Functional magnetic resonance imaging (fMRI) has become a common tool for presurgical sensorimotor mapping, and is a significant preoperative asset for tumors located adjacent to the central sulcus. fMRI has changed surgical options for many patients. This noninvasive tool allows for easy display and integration with other neuroimaging techniques. Although fMRI is a useful preoperative tool, it is not perfect. Tumors that affect the normal vascular coupling of neuronal activity will affect fMRI measurements. This article discusses the usefulness of blood oxygen level dependent (BOLD) fMRI with regard to preoperative motor mapping.
Key points
- •
Functional magnetic resonance imaging (fMRI) has become a common tool for presurgical sensorimotor mapping.
- •
fMRI is a significant preoperative asset for tumors located adjacent to the central sulcus.
- •
fMRI has changed surgical options for many patients. This noninvasive tool allows for easy display and integration with other neuroimaging techniques.
- •
Although fMRI is a useful preoperative tool, it is not perfect. Tumors that affect the normal vascular coupling of neuronal activity will affect fMRI measurement.
Introduction
In the early 1990s, functional magnetic resonance imaging (fMRI) entered neuroimaging as a unique resource in the arsenal of preoperative planning tools for patients with brain tumors. fMRI is a technique that takes advantage of the differences in magnetic susceptibility between oxyhemoglobin and deoxyhemoglobin. It is a less invasive neuroimaging method than its positron emission tomography (PET) predecessor, given that the contrast agent is endogenous. fMRI is possible because oxyhemoglobin has a magnetic resonance (MR) signal different from that of than deoxyhemoglobin. When a task is performed, oxygenated blood in excess of the amount needed (termed luxury perfusion) is delivered to the active area. The difference in magnetic susceptibility between deoxyhemoglobin concentrations and oxyhemoglobin concentrations creates the signal in functional imaging. This effect is termed the blood oxygen level dependent (BOLD) signal. fMRI provides good spatial localization (as low as 1 mm) and temporal acquisition resolution (as low as 1 second) but is limited by the resolution of the hemodynamic response (8–30 seconds). The superior spatial resolution is particularly advantageous for mapping peritumoral eloquent areas for treatment planning.
fMRI can effectively map the sensory and motor areas. The motor gyrus is somatotopically organized, with all body parts represented in a way that is preserved across different people. fMRI can provide a multidimensional map in a single mapping session. Such maps of sensorimotor function help the surgeon to assess the risks of surgery and guide intraoperative mapping techniques. The primary motor and sensory areas in fMRI are of particular interest for surgical planning, because iatrogenic damage to these areas can cause permanent neurologic deficits. As a result the precise localization of various motor and sensory areas is useful, particularly in the setting of a space-occupying lesion.
Primary motor and sensory cortices are distinct in the functions they subserve. However, as a result of significant neuronal reciprocity in the region, injury to either can result in a mixed motor/sensory deficit. For example, injury to the primary motor gyrus usually leads to a permanent, largely irreversible paresis. Injury to the sensory cortex, while producing the expected sensory perceptual deficits, can also lead to a similar type of paresis seen with injury to the motor strip as a result of the lack of proprioceptive information. A variety of other deficits is seen with injury to the postcentral gyrus, depending on whether the left or right hemisphere is damaged. Some of these include 2-point discrimination, astereognosis (inability to discern objects by feeling them), and agraphism (inability to write). This article discusses the usefulness of BOLD fMRI with regard to preoperative motor mapping.
Introduction
In the early 1990s, functional magnetic resonance imaging (fMRI) entered neuroimaging as a unique resource in the arsenal of preoperative planning tools for patients with brain tumors. fMRI is a technique that takes advantage of the differences in magnetic susceptibility between oxyhemoglobin and deoxyhemoglobin. It is a less invasive neuroimaging method than its positron emission tomography (PET) predecessor, given that the contrast agent is endogenous. fMRI is possible because oxyhemoglobin has a magnetic resonance (MR) signal different from that of than deoxyhemoglobin. When a task is performed, oxygenated blood in excess of the amount needed (termed luxury perfusion) is delivered to the active area. The difference in magnetic susceptibility between deoxyhemoglobin concentrations and oxyhemoglobin concentrations creates the signal in functional imaging. This effect is termed the blood oxygen level dependent (BOLD) signal. fMRI provides good spatial localization (as low as 1 mm) and temporal acquisition resolution (as low as 1 second) but is limited by the resolution of the hemodynamic response (8–30 seconds). The superior spatial resolution is particularly advantageous for mapping peritumoral eloquent areas for treatment planning.
fMRI can effectively map the sensory and motor areas. The motor gyrus is somatotopically organized, with all body parts represented in a way that is preserved across different people. fMRI can provide a multidimensional map in a single mapping session. Such maps of sensorimotor function help the surgeon to assess the risks of surgery and guide intraoperative mapping techniques. The primary motor and sensory areas in fMRI are of particular interest for surgical planning, because iatrogenic damage to these areas can cause permanent neurologic deficits. As a result the precise localization of various motor and sensory areas is useful, particularly in the setting of a space-occupying lesion.
Primary motor and sensory cortices are distinct in the functions they subserve. However, as a result of significant neuronal reciprocity in the region, injury to either can result in a mixed motor/sensory deficit. For example, injury to the primary motor gyrus usually leads to a permanent, largely irreversible paresis. Injury to the sensory cortex, while producing the expected sensory perceptual deficits, can also lead to a similar type of paresis seen with injury to the motor strip as a result of the lack of proprioceptive information. A variety of other deficits is seen with injury to the postcentral gyrus, depending on whether the left or right hemisphere is damaged. Some of these include 2-point discrimination, astereognosis (inability to discern objects by feeling them), and agraphism (inability to write). This article discusses the usefulness of BOLD fMRI with regard to preoperative motor mapping.
Anatomic organization of the sensorimotor system
The 4 main regions that subserve motor control that are of interest to neurosurgeons are the primary motor cortex, the primary sensory cortex, the premotor cortex, and the supplementary motor area (SMA). The motor and sensory gyri taken together are often referred to as one larger area termed the primary sensorimotor cortex.
The Primary Sensorimotor Cortex
The primary motor cortex, located in the precentral gyrus, is responsible for executing movement ( Fig. 1 ). Its position delineates the frontal from the parietal lobes. The motor gyrus marks the posterior limit of the frontal lobe and the sensory gyrus marks the start of the parietal lobe. The motor gyrus is somatotopically mapped; different body regions are distinctly represented in cortical space in a common (but not steadfast) pattern medially to laterally. Historically, the motor gyrus has been localized using anatomic markers. The most salient anatomic marker of the motor gyrus is the reverse omega portion of the central sulcus (see Fig. 1 ). This reverse omega typically demarcates the location of the hand motor region of the motor homunculus. However, the presence of this marker is occasionally unreliable. Fig. 2 shows a case whereby a reverse omega sign would have incorrectly indicated the position of the motor gyrus. Although cases like this are rare, they do occur. Furthermore, lesions can obscure traditional anatomic markers, making difficult their identification based on visual inspection of MR images alone. Fig. 3 shows a case in which anatomic markers have been obscured by tumor, making anatomic prediction of the location of the motor gyrus impossible without a technique such as fMRI.
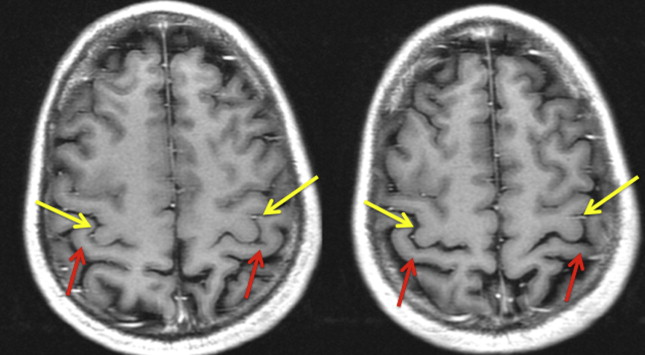
The face/tongue region of the primary motor gyrus is located on the lateral/inferior aspect of the motor gyrus. This region is anatomically just posterior to the area of Broca in the inferior frontal gyrus. Fig. 4 shows an fMRI map of both hand and tongue motor movements acquired simultaneously from an intact patient. Of note, finding the tongue motor region by “pulling down” the sulcus, whereby one first locates the more cephalad component of the central sulcus/reverse omega and follows the sulcus inferiorly, can be misleading and inaccurate. The inferior aspect of the central sulcus moves anteriorly as it is traced inferiorly and shortens, making precise localization of the inferior aspect of the motor gyrus particularly difficult to be discerned anatomically alone. For this reason, fMRI is particularly useful for localizing the face/lips/tongue portion of the motor gyrus at its inferior aspect.
Another way in which fMRI contributes significantly to motor gyrus localization is in the foot motor region. The foot motor region is located most medially just over the interhemispheric fissure. This region is often localized medial and slightly posterior to the hand motor region in the axial plane ( Fig. 5 ). Direct cortical stimulation (the surgeon’s intraoperative gold standard for functional mapping) of this region is difficult because the sagittal sinus makes the cortex difficult to access. Therefore, fMRI localization of the foot motor region is valuable for presurgical planning.
fMRI typically maps these 3 main motor areas (foot, hand, and face/tongue) for neurosurgical planning, partly because these 3 areas span the gyrus medially to laterally and partly because tasks involving these areas are easily amenable to functional paradigms.
The primary sensory gyrus (also known as the postcentral gyrus) is located just posterior to the precentral gyrus, from which it is divided by the central sulcus. Like the primary motor gyrus, the organization of the sensory gyrus is also somatotopically organized (see Fig. 1 ).
Secondary Motor Areas
Although the primary motor and sensory areas are the main focus of most neurosurgical planning targets, damage to secondary motor areas also carries a risk of morbidity. As a result, their precise localization is becoming increasingly important during fMRI examinations. The most common secondary motor areas of interest for neurosurgical planning are the SMA and the premotor area. To study the secondary areas, paradigms often focus on a unilateral volitional movement contrasted with rest.
Supplementary Motor Area
The SMA is located in the superior frontal gyrus just medial to the superior frontal sulcus ( Fig. 6 ). Although it is an expansive area with ill-defined anterior borders, the posterior border of the SMA is the foot motor region of the primary motor gyrus. The SMA is made up of an anterior portion (pre-SMA), more active on fMRI during language tasks, and a posterior portion, more active on fMRI during motor tasks. The boundary between the pre-SMA and SMA proper has been delineated using a VCA (vertical commissure anterior) line or a line drawn vertically from the AC/PC (anterior/posterior commissure) line.
Recent studies suggest that the motor portion of the SMA is, like the primary motor gyrus, also somatotopically arranged. In lower animals, it has been shown that the hind limb is located in caudal sites, whereas the forelimb and facial movements are closer to the pre-SMA, the more anterior, language-related portion of the SMA, and are thus more rostral. Although somatotopic organization is more commonly associated with the primary motor cortex, the 3 main motor areas (hand, foot, and face/tongue) may also be somatotopically mapped along the more rostral axis of the SMA.
The SMA is broadly responsible for motor planning and activates temporally before the primary motor gyrus. Furthermore, it is active when movements are both internally and externally cued. The SMA is best known for being associated with voluntary movement but will also activate on fMRI during passive tasks. The posterior portion of the SMA is more involved in finger-movement tasks, whereas the anterior part of the SMA is active during cognitive and language processing. A centralized region of the SMA that is active during both language and motor tasks suggests a region that may be essential. Further investigation is needed to determine whether insult to this centralized region carries an increased incidence or degree of postoperative deficit.
More recent work suggests a role for the SMA in cortical compensation. BOLD fMRI can be used preoperatively to look at the patterns of activation as a tumor invades either the primary motor area or the supplementary motor area. The SMA has been shown to be involved in temporal planning and organization of motor movements before execution, in addition to sequencing multiple movements. Peck and colleagues characterized the role of the SMA in patients with high-grade gliomas and their role in cortical reorganization. The study used fMRI to look at the BOLD hemodynamic responses in the primary motor and SMA of tumor patients. Here, block paradigms were used to assess latency differences so that the more sensitive hemodynamic response would be isolated. This work concluded that patients with glial tumors located within the primary motor cortex experienced lesion-induced compensation in the BOLD magnitude and firing pattern in both the primary motor cortex and the SMA. Cortical reorganization was visibly demonstrated, with the SMA’s role assuming functions for which the primary motor cortex was likely previously responsible.
Why functional MR imaging?
fMRI is especially useful for locating the primary sensorimotor cortex if normal sulci and/or gyral patterns are distorted, or in rare cases when reorganization has occurred secondary to an invasive tumor. fMRI, as a preoperative neuroimaging tool to localize the sensory/motor system, is popular for a variety of reasons. This method is minimally invasive and easily repeatable. Moreover, given that patients with brain tumors are often impaired, fMRI of sensorimotor regions can be acquired with a variety of paradigms that are both volitional and passive. Patients undergo preoperative motor mapping for a multitude of reasons; mostly when anatomic landmarks cannot be identified with certainty by traditional anatomic means. fMRI is also being investigated as a tool to predict deficits. Lastly, fMRI can be used to interrogate cortical reorganization in the motor system, the clinical utility of which is still under investigation.
Several studies over the past decade provide evidence for the usefulness of fMRI for preoperative sensorimotor mapping. In a 2007 study by Pujol and colleagues, patients were examined to identify the sensory motor cortex over a 5-year period. Subjects performed a hand-motion paradigm (opening and closing). The fMRI map correctly identified the location of the sensorimotor cortex in 96% of the cases (141 of 147 patients examined). The 4% that could not be identified displayed head motion greater than 2 mm. Although both conventional MR and fMRI were used and compared in this study, fMRI significantly increased the confidence of the identification of motor gyrus.
Studies investigating the outcomes of fMRI for preoperative identification of eloquent areas are ongoing. An important step in this direction was published by Petrella and colleagues, who investigated presurgical fMRI in 39 patients who were candidates for tumor resection. Two paradigms were used to map sensorimotor areas. Treatment plans following inspection of the fMRI results were altered in 49% of patients. In this study, fMRI sufficiently changed treatment options, offering patients who would have otherwise been deemed inoperable the chance for surgical resection. In addition, in 9 patients for whom surgery was not originally offered, 5 were reconsidered for a craniotomy with intraoperative mapping. In cases where the course of treatment continued as planned, fMRI provided the neurosurgeons with further confidence in their surgical decision making. In a 2003 study by Wilkinson and colleagues, preoperative maps created through fMRI data were essential to safe resection by way of allowing for gross total resections and no postoperative deficits in 17 patients mapped with fMRI. Identifying the eloquent areas to prevent damage during tumor removal is of the utmost importance during mapping. Tumors initially believed to be at too high risk for safe resections were now possible to resect because anatomic locations were available through preoperative fMRI. In this study, no patients displayed permanent neurologic damage after surgery.
Paradigms
fMRI localization depends on the paradigm used to elicit the activation. In the motor system, these paradigms are relatively straightforward. Common paradigms for the motor area are finger tapping, tongue motion, and foot motion. Finger tapping most commonly involves having patients tap their fingers while in the scanner, while simultaneously avoiding movement of the arms or shoulders. During the tongue-motion paradigm, patients are asked to keep teeth closed to avoid head-motion artifacts and sweep their tongue against the back of their teeth. Motor foot localization consists of repetitive flexion and extension of the toes without moving the ankles. In most cases, small movements of the foot, hand, or tongue provide a significant signal, particularly when head motion is absent.
A variety of ways is used to perform motor paradigms with patients. Some methods are designed to localize the motor gyrus in both hemispheres simultaneously with bilateral finger tapping (to asses motor gyrus displacement), and in some cases patients can be asked to both move their tongue while tapping their fingers to localize both hand and tongue in a single experimental run. Yet another design involves no rest; that is, instead of alternating between a single motor task and rest, patients are instead asked to alternate between finger tapping and tongue motion. In all cases, careful attention should be paid to minimizing head motion. Short scanning time and clear instruction helps to minimize artifacts.
fMRI examinations of motor function, like any fMR examination, can be performed using a block design or an event-related design. In many fMRI examinations there are 2 states that are statistically contrasted in postprocessing analysis. In an event-related design the patient performs one event (eg, a finger tap), which is followed by rest. This type of paradigm allows for detailed estimation of the hemodynamic response. Event-related designs, though possible in patient populations, are arguably preferred in basic science fMRI, as more precise neuroanatomic parameters can be extracted from single events. However, this type of design requires many repetitions because the change from baseline for any one event is small (on the order of about 2%–6%). Accordingly, event-related designs tend to be longer than block designs given the need for many repetitions for adequate statistical power. This approach can be problematic for patients with brain tumors who, in the authors’ experience, can have trouble keeping still and following complicated instructions with rapid alternations in task demand.
Block designs, by contrast, average the signal from many of the same types of events over a single epoch. For example, a typical block-designed motor task would have the patient resting for 5 images and finger tapping for 5 images. This alternating cycle of rest and task would repeat 5 or 6 times and last approximately 5 minutes, depending on the time to repeat of the images. The advantage of the block design is that the task-related images and the rest-related images are signal averaged. Therefore the block design maximizes detection of the signal, whereas the event-related design maximizes estimation of the signal. That being said, there is a role for event-related motor paradigms. Marquart and colleagues indicated that for finger-movement tasks whereby head-motion artifacts are less likely to occur, a block trial is preferred because of greater activation seen within the sensorimotor cortex and/or the SMA. However, for toe- and tongue-movement tasks that are more susceptible to movement artifacts, event-related or single-event paradigms adequately localize the foot motor areas.
There are also many special considerations when using fMRI maps for clinical use. For example, in patients with brain tumor it is helpful to have a shorter task or paradigm duration, as patients have a more difficult time than normal controls in keeping their head still. Whereas fMRI data are often acquired using an event-related design in healthy control subjects, patients with brain tumors often benefit from the signal averaging afforded by block designs. Furthermore, areas can be activated that are associated with the task being investigated but are not essential for the task. Of course, this is an important consideration for BOLD fMRI mapping for neurosurgical planning, the goal of which is to isolate essential eloquent areas. That being said, fMRI is commonly used to map eloquent areas presurgically and has been shown to be sufficiently accurate, particularly in motor areas, for neurosurgical planning in a multitude of studies.
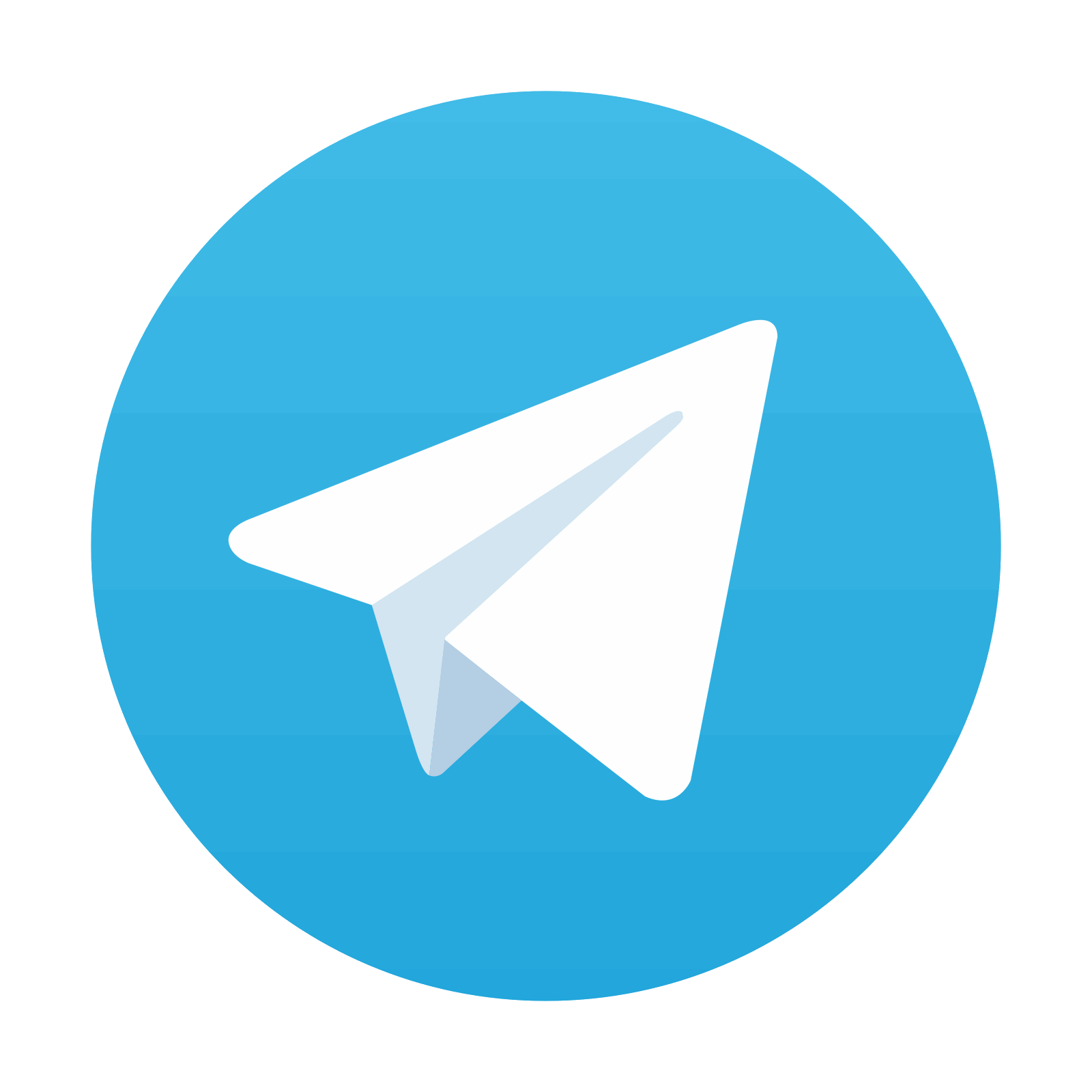
Stay updated, free articles. Join our Telegram channel

Full access? Get Clinical Tree
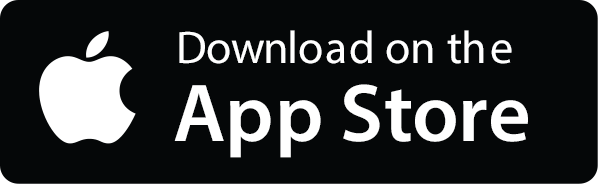
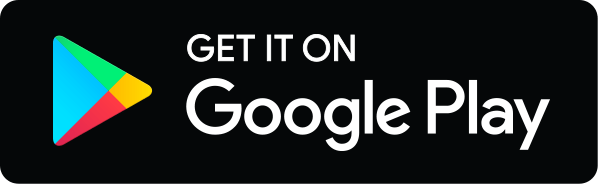