calculated estimates of the potential for cavitation and tissue heating respectively, based on study parameters and time of study. These are not direct measurements of either heating or pressures in the acoustical field
Mechanical Index
The MI indicates the probability that cavitation will occur. For tissues not containing stabilized gas bodies (lung and intestine), the risk of cavitation is low as long as the MI is ≤0.7. For structures adjacent to lung or intestine, scanning time should be limited if the MI exceeds 0.4 (Fig. 3.5).
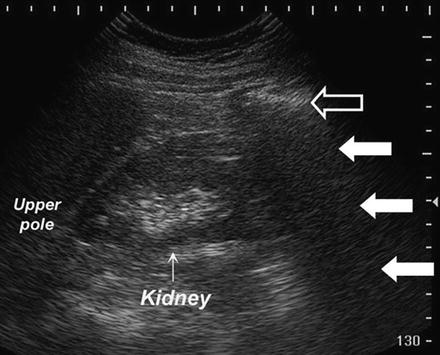
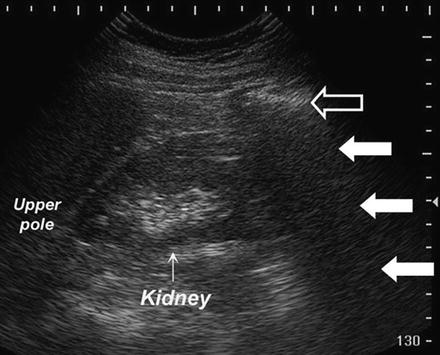
Fig. 3.5
The risk for cavitation is higher in tissues which are in proximity to gas-containing bodies such as the bowel. Here, bowel gas (open arrow) and the resultant distal acoustic shadowing (white arrows) obscures the lower pole of the right kidney (arrow)
Thermal Index
The TI indicates the probability that tissue temperature within the sonographic field will be increased by 1 °C. The precise consequences of tissue heating are not completely understood, but even tissue temperature elevations of up to 6 °C are not likely to be dangerous unless exposure time exceeds 60 s [2].
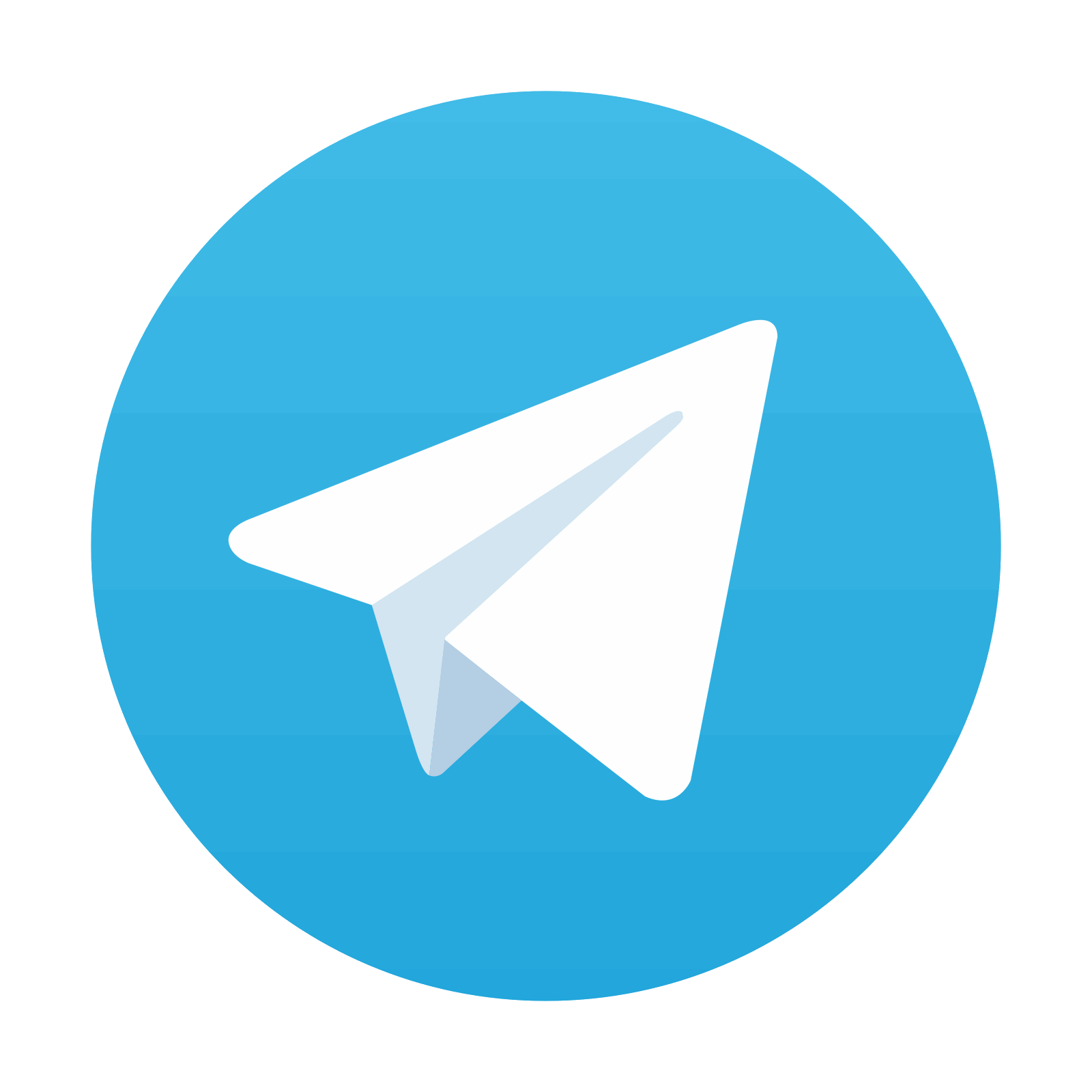
Stay updated, free articles. Join our Telegram channel

Full access? Get Clinical Tree
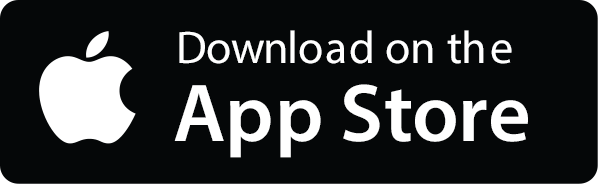
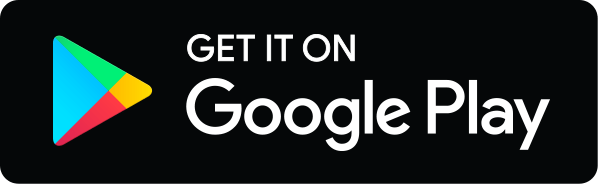