Fig. 1
Biocontinuum of adverse early and late bladder effects are shown (with permission from Rubin and Casarett 1968)
2 Anatomy and Histology
2.1 Normal Bladder Anatomy
The bladder consists of two main parts: (1) the body where urine is collected, and (2) the bladder neck, which is a 2–3 cm long, funnel-shaped extension of the body that traverses the urogenital diaphragm and connects with the urethra. The trigone region sits at the bladder base between the inlet of the two ureters and the urethral opening. The wall of the bladder is mainly composed of smooth muscle, called the detrusor muscle, and easily distends to allow passive bladder filling. At the neck of the bladder, the detrusor muscle fibers form the involuntary internal sphincter. These muscle fibers are in continuity with the muscle fibers of the wall of the prostatic urethra in males and the wall of the urethra in females. The muscular contraction of the bladder wall results in expulsion of urine through the urethra. Continence is regulated by the internal and external urinary sphincters, which respond to spinal signaling reflexes regulated by cortical control.
2.1.1 Anatomic Relationships
When empty, the urinary bladder sits in the true pelvis, posterior and slightly superior to the pubic symphysis, separated from the bone by the retropubic space. It rests on the pelvic floor and is bounded by the peritoneum superiorly. The uterus and ileum may also be superior to the bladder in females, and the colon and ileum may be superior in males. Condensations of the peritoneum provide loose attachments of the bladder to the lateral pelvic wall and anterior abdominal wall, including the median umbilical ligament, which attaches the bladder apex (dome) to the umbilicus. The bladder bed is formed by the pubic bones and obturator internus muscles anteriorly, the levator ani laterally, and posteriorly the vas deferentia, seminal vesicles, and rectum in males and the vagina and uterus in females (Fig. 2).
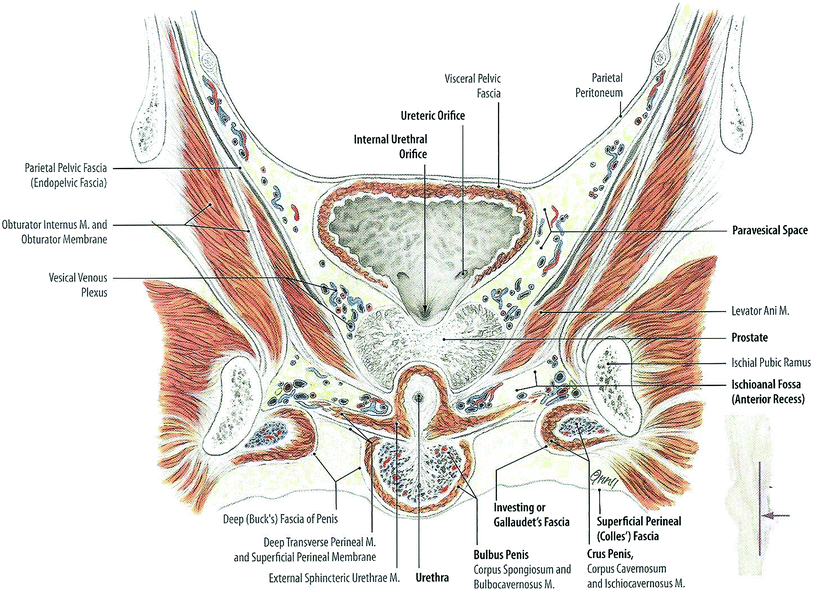
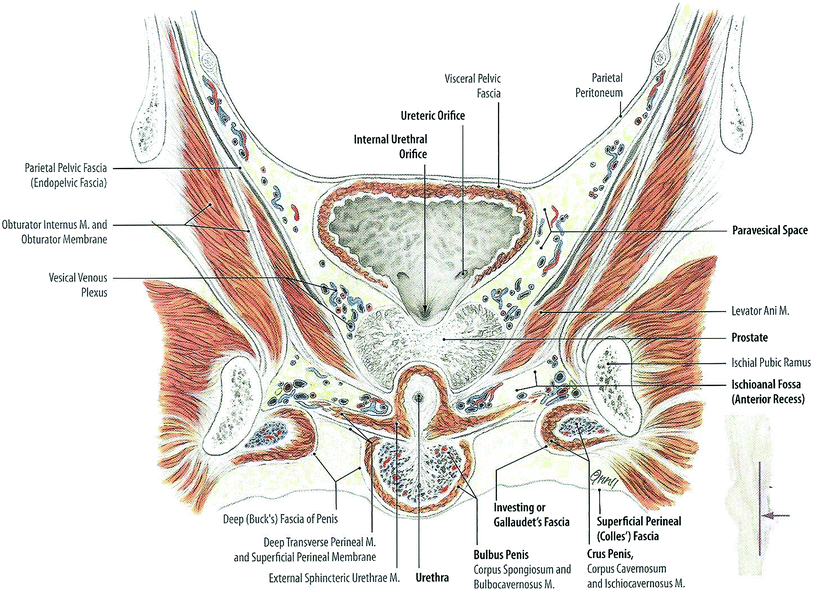
Fig. 2
Anatomy of the bladder (with permission from Tillman 2007)
2.1.2 Bladder Structure
The bladder is a flexible organ; it is rounded when it contains urine, and pyramid-shaped when empty. The empty pyramid-shaped bladder has four surfaces: the superior surface is bounded by the peritoneum; the two inferolateral surfaces are in contact with the fascia covering the levator ani; and the posterior–inferior surface is the fundus, which forms the base of the pyramid, and is adjacent to the anterior wall of the vagina in females and the anterior wall of the rectum in males. The apex (or dome) is the anterior portion of the bladder, which is located near the superior edge of the pubic bone, and ends as a fibrous cord, the median umbilical ligament, which is derived from the urachus, the original connection between the bladder and the allantois. The neck of the bladder is formed by the convergence of the fundus and the inferolateral surfaces, and is the most inferior part of the bladder. The neck of the bladder is continuous with the urethra, and is typically held in place by the pubovesical ligaments in females and the puboprostatic ligaments in males. The rest of the bladder is not fixed by connective tissue, and can be highly mobile. The ureters enter the bladder wall inferomedially on opposite sides at an oblique angle. The two ureteric orifices and the internal urethral orifice at the neck of the bladder form the three points of the bladder trigone.
2.1.3 Normal Bladder Innervation
Continence is regulated by the internal and external sphincters. The internal sphincter is derived from smooth muscle fibers, which are interconnected with the smooth muscle of the bladder wall. Sympathetic innervation from T11 to L2 maintains the smooth muscle tone of the internal sphincter and the trigone, which allows for involuntary continence. These sympathetic nerves arrive at the bladder via lumbar and pelvic splanchnic nerves. The external urinary sphincter is composed of voluntary, striated muscles surrounding the middle third of the urethra in females and the tip of the prostatic urethra in males, with contribution from the muscles of the pelvic floor such as the levator ani. Motor innervation to the external sphincter and pelvic floor muscles arises between the S2 and S3 levels and is delivered via the pudendal nerves.
Motor control of the detrusor muscles and inhibition of the internal sphincter occurs via parasympathetic fibers, which arise from the gray matter of the spinal cord (S2–S3) and travel to the bladder via the sacral and pelvic splanchnic nerves. Sensations of stretch and bladder fullness are carried along the pelvic parasympathetic nerves, while pain, touch, and temperature sensory innervation travel via sympathetic pathways to the T11–L2 spinal cord level. These fibers transmit sensations of pain from over-distention or inflammation.
2.1.4 Bladder Vasculature
The bladder has a rich vasculature, and the major blood supply to the bladder is derived from branches of the internal iliac arteries. The anterior–superior portions of the bladder are supplied by the superior vesical arteries, while the fundus is supplied by the inferior vesical arteries in males and the vaginal arteries in females. Other minor blood suppliers include the obturator and inferior gluteal arteries. Venous drainage of the bladder occurs through the vesical venous plexus, the prostatic venous plexus in males, and the vaginal venous plexus in women. The vesical venous plexus predominantly drains to the internal iliac veins via the inferior vesical veins, but also may drain to the vertebral venous plexus via the sacral veins.
2.2 Histology and Functional Subunit
The surface of the bladder is comprised of an epithelial cell layer overlaying a muscle layer. The epithelial lining is composed of 3–7 layers of transitional cells, which rests on top of a basement membrane. The epithelial lining is continually repopulated by rapidly dividing cells in the basal epithelium. The superficial luminal cells are large, umbrella-like, and connected by tight junctions, which contributes to the impermeability of the epithelial layer. Overlying the epithelial lining is a sulfated polysaccharide layer (glycosaminoglycan), which prevents the attachment of small molecules, bacteria, protein, and ions found in urine. Radiation or inflammation may damage this superficial polysaccharide layer or disrupt the tight junctions of the luminal urothelium cells, resulting in the introduction of bacteria, ions, or small molecules to the underlying epithelium and leading to infection, cystitis, or other toxicity (Fig. 3).
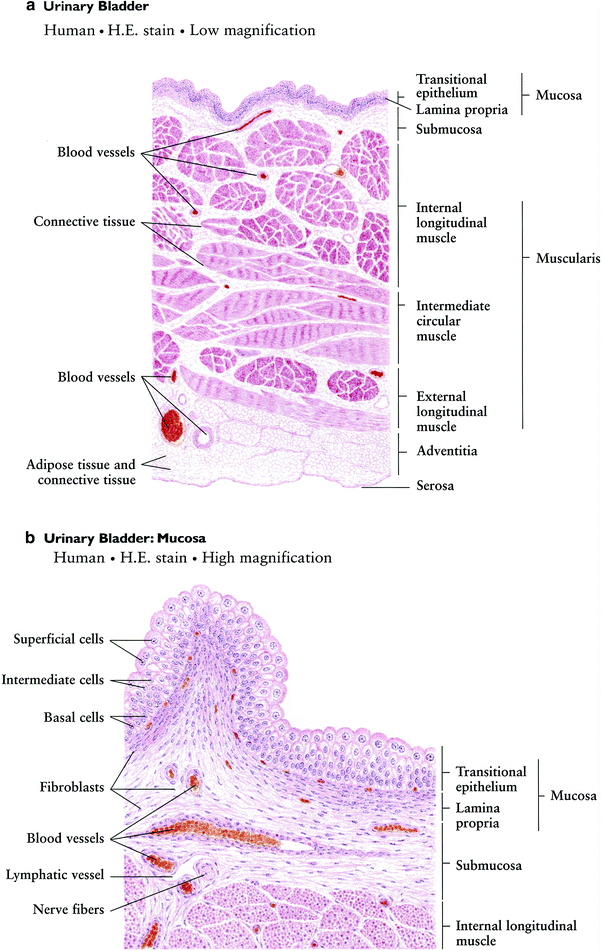
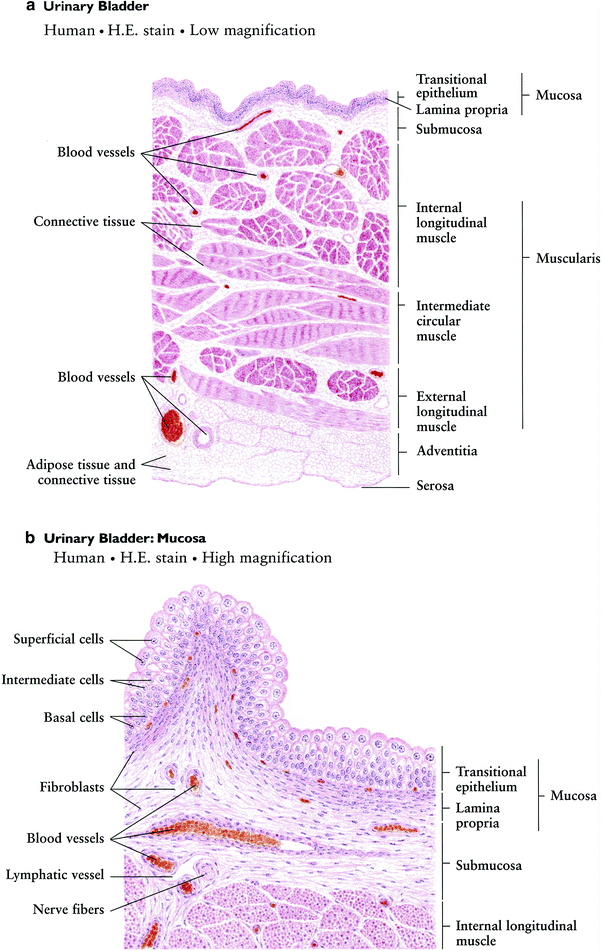
Fig. 3
Histology of the bladder: a low magnification, b high magnification (with permission from Zhang 1999)
Below the extracellular matrix of the basement membrane is the lamina propria, which is composed of loose connective tissue and a few smooth muscle fibers, and permits a significant amount of stretching of the mucosa. Beneath the lamina propria, lies the detrusor muscle layer. The detrusor smooth muscle is arranged in widely spaced bundles, infiltrated by a rich network of arterioles, venules, and small parasympathetic and sympathetic nerves. In the body of the bladder, the smooth muscle fibers are oriented in different directions and interweaving, but near the neck of the bladder, the smooth muscle bundles coalesce into three distinct layers: inner, outer, and medial. The inner and outer layers are oriented longitudinally, whereas the medial layer is oriented circularly. The inner layer extends into the urethra to form the longitudinal inner urethral muscle layer, while the medial layer condenses to form a dense circle that is most prominent anteriorly. The outer layer extends down into the urethra in females and to the end of the prostatic urethra in males, and constitutes the involuntary internal sphincter.
3 Physiology, Biology, and Pathophysiology
3.1 Physiology
No specific functional unit has been defined for the bladder. Different portions of the bladder such as the body and the neck have varying composition and architectural arrangements of smooth muscle, vessels, and nerves. However, all the structures listed in the prior section including the superficial polysaccharide layer, epithelium, bladder mucosa, detrusor muscle (muscularis propria), small blood vessels, and small nerves branches may all be considered to form part of a functional subunit (Fig. 4a) as described by Marks et al. (1995).
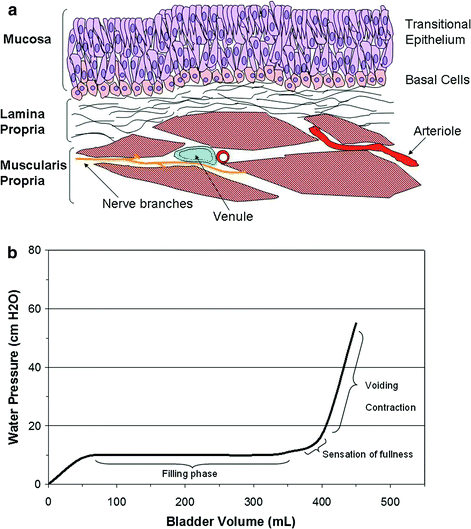
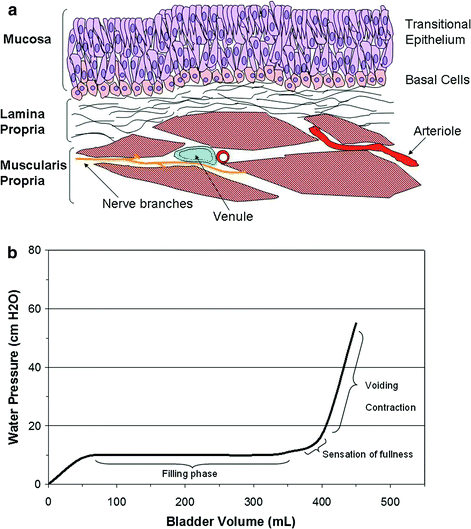
Fig. 4
a The functional subunit of the urinary bladder is illustrated. Normal function depends upon the successful interaction of the bladder wall mucosa, consisting of several layers of urothelium and a basement membrane, the lamina propria, detrusor smooth muscle layers, and supporting neurovascular structures. (Adapted from Marks et al. 1995). b A normal cystometrogram study showing a large capacity, compliant bladder. Little change in bladder pressure is produced with filling until capacity is reached. The pressure is high during voiding contraction as shown. (Adapted from Marks et al. 1995)
The bladder is a compliant structure that acts as a storage receptacle for urine, but also a dynamic muscular organ that allows for voluntary release of urine. Urine storage occurs by passive, compliant filling of the bladder with urine from the ureters. Urine is maintained in the bladder by an intact voluntary external sphincter, involuntary internal sphincter, and a ureteral antireflux mechanism, until voiding occurs. The antireflux mechanism, which is created by the oblique angle of entry of the ureters and basal detrusor muscle tone, ensures forward flow during micturition.
The normal bladder capacity is 400–500 ml in normal adults, although the bladder may expand significantly particularly in patients with atonic bladders. Table 1 describes the physiological parameters of the normal bladder. The normal bladder maintains low pressure (5–10 cm H2O) filling until bladder capacity is reached, due to the compliance of the bladder wall (Fig. 4b). When the volume of urine in the bladder reaches beyond 300–400 ml, the intravesicular pressure rises rapidly. Once the bladder is filled to capacity, sensory stretch receptors in the bladder wall trigger the micturition reflex and lead to contraction of the detrusor muscle and relaxation of the internal sphincter. The spinal cord mediated micturition reflex is autonomic, but can be modulated by voluntary control from the cortex and inhibitory signals from the pons. Additionally, voluntary motor control of the external sphincter also allows control over micturition. Voluntary urination is initiated by voluntary contraction of the abdominal muscles which increases intra-abdominal pressure and forces more urine into the bladder neck, leading to increased distention of the bladder neck, stimulation of the stretch receptors and triggers the micturition reflex. Once the spinal reflex is activated, a positive feedback loop occurs with detrusor muscle contractions raising intravesical pressure and activating stretch receptors, which rapidly increases the pressure in the bladder by 20–40 cm H2O. Micturition then occurs with passive opening of the bladder neck, involuntary relaxation of the internal sphincter, and voluntary relaxation of the external sphincter. Once micturition occurs, the neural components of the micturition reflex enter an inhibited state for a period of minutes to an hour, which prevents further activation of the reflex. In a normal bladder, nearly all the urine will be emptied with approximately 5–10 ml left in the bladder.
Table 1
Physiological parameters of the normal bladder
Functional parameter | |
---|---|
Bladder capacity | 400–500 mL |
Bladder wall volume | 50–70 cm3 |
Resting bladder pressure | 5–10 cm H2O |
Contraction pressure | 20–40 cm H2O |
Functional urethral length | 6–7 cm (men) |
4 cm (women) | |
Urine flow rate | 20–25 mL/s (men) |
25–30 mL/s (women) |
3.2 Biology and Pathophysiology of Radiation-Induced Bladder Injury
Considering Marks’ proposed functional subunit of the bladder, possible targets of radiation-induced damage include the epithelial cell layer, detrusor muscles, nerves, and microvasculature. The bladder responds to radiation injury and other stressors with inflammation, tissue reorganization, and cellular proliferation. The specific molecular mechanisms underlying the bladder response to injury have not been elucidated. Our limited understanding of the pathophysiology of radiation-induced bladder injury is derived largely from animal studies. The largest series of animal studies on the bladder were published by Stewart et al. at the Gray Laboratories in the 1980s (Stewart 1985, 1986; Stewart et al. 1978, 1980, 1981, 1984, 1990, 1991). These studies examined the histological changes and assessed bladder function via a urinary frequency assay in mice treated with radiation.
3.2.1 Urothelium
The urothelium’s sensitivity to radiation has been observed in both mouse and rat models, and in the histology of bladder biopsy specimens from patient’s treated with radiation (Stewart 1986; Antonakopoulos et al. 1982, 1984). Early changes and signs of damage including nuclear irregularity, cellular edema, and increased lysosomes and autophagic vacuoles in intermediate and basal urothelial cells, are seen approximately 3 months after irradiation. By 6–12 months, there is a dose-dependent increase in cellular proliferation and normal differentiation may be lost in many areas (Fig. 5a). Stewart looked at the proliferative response of mouse urothelium, using a thymidine incorporation assay and functional changes in the mouse bladder using urinary frequency and hematuria as endpoints, after treatment with radiation, cyclophosphamide, or both (Stewart 1985; Edrees et al. 1988). While cyclophosphamide-induced early increase in urinary frequency, hematuria, denuding of the urothelial layer, and rapid epithelial proliferation within 1 week of treatment, the effects of radiation alone were not seen until 4 months after treatment. These data suggest that early epithelial injury and turnover are not immediate precursors to late radiation-induced bladder toxicity. Edrees et al. demonstrated that there appears to be a synergistic effect between cyclophosphamide and radiation, since the combination led to early radiation dose-dependent damage within a week of treatment, and also more severe late radiation damage at 9–12 months compared to radiation alone (Edrees et al. 1988).
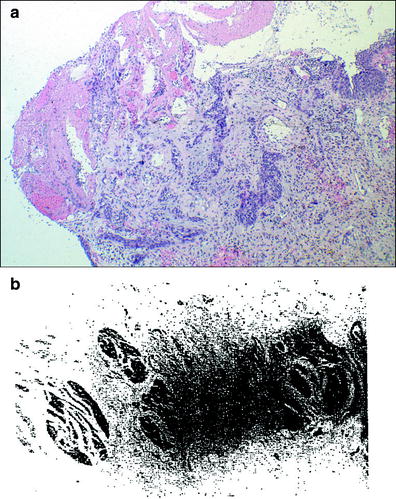
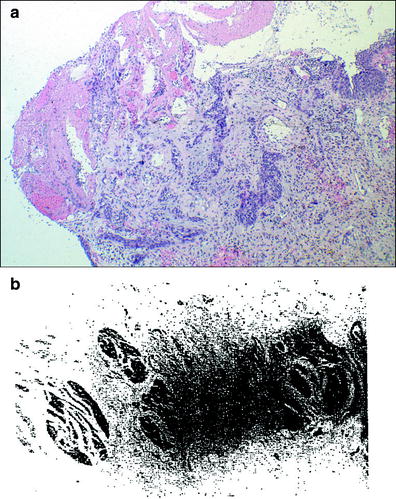
Fig. 5
a Histological image of radiation-induced bladder injury. Florid epithelial proliferation and reactive changes in the lamina propria in case of radiation cystitis. Images courtesy of Dr. Robert H. Young, Department of Pathology, Massachusetts General Hospital. b Photomicrograph of the muscularis propria of an irradiated bladder shows severe fibrosis of the bladder wall, which had a urine capacity of about 40–50 ml. This bladder was irradiated for a primary carcinoma that was eliminated by therapy. The patients subsequently died of metastases that were not apparent at the time of therapy (with permission from Fajardo 2001)
Disruption of the bladder epithelium may contribute to the development of early symptoms seen with bladder radiation. Radiation injury of the epithelium disrupts the normal tight junctions between urothelial cells and the protective polysaccharide layer, which allows hypertonic urine, toxins, ions, and bacteria to penetrate into the underlying tissue. The resultant damage and inflammation may lead to the onset of early radiation-induced symptoms including irritative voiding symptoms such as urinary frequency and urgency (Marks et al. 1995).
3.2.2 Microvasculature and Endothelium
Injury to the vascular endothelium also occurs early after radiation in mouse models. Endothelial cell edema is observed 3 months after radiation, and endothelial proliferation is identified ≥6 months after radiation, but does not occur to the same degree as seen in the urothelium (Stewart 1985). Further cell damage, including endothelial cell edema and necrosis, and perivascular fibrosis, occurs 6–12 months after radiation, and these findings have also been observed in human histological specimens (Antonakopoulos et al. 1984). Subsequently, vascular occlusion and focal bladder ischemia may occur months to years after radiation.
3.2.3 Detrusor Smooth Muscle
The smooth muscle can also be effected, and cellular edema occurs early and can be followed by cellular injury. Subsequent late tissue remodeling includes fibroblast proliferation, collagen disposition, and progressive fibrosis, which can lead to decreased bladder compliance, functional restriction of bladder capacity, and bladder contracture (Antonakopoulos et al. 1984). Extensive fibrosis can disrupt/replace the normal musculature. Experiments by Lundbeck et al. using cystometry to measure bladder pressure changes during bladder filling after radiation treatment in mice showed an acute dose-dependent decrease in bladder capacity by 14 days (Lundbeck et al. 1989a, b). The decreased compliance resolved over 14–28 days. Irreversible, late reduction in bladder compliance occurred at variable time intervals after treatment, and is consistent with clinical contracted bladder syndrome. Late loss of compliance was more commonly seen in animals treated with doses higher than 20 Gy (Stewart et al. 1991), and occurred after a latent period ranging between 35 and 401 days (Bentzen et al. 1992) (Fig. 5b).
3.2.4 Neural Tissue
While the bladder wall is richly innervated with sympathetic and parasympathetic nerves, the role of radiation-induced nerve injury in producing early and late symptoms has not been systematically studied and remains unclear. In some studies, early changes in bladder function after radiation do not appear to be caused by nerve injury (Stewart 1985, 1986; Antonakopoulos et al. 1982). Nevertheless, in patients treated with combined modality therapy, surgical procedures such as radical prostatectomy or radical hysterectomy may result in mechanical injury to the innervation of the bladder, leading to a spectrum of clinical outcomes from mild dysfunction to atonic bladders. It remains unclear whether adjuvant radiation in these settings can exacerbate the underlying mechanical nerve injuries. The bladder wall does have stretch and pain receptors, and radiation-induced injury may lead to stimulation of these sensory nerves and result in pain, spasm, and incontinence.
3.2.5 Early Versus Late Functional Complications
Stewart et al. examined functional outcomes sequentially from 1 to 53 weeks after radiation with endpoints of urinary frequency and cystometric measurement of bladder volume (Stewart et al. 1991). Early signs of damage, including increase in urinary frequency and decrease in bladder volume, occurred 1–3 weeks after radiation, and typically lasted for less than 1 week. Late toxicity developed 16–40 weeks after fractionated treatment with total doses greater than or equal to 20 Gy, and was typically irreversible. Microscopically, the early, transient damage was not associated with changes in the epithelium or muscle layers of the bladder, but the irreversible late damage was accompanied by epithelial denudation, focal hyperplasia, fibrosis, and ulceration.
The exact mechanisms that cause the symptoms of early and transient bladder radiation-induced injury are unknown. As discussed above, disruption of the epithelial cell layer, tight junctions, and polysaccharide layer may result in permeation of urinary toxins into the bladder wall and subsequent tissue damage and inflammation (Marks et al. 1995). However, other investigators have proposed that smooth muscle damage as the underlying cause for early bladder toxicity, since mouse models have shown early smooth muscle cell edema and loss of bladder volume (Antonakopoulos et al. 1984).
Late injury is manifested mainly as bladder wall fibrosis, loss of bladder wall compliance and contraction. The existing evidence to date points toward vascular ischemia as the underlying pathophysiology behind these changes, and thus, the vascular endothelial cell has been proposed as the actual target for late radiation-induced bladder injury. As discussed above, endothelial cell injury and remodeling occur months to many years following radiation therapy, while acute epithelial cell injuries do not appear to be correlated with the development of late toxicity (Stewart 1985, 1986). Vascular endothelial hyperplasia, perivascular fibrosis, and vascular occlusion occur months to years after radiation therapy, leading to bladder wall ischemia, remodeling, fibrosis, degeneration, and necrosis, which in turn result in clinical bladder dysfunction, loss of compliance, and contracture.
3.2.6 Fractionation
There is evidence in animal models that suggest large fraction size is associated with higher complication rates (Stewart et al. 1981, 1984). Stewart et al. examined urinary frequency and bladder capacity in mice 10–14 months after irradiation to a range of doses using various fractionation regimens (1, 2, 5, 10, or 20 fractions), and observed increased late complications with high dose hypofractionation (>8 Gy). Based on these experiments, the authors estimated an α/β ratio in the range of 5–10 Gy, which is relatively higher than most other normal, slow dividing tissues.
3.2.7 Secondary Malignancy
Animal models have correlated bladder irradiation with secondary malignancies of the bladder. The development of transitional cell carcinomas in 10 of 20 F344 rats, 20 months after bladder radiation has been previously described (Antonakopoulos et al. 1982).
3.2.8 Reirradiation
Mouse models have also shown that retreatment of a previously radiated bladder (20–60 % tolerance dose with initial treatment) resulted in rapid development of functional damage within 2 weeks as manifested by increased urinary frequency and decreased compliance (Stewart et al. 1990). Reirradiation late bladder damage was inversely related to the dose given in the first treatment, but was independent of the interval between treatments. This suggests that there is minimal “slow repair.”
3.3 Recovery/Regeneration
As discussed above, signs of early bladder damage including increase in urinary frequency and decrease in bladder volume occurred 1–3 weeks after radiation in mouse models, and typically lasted for less than 1 week (Stewart et al. 1991). Some investigators have found increased mitotic activity in epithelial cells after radiation treatment (Schreiber et al. 1969). Stewart et al. demonstrated that the bladder has the capacity for sublethal damage repair during fractionated radiation. Mice treated with fractionated radiation experienced bladder damage as manifested by increased urinary frequency and decreased volume, but sublethal repair occurred within 24 h and was greater than repair observed in mouse skin (Stewart et al. 1981). These data suggest that early bladder insults may be reparable and early bladder toxicity self-resolves as also seen clinically in patients treated with radiation to the bladder. After resolution of the symptoms of early bladder injury, there is a variable latent period lasting months to years before the development of late bladder injury. However, the connection between repair of early injury and development of late toxicity remains unclear. Late bladder wall fibrosis, loss of compliance, and contraction are typically irreversible.
4 Clinical Syndromes (Endpoints)
The response of the bladder to radiation therapy can be divided into acute reactions that occur within 3 months of radiation, subacute reactions that occur 3–6 months after radiation, and late reactions that occur >6 months after radiation. As described above, acute symptoms such as hematuria or cystitis due to either radiation or chemotherapy may be secondary to reparable damage of the surface epithelium and smooth muscle of the bladder. These symptoms typically resolve spontaneously. Subacute effects often remain undetected. Long-term complications from radiation include fibrosis of the muscular wall of the bladder, with a resultant decrease in bladder capacity and compliance, and in severe cases, bladder contraction. Late hematuria may occur due to bladder mucosal telangiectasias, but may also be due to secondary bladder malignancy. LENT SOMA tables generated by RTOG/EORTC provide an overview and basis for grading toxicity (Tables 2 and 3).
Table 2
The subjective, objective and management components of the SOMA system for bladder
Grade 1 | Grade 2 | Grade 3 | Grade 4 | |
---|---|---|---|---|
Subjective | ||||
Dysuria | Occasional and minimal | Intermittent and tolerable | Persistent and intense | Refractory and excruciating |
Frequency | 3–4 h intervals | 2–3 h intervals | 1–2 h intervals | Hourly |
Hematuria | Occasional | Intermittent | Persistent with clot | Refractory |
Incontinence | <weekly episodes | <daily episodes | ≤2 pads/undergarments/day | Refractory |
Decreased stream | Occasionally weak | Intermittent | Persistent but incomplete obstruction | Complete obstruction |
Objective | ||||
Hematuria | Microscopic, normal hemoglobin | Intermittent macroscopic, <10 % decrease in hemoglobin | Persistent macroscopic, 10–20 % decrease in hemoglobin | Refractory, >20 % decrease in hemoglobin |
Endoscopy | Patchy atrophy or Telangiectasia without bleeding | Confluent atrophy or Telangiectasia with gross bleeding | Ulcerations into muscle | Perforation, fistula |
Maximum volume | >300–400 cc | >200–300 cc | >100–200 cc | <100 cc |
Residual volume | 25 cc | >25–100 cc | >100 cc | |
Management | ||||
Dysuria | Occasional non-narcotic | Regular non-narcotic | Regular narcotic | Surgical intervention |
Frequency | Alkalization | Occasional antispasmodic | Regular narcotic | Cystectomy |
Hematuria/Telangiectasia | Iron therapy | Occasional transfusion or single cauterization | Frequent transfusion or coagulation | Surgical intervention |
Incontinence | Occasional use of incontinence pads | Intermittent use of incontinence pads | Regular use of pad or self-catheterization | Permanent catheter |
Decreased stream | <Once-a-day self-catheterization | Dilatation, >once-a-day self-catheterization | Permanent catheter, surgical intervention |
Table 3
Analytic (A) component of the SOMA system for bladder
Analytic | |
Cystography | Assessment of mucosal surface |
Volumetric analysis | Assessment of bladder capacity in milliliters |
Contrast radiography | Assessment for ulcers, capacity and contractility |
Ultrasound | Assessment of wall thickness, sinus and fistula formation |
Electromyography | Assessment of sphincter activity using intralumenal pressure transducer, contraction pressure, and volume curves |
4.1 Detection
4.1.1 Initial Evaluation
The initial evaluation should include a careful history of the patient’s urinary complaints and a directed physical exam. Important questions include asking about the timing of onset of symptoms in relation to radiation therapy completion, distinguishing irritative (urinary frequency, urgency, dysuria) from obstructive symptoms (hesitancy, weak stream, dribbling), and identifying the presence of incontinence. If the patient is incontinent, the kind of incontinence should be distinguished, including urge incontinence (occurring after suddenly feeling the need or urge to urinate), stress incontinence (occurring when coughing, laughing, sneezing, or other movements that put pressure on the bladder), and overflow incontinence (occurring when there is unexpected leakage of small amounts of urine because of a full bladder). A broad differential for late bladder symptoms including tumor recurrence, urinary infection, bladder stones, and secondary malignancy should be considered in patients who have received radiation therapy. In particular, secondary malignancy should be considered in patients with a late onset of irritative symptoms or hematuria after radiation therapy or cyclophosphamide.
The initial physical exam should include careful palpation and percussion of the abdomen and subrapubic region to assess for bladder tenderness and distention. The external genitalia should be inspected for inflammation and/or other lesions, and a rectal exam should be performed to assess for pelvic masses. In females, a pelvic exam is usually indicated, particularly if there are symptoms concerning for urinary tract fistula such as continuous incontinence. A clean catch urine specimen is often used to assess for infection and urine analysis. A urinary tract infection can mimic and/or exacerbate radiation injury, and thus the urine should be examined for red blood cells, white blood cells, and bacteria, a urine culture should be obtained, and any infection treated promptly. In patients with symptoms that may be indicative for a secondary cancer (e.g. irritative symptoms, gross hematuria and/or persistent microscopic hematuria on urine analysis without evidence of infection after radiation or cyclophosphamide), a urine cytology to assess for malignancy and referral to an urologist may be indicated (Tables 2 and 4).
Table 4
Representative acute and long-term toxicities to the bladder in patients treated with external beam radiation attributable to either focal or global therapy
Focal | Global |
---|---|
Hematuria | Dysuria |
Fistula | Frequency |
Obstruction | Urgency |
Ulceration | Contracture |
Necrosis | Spasm |
Reduced flow | |
Incontinence |
4.2 Diagnosis
4.2.1 Imaging Evaluation
Initial radiographic work-up can include bladder ultrasound to assess for urinary retention, bladder wall thickness, masses, and bladder stones. Ultrasound of the upper tracts may be indicated if there are symptoms of flank pain, hematuria or renal dysfunction, and cystography may be indicated in patients with severe bladder dysfunction including incontinence (Fig. 6a, b).
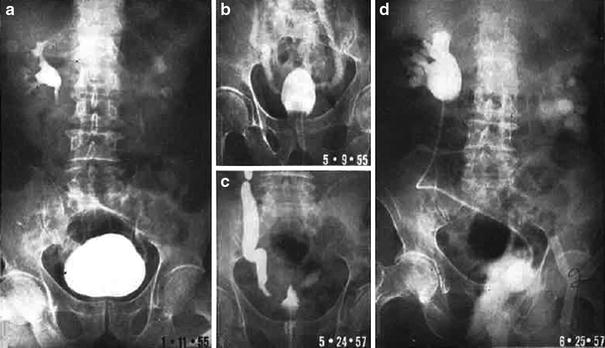
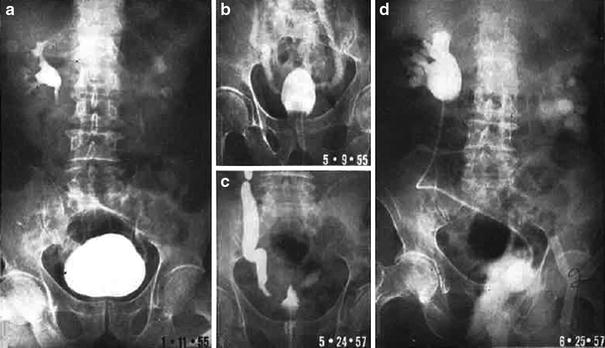
Fig. 6
a The patient presented with a Stage C, Grade IV carcinoma with adequate bladder capacity. b Two months after the completion of a 6 week course of 6,000 R irradiation, a decrease in bladder capacity and bilateral hydronephrosis were noted. c Severely contracted bladder with bilateral hydronephrosis. d Diversion required and performed via cutaneous ureterostomies (with permission from Rubin and Casarett 1968)
4.2.2 Urodynamics
Referral to an urologist for urodynamic evaluation may be indicated in patients with severe symptoms of late effects of radiation including incontinence, inability to empty the bladder, and severe irritative voiding symptoms. Urodynamic evaluation includes simultaneous measurement during voiding of intraluminal pressure, sphincteric activity and flow rate, and cystometry provides a measure of bladder capacity and compliance (Fig. 4b). A voiding cystourethrogram, which allows visualization of the urethra in males and assessment for vesicoureteral reflux, may be helpful in patients with dysuria and persistent infections. Fluoroscopy can provided added information about bladder wall movement during voiding.
4.2.3 Acute Symptoms
Acute side effects during radiation therapy and up to 3 months after treatment typically manifest as symptoms of hematuria, dysuria, increase in urinary frequency and urgency, bladder spasm, and incontinence. These symptoms typically can be medically managed and resolve several weeks to months after therapy. Severe Grade 4 complications such as fistula or obstruction are rarely seen, and typically related to tumor location and changes rather than a direct radiation effect.
The reported incidence of acute bladder symptoms in patients receiving radiation for various primary tumors of the pelvis varies widely from 23 to 80 % (Amdur et al. 1990; Pilepich et al. 1987; Quilty et al. 1985; Shipley et al. 1985). In males, acute symptoms may be due to inflammation of the prostate and prostatic urethra, which can be difficult to distinguish from bladder toxicity. The wide range in incidence rates reflects the heterogeneity in dose and treatment technique for different tumors, but also likely underscores the inherent difficulty in collecting such subjective data. Since acute toxicity from radiation is typically not severe, subtle urinary symptoms may be under-reported by physicians and patients.
4.2.4 Late Toxicity Syndromes
Long-term toxicities, those occurring ≥6 months after radiation, include similar symptoms to those listed under short-term side effects, but can progress to permanent bladder contracture causing frequency, incontinence, fistula, and ureteral or urethral obstruction, and hematuria from chronic cystitis and telangiectasia. Necrosis and perforation of the bladder wall are extremely rare from radiation alone, unless directly related to tumor. Incontinence is an important potential side effect, but is clearly confounded by the natural aging process, as well as any surgical procedures that may have previously been performed. For example, women that have had many children, or those that have had a hysterectomy, may be more prone to incontinence, as are men that have had prior bladder or prostatic surgery (Fig. 7a).
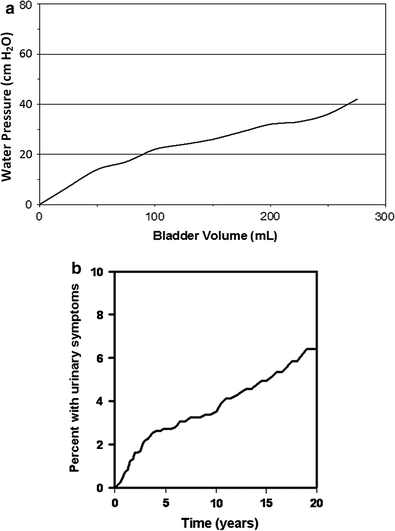
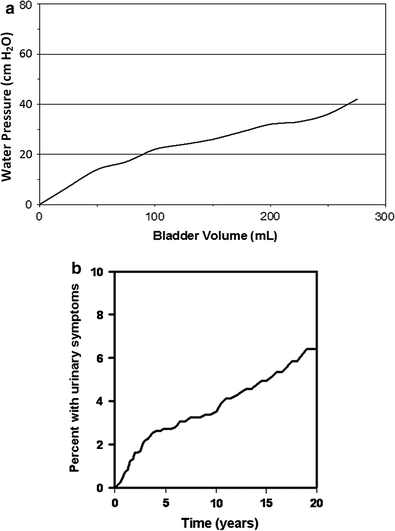
Fig. 7
a A cystometrogram performed 2 years after irradiation therapy for the management of cervical cancer. The bladder is reduced in capacity and shows a persistent rise in intravesical pressure with filling, which is consistent with loss of compliance due to fibrosis. (Adapted from Marks et al. 1995). b The cumulative frequency of developing “late” bladder complications, as a function of time in cervical cancer patients, persistently increases over decades. (Adapted from Eifel et al. 1995)
Late low-grade complications may impact the patient’s quality of life, and therefore should be consistently recorded and reported. Detecting long-term bladder complications requires at least 10 years of follow-up time and thus, bladder complications may be under-reported in the literature (Eifel et al. 1995). In general, the time to onset of late complications is several months to several years, but typically occurs 2–3 years after treatment with a median time of approximately 13–20 months, though the cumulative risk still rises at 4 years (Duncan and Quilty 1986; Duncan et al. 1986; Green et al. 1990; Greskovich et al. 1991; Lawton et al. 1991; Pilepich et al. 1981, 1987; Perez et al. 1984). The latency of several months before the onset of clinical symptoms in patients parallels the time of onset in mouse models, where a dose-dependent decrement of bladder capacity and increment in urinary frequency occurs only after 6 months (Stewart 1985; Stewart et al. 1978).
The long latency of late bladder symptoms was illustrated in a study of 1,784 patients with stage IB cervical cancer treated from 1960 to 1989 with approximately 45 Gy of external beam radiation therapy (EBRT) followed by tandem and ovoid brachytherapy (Eifel et al. 1995). Of these patients, 6.5 % developed hemorrhagic cystitis, a median of 3 years from the beginning of radiotherapy. The actuarial risk of hematuria was 6 % at 5 years, 7 % at 10 years, and 10 % at 20 years, with the risk of ≥ Grade 3 hematuria 1 , 1, and 2 %, respectively (Fig. 7b). Regression analysis controlling for confounders such as other medical conditions or type of radiation was not performed. Other series in patients treated with radiation therapy for cervical cancer have observed the first manifestation of bladder damage at an interval of 28 (Kottmeier 1964) and 39 (Zoubek et al. 1989) years after radiation treatment. While these are small case reports, long-term follow-up over decades may be necessary to accurately assess the risk of late bladder toxicity.
4.2.5 Grading Toxicity
Toxicity reporting has not been standardized. The methods used for toxicity scoring are not stated in many manuscripts. Timing criteria to distinguish between acute, subacute, and late complications are often not given and have not been standardized. In addition, complications are often only reported as crude numbers, rather than actuarial values, so one must be careful to exclude those patients no longer at risk for late bladder complications (e.g. those patients who underwent cystectomy for reasons other than complications).
Late damage may be assessed either symptomatically, radiographically, cystoscopically, cystometrically, or histologically. Symptoms can be quite varied (e.g. pain, bleeding, frequency, etc.) and the grading of toxicities based on treatment is subjective. Furthermore, it is difficult to classify some toxicities purely as bladder versus urethral in origin. Urethral symptoms such as hesitancy may be related to the amount of tumor present (for prostate cancer patients), or to the specific type of treatment (external beam only vs. brachytherapy only).
The vast majority of the available toxicity data is from retrospective chart reviews, which may underestimate the prevalence of complications. Patient-reported studies may provide a more accurate estimate of the prevalence of toxicity. For instance, Parkin et al., using a mailed questionnaire in patients treated with radiation for cervical cancer, noted 50 % of women reported urinary symptoms (Parkin et al. 1987). While a chart review likely underestimates the prevalence of symptoms, a mailed questionnaire may overestimate the problem since symptomatic patients may be more likely to respond to a questionnaire than asymptomatic patients. Additionally, scoring systems based on interventions directed by a physician may result in differences between institutions. A particular physician who is more likely to prescribe an intervention will note a higher rate of toxicity than would another physician who is less often prescribing an intervention. Toxicity scoring scales that require invasive procedure (e.g. cystoscopy) have fallen out of favor due to potential patient discomfort, inconvenience, and toxicity.
Several validated scoring systems exist for bladder toxicity including the RTOG-LENT/SOMA (Tables 2 and 3) criteria (Cox et al. 1995). In 2007, the most recent RTOG gynecologic group trials have incorporated the common toxicity criteria/adverse events (CTCAE) reporting version 3.0 which lists the following under renal/genitourinary toxicity: bladder spasms, cystitis, fistula, urinary incontinence, leak (including anastomotic, but without development of a fistula), obstruction, perforation, prolapse of stoma, renal failure, stricture/stenosis, urinary frequency/urgency, urinary retention, urine color change, and ‘other’, not distinguishing between acute or long-term effects. Further work is necessary to standardize the scoring of acute and late bladder toxicity.
In general, patient-reported toxicity data may be superior to physician reported data, although collection is more complex (Talcott et al. 1997; Litwin et al. 1995). Several patient-assessment scales exist, including the expanded prostate cancer index composite (EPIC) and Clark and Talcott scales, which continue to be developed and integrated into current clinical trials (Clark and Talcott 2001).
4.2.6 Differential Diagnosis of Radiation-Induced Bladder Toxicity
Urinary tract infections may frequently cause symptoms such as dysuria, urgency, and frequency that may mimic radiation injury. Further, radiation may cause mucosal changes, predisposing the patient to urinary tract infections. Therefore, it is imperative to be aware of the increased risk of infections and to appropriately diagnose a urinary tract infection with urinalysis and urinary cultures in patients presenting with dysuria and frequency.
In patients with symptoms suggestive of late bladder contraction and decreased compliance including increased frequency, incontinence, and pain, the differential diagnosis includes secondary malignancy, bladder neuromuscular dysfunction due to comorbidities such as diabetes mellitus, and underlying bladder pathology such as detrusor hypertrophy from outlet obstruction in men with benign prostatic hypertrophy (BPH). In patients with a history of transitional cell carcinoma or a long latent period after radiation (e.g. 10–20 years), the physician should have a particularly low index of suspicion for a secondary malignancy (See Sect. 4.2.7) and consider referral to a urologist (e.g. for cystoscopy).
4.2.7 Risk of Secondary Malignancy
Most of the data for secondary bladder malignancy after pelvic radiation therapy comes from retrospective series. Duncan et al. observed 8 secondary bladder malignancies in 2,674 patients treated with radiation for cervical cancer, which despite the low total number of cases, represents an incidence rate of 57.6 times that of the general female population (Duncan et al. 1977). In a case-controls series of 251 women with ovarian cancer, Kaldor et al. found a nearly 2-fold risk increase in secondary bladder malignancy for patients treated with radiation versus those treated with surgery alone (Kaldor et al. 1995). The addition of chemotherapy to radiation increased this risk. The use of cyclophosphamide increased the risk of secondary bladder malignancy 4-fold, regardless of the use of radiation or not.
The development of secondary malignancy of the bladder may have a very long latency period with bladder tumors observed as long as 10 years after radiation for ovarian cancer (Kaldor et al. 1995) and 20 years after radiation for cervical cancer (Duncan et al. 1977), which underscores the need for long-term follow-up and a high index of suspicion for secondary malignancy in patients presenting with hematuria after radiation therapy. Secondary bladder malignancies after radiation therapy are typically high grade, patients often present with locally advanced disease, and prognosis may be poor (Quilty and Kerr 1987).
An increased risk of secondary bladder malignancy after radiation exposure from the Chernobyl nuclear reactor meltdown has also been documented (Romanenko et al. 2002). In patients from the Ukraine undergoing cystoscopy for chronic cystitis or urinary retention, elevated urinary cesium-137 levels were detected in 156 patients residing in contaminated areas. In these patients, biopsies showed urothelial dysplasia in 139 (89 %), carcinoma in situ in 91 (58 %), and small transitional cell carcinoma in 10 (6.4 %). In a control group of 48 patients from noncontaminated areas of Ukraine, nine patients had dysplasia (19 %), but none had in situ or invasive carcinomas.
One analysis of secondary malignancy risk after brachytherapy and/or external beam radiation therapy for prostate cancer using Surveillance, Epidemiology, and End Results (SEER) data showed 11 cases of secondary bladder cancer in 448 patients at 5 years of follow-up. The incidence of secondary malignancy was 1.6 % in patients treated with brachytherapy alone versus 5.8 % in those receiving both brachytherapy and external beam radiotherapy (p = 0.0623). The relative risk of radiation therapy was 2.34 with a 95 % confidence interval of 0.96–3.72, and absolute excess risk of 35 cancers per 10,000 patients (Liauw et al. 2006). Similarly, in another SEER analysis, men with prostate cancer treated with external beam radiation therapy had an increased risk of secondary bladder cancer with an odds ratio of 1.63 with a 95 % confidence interval of 1.44–1.84 (Moon et al. 2006).
5 Radiation Tolerance: Predicting RT-Induced Injury
The different primary cancers originating in the pelvis have distinct treatment modalities, and thus radiation effects on the bladder are influenced by widely heterogeneous radiation techniques, dosing, proportion of the bladder irradiated, contribution from external beam versus brachytherapy, influence of chemotherapy and surgery in cases of combined modality, and underlying bladder pathology. Additionally, many patients may have underlying bladder pathology and dysfunction such as bladder muscle hypertrophy due to outlet obstruction in the case of men with benign prostatic hypertrophy, neurogenic bladders in patients with neuromuscular disorders, and chronic cystitis, which can confound the effects of radiation therapy and/or increase the risk of complications. Thus the correlation of radiation dose to late complications remains unclear, and the guidelines for the dose tolerance of the bladder have been revised upward since the 1990s.
5.1 Radiation Dose/Time Fractionation
Depending on the location of the primary tumor, anatomic variation of the bladder, and the type of radiation administered, the dose received by the bladder varies substantially. The incidence and type of bladder toxicity may be altered by radiation technique including: external beam radiation therapy versus brachytherapy, and low-dose whole bladder irradiation versus high dose partial bladder irradiation. To date, the ability to correlate 3-dimensional bladder dosimetry with the risk of complication has not been possible, since the bladder volume and location is highly variable. Additionally, the radiation-induced bladder toxicity profile for a specific patient will depend not only on the specifics of the treatment regimen, but also on the origin, stage, and the location of the primary tumor. For example, patients with bladder cancer may appear to have more acute radiation-induced symptoms than prostate cancer patients, but these symptoms may be related to the tumor response, the condition of the underlying and surrounding mucosa, the amount of bladder irradiated, and the daily motion of the bladder in and out of the field (Fig. 8).
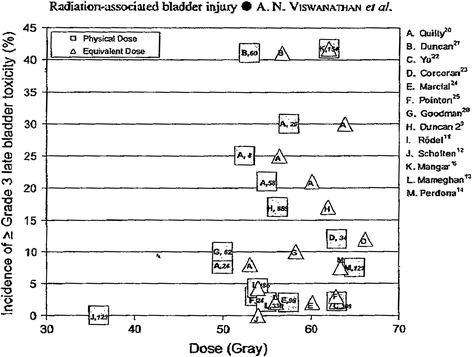
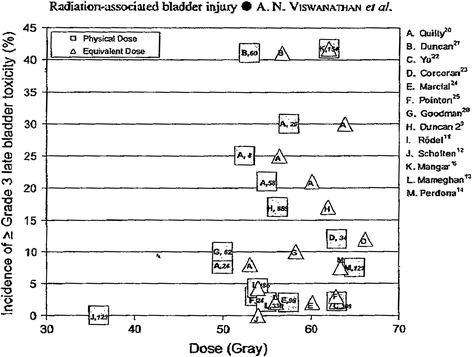
Fig. 8
Incidence of RTOG Grade 3 or greater later bladder toxicity in relation to average dose (squares) or linear-quadratic model equivalent dose (triangles) in 2 Gy fractions [From QUANTEC review, Viswanathan 2010]
Bladder volume and location uncertainties make measuring true dose–volume relationships and estimating bladder dose tolerances difficult. In this section, the incidence of bladder complications after radiation treatment for bladder, prostate, and gynecological cancers will be reviewed, and the influence of radiation technique, bladder volume uncertainties, and contribution of brachytherapy dose will be discussed. The effects of whole bladder versus partial bladder irradiation on the risk of late complications will also be reviewed by cancer type.
5.1.1 Hypofractionation
Hypofractionation appears to increase the risk of late bladder toxicity (Marks et al. 1995). In prostate cancer patients treated with 5.17 Gy per fraction twice a week through AP-PA fields over 9 weeks with a 3-week break mid-way through therapy, there was a 19 % rate of serious bladder injury, including both global (cystitis and contracture) and focal (fistula) injury (Lindholt and Hansen 1986). Total dose with hypofractionation may also influence the risk of toxicity; another series found acceptable late bladder toxicity for patients receiving whole bladder treatment for bladder cancer in 20 fractions to 50 or 52.5 Gy, but a high toxicity rate with a total dose of 57.5 Gy (Quilty et al. 1985).
As with other normal tissues, the duration between treatments may be important. In one study of bladder cancer radiation therapy with 2D techniques, there was a 32 % risk of severe bladder injury with a split-course technique of 40 Gy to a large volume of the bladder (whole pelvic fields), followed by a boost dose to a small portion of the bladder to a dose of 20 Gy with both courses delivered at 2 Gy per fraction with 3 fractions per day and an inter-fraction interval of 4 h (Vanuytsel et al. 1986). However, in a randomized trial of 229 patients with muscle-invading bladder tumors treated without chemotherapy, there was no differences in acute and late bladder complications in patients treated with daily radiation therapy to 64 Gy in 32 fractions versus twice daily radiation therapy to 60.8 Gy in 32 fractions with a minimum 6 h gap between treatments (Horwich et al. 2005).
5.2 Dose–Volume Estimates (Focal vs. Global Bladder Radiation)
Side effects from bladder radiation may arise either from whole organ radiation (global injury), such as in treatment to the male or female pelvis, though this is usually to a more limited dose (40–50 Gy), or from higher doses to a small field resulting in focal injury, such as in cone down volumes for bladder cancer (approximately 65 Gy) or prostate cancer (>70 Gy). Brachytherapy results in significantly higher doses to the bladder than that administered by external beam alone (>75 Gy), but the volumes of bladder exposed to these high doses are much smaller (Fig. 9). Therefore, when discussing bladder toxicities in relationship to dose, it is important to acknowledge the heterogeneity of dose depending on whether the patient has received brachytherapy, external beam radiation, or both and the range of toxicities that may develop (Table 4). Unfortunately, no studies to date have comprehensively monitored true 3-dimensional bladder dosimetry in relation to toxicities. The 1991 publication by Emami et al. reported a bladder TD5/5 for whole and 2/3 volume irradiation of 65 and 80 Gy, respectively, based on initial series that did not utilize 3-dimensional imaging (Emami et al. 1991). The TD50/5 data point of 80 Gy was considered speculative. The endpoint for these estimates was “symptomatic bladder contracture and volume loss” (n = 0.5, m = 0.11).
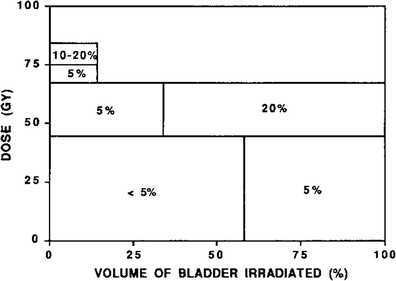
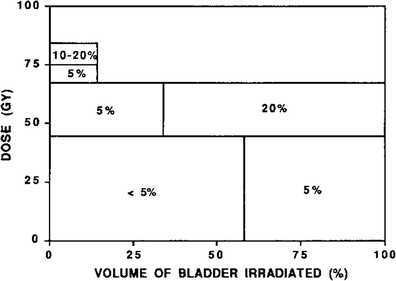
Fig. 9
Schematic diagram illustrating the relationship between dose–volume parameters and the complication rate shown. Adapted from Marks et al. 1995 with permission
Marks et al. in 1995 generated estimates of bladder toxicity based on complication rates seen on review of the existing literature of radiation treatment for prostate cancer, bladder cancer, and cervical cancer. Analyzing the incidence of Grade 3–4 toxicity and dose–volume relationships after external beam radiation therapy and/or brachytherapy, Marks et al. hypothesized that there were different dose tolerances for global and focal radiation-induced injury (Marks et al. 1995). Based on the published literature up to that point, Marks et al. estimated a clinical complication rate of ~5–10 % with a whole bladder dose of 50 Gy, and higher complication rates with doses of 50–65 Gy. Irradiation of approximately one-third to half of the bladder volume to a dose of 50–65 Gy resulted in ~5–10 % complication rates. Irradiation of smaller portions of the bladder (<20 %) to higher doses of 65–75 Gy resulted in late toxicity in ~5–10 % of patients. Additionally, the urethral tolerance dose was estimated to be greater than ~60–70 Gy; these doses resulted in urethral strictures in ~0–5 % of patients. Based on these data, Marks et al. estimated that whole bladder irradiation >50 Gy in 2 Gy fractions resulted in a significant risk of severe global bladder dysfunction, while partial bladder volume irradiation to doses greater than 75–80 Gy may be associated with a >10 % risk of serious bladder injury. However, these data are based on older studies and may overestimate the risk of bladder injury with current radiation techniques. Finally, Marks et al. estimated based on mice model data and the existing clinical data at that time that fraction sizes greater than 2.0 Gy increased the risk of bladder toxicity (Duncan et al. 1986; Quilty and Duncan 1986).
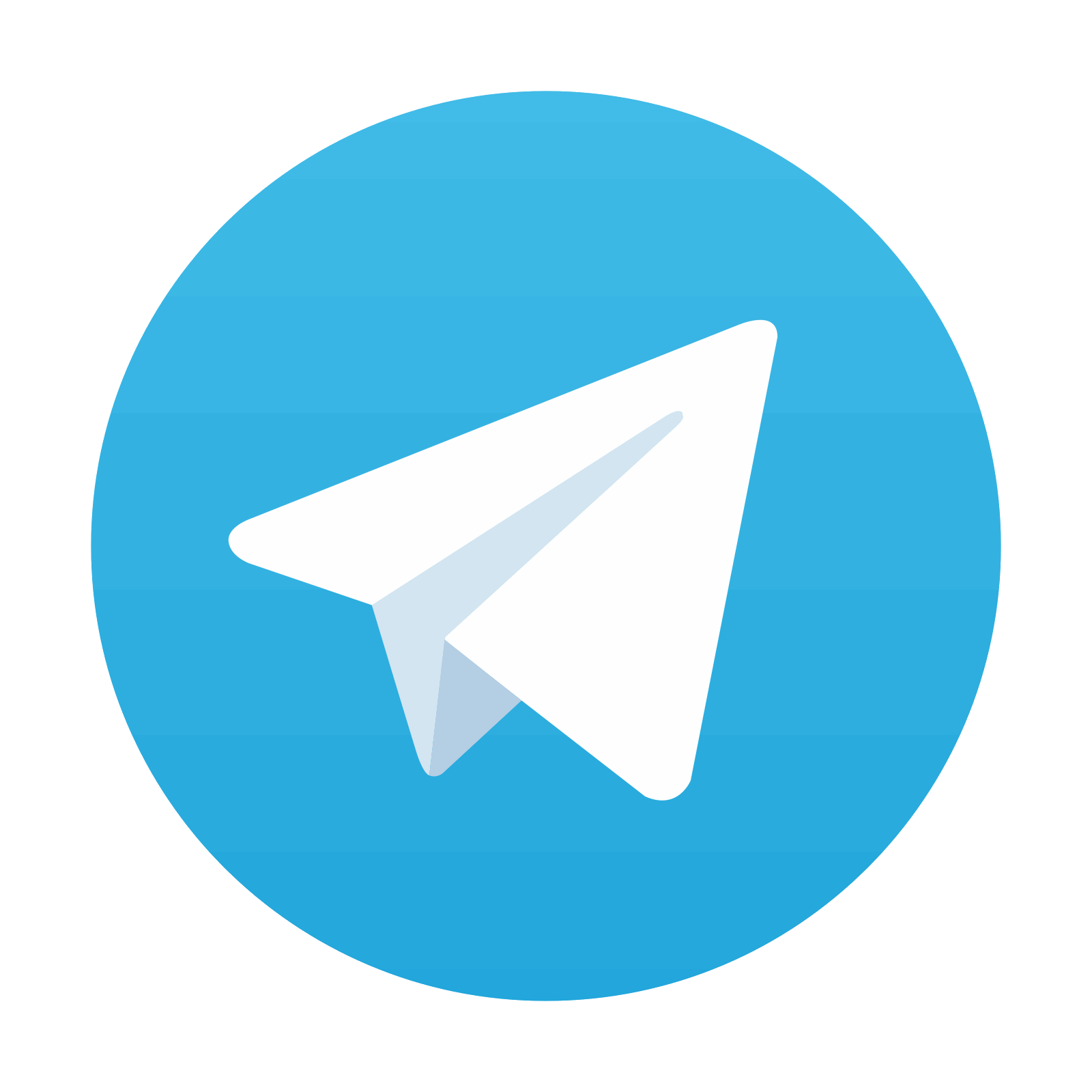
Stay updated, free articles. Join our Telegram channel

Full access? Get Clinical Tree
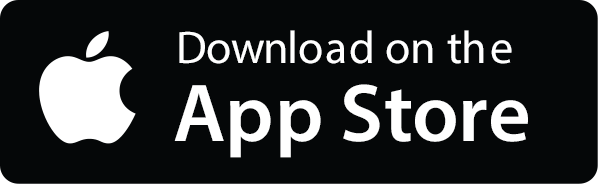
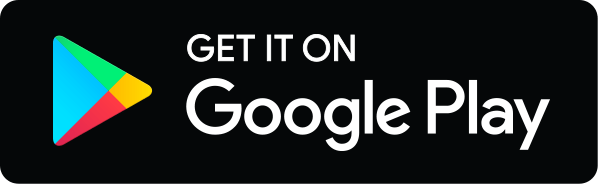